ABSTRACT
Hydroxytyrosol (HT) and oleuropein (OLE) are natural polyphenols found in extra virgin olive oil. Accumulating evidence indicates that ingestion of olive oil contributes to reduce the risk of cardiovascular diseases and stroke. It has been reported that HT and OLE inhibit human platelet aggregation. We have shown that collagen induces the phosphorylation of heat shock protein 27 (HSP27) in human platelets, resulting in the release of HSP27, an extracellular pro-inflammatory agent. In this study, we investigated the effects of HT and OLE on the collagen-stimulated human platelet activation. The PDGF-AB secretion and the soluble CD40 ligand (sCD40L) release by collagen were reduced by HT or OLE. HT and OLE significantly suppressed the phosphorylation of HSP27 and the release of phosphorylated-HSP27. These findings suggest that olive polyphenol reduces the collagen-stimulated phosphorylation of HSP27 in human platelets and the release. Our results may provide a novel anti- inflammatory effect of olive polyphenol.
Graphical abstract
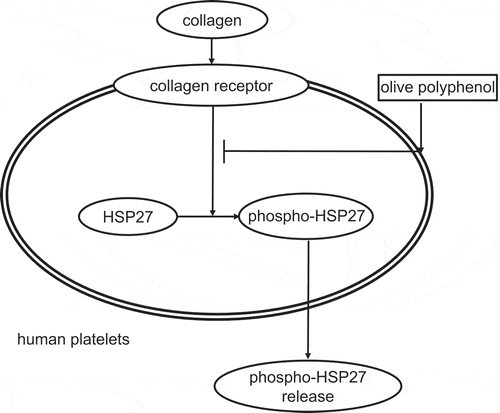
Olive polyphenol reduces the collagen-stimulated phosphorylation of HSP27 in human platelets and the release.
Olive oil, a vital component in Mediterranean diet, contains various types of phenolic compounds including phenolic alcohols, phenolic acid, flavonoids, lignans, and secoiridoids [Citation1,Citation2]. It is well known that olive oil consumption reduces the incidence of atherothrombotic diseases such as myocardial infarction and stroke in human being [Citation1,Citation2]. Oleuropein (OLE), the major content of olive oil phenolic compounds, is hydrolyzed to hydroxytyrosol (HT) during the storage of olive oil [Citation3]. In addition, in vivo, OLE is also hydrolyzed into HT in the gastrointestinal tract following oral intake [Citation3]. OLE and HT are classified into phenolic alcohol, and phenolic secoiridoid, respectively [Citation1,Citation2]. It has been shown that olive polyphenols including OLE and HT have cytoprotective effects such as anti-oxidant, anti-inflammatory and anti-tumor biological activities [Citation4–Citation8]. However, the exact mechanism of olive polyphenols against atherosclerotic diseases has not yet been precisely clarified.
Human platelets play a pivotal role in the development of thrombus formation associated with cardiovascular diseases in addition to hemostasis [Citation9,Citation10]. The platelets activation is triggered by initial tethering at the site of vascular injury exposing sub-endothelial collagen [Citation9,Citation10]. It is well known that collagen is a potent stimulator for human platelets via glycoprotein (GP) VI and integrin α2β1 on the plasma membrane [Citation11–Citation13]. Collagen is also well recognized to induce GPVI activation in human platelets [Citation14]. The activated GPVI induces integrin activity, leading to platelet aggregation and the activation of platelets, which is accompanied with the granule secretion of platelet-derived growth factor-AB (PDGF-AB) stored in α-granule [Citation11,Citation13,Citation15]. In addition, the activated human platelets release various inflammatory mediators including soluble CD40 ligand (sCD40L)[Citation9]. These agents act as inducers of atherosclerosis, which is closely related to the cardiovascular or cerebrovascular diseases [Citation16–Citation19]. Regarding the effects of olive oil-derived polyphenols on human platelets, it has been reported that HT and OLE inhibit the platelet aggregation and thromboxane B2 production induced by collagen [Citation20,Citation21]. However, it remains to be investigated how HT and OLE affect the functions of human platelets.
Various environmental stresses such as heat induce the expression of heat shock proteins (HSPs) in cells [Citation22,Citation23]. It is firmly established that HSPs intracellularly play important roles in proteostasis as molecular chaperones [Citation22,Citation23]. Currently, the HSP family are classified into seven groups: HSPH (HSP110), HSPC (HSP90), HSPA (HSP70), HSPD/E (HSP60/10), CCT (TRiC), DNAJ (HSP40) and HSPB (small HSP)[Citation24]. The HSP group with a molecular weight of 13–43 kDa is defined as HSPB [Citation23,Citation24]. HSP27 (HSPBs) ubiquitously exists in various unstressed cells including human platelets [Citation23]. It is generally recognized that HSP27 receives post-translational modification such as phosphorylation [Citation25]. Human HSP27 is reportedly phosphorylated at three serine residues (Ser-15, Ser-78, and Ser-82) [Citation22,Citation25,Citation26]. Accompanied with the phosphorylation, HSP27 is rapidly dissociated into a dimer or monomer from unphosphorylated aggregated form, resulting in the modulation of its chaperone activity [Citation22,Citation27–Citation29]. In addition to chaperone activity, it has been reported that HSP27 plays important roles such as vascular contraction and apoptosis [Citation25,Citation27,Citation30–Citation33]. On the other hand, accumulating evidence indicates the extracellular functions of HSP27 such as the modulation of inflammation [Citation34]. With regard to HSP27 in human platelets, we have previously demonstrated that collagen induces HSP27 phosphorylation, and that PDGF-AB secretion and sCD40L release are correlated with the phosphorylation of HSP27 [Citation35,Citation36]. In addition, we have recently shown that phosphorylated-HSP27 is released from the collagen-stimulated platelets derived from diabetic patients [Citation37].
In the present study, we investigated the effects of HT and OLE on the collagen-stimulated activation of human platelets. We herein show that both HT and OLE suppress the collagen-stimulated phosphorylation of HSP27 in human platelets, leading to the reduction of phosphorylated-HSP27 release.
Materials and methods
Materials
HT (3-hydroxytyrosol) and OLE were purchased from Sigma-Aldrich (St. Louis, MO, USA). Collagen was obtained from Takeda Austria Gmbh (Linz, Austria). Phospho-specific HSP27 (Ser-78) antibodies and phosphorylated-HSP27 (Ser-78) ELISA kit were purchased from Enzo Life Science, Inc. (Farmingdale, NY, USA). HSP27 antibodies and GAPDH antibodies were obtained from Santa Cruz Biotechnology, Inc. (Santa Cruz, CA, USA). PDGF-AB enzyme-linked immunosorbent assay (ELISA) kit and sCD40L ELISA kit were purchased from R&D Systems, Inc. (Minneapolis, MN, USA). Other materials and chemicals were obtained from commercial sources. HT and OLE were dissolved in dimethyl sulfoxide. The maximum concentration of dimethyl sulfoxide was 0.5%, which had no influence on the platelet aggregation, the protein detection by Western blot analysis or ELISA for PDGF-AB, sCD40L and phosphorylated-HSP27.
Preparation of platelets
Blood samples were donated from healthy volunteers, and a 1/10 volume of 3.8% sodium citrate was added immediately as an anti-coagulant. Platelet-rich plasma (PRP) was obtained by centrifuging at 155 × g for 12 min at room temperature. Platelet-poor plasma (PPP) was obtained from the residual blood by centrifuging at 1,400 × g for 5 min. This study was approved by the Ethics Committee of Gifu University Graduate School of Medicine, Gifu, Japan. Written informed consent was obtained from all participants.
Platelet aggregation
Platelet aggregation was measured using an aggregometer (PA-200; Kowa Co. Ltd., Tokyo, Japan) with laser scattering, which can detect the light-transmittance and the size of platelet aggregates based upon particle counting (small: 9–25 μm, medium: 25–50 μm and large: 50–70 μm). In brief, PRP was pretreated at room temperature with various doses of HT or OLE for 15 min. After the pretreatment, PRP was pre-incubated for 1 min at 37°C with a stirring speed at 800 rpm. PRP was then stimulated by collagen, and platelet aggregation was monitored for 4 min. The dose of collagen was adjusted individually to achieve a percentage transmittance of 80%-100%. The percentage of isolated PRP was recorded as 0%, and that of the appropriate PPP (blank) was recorded as 100%.
Protein preparation after stimulation
After the stimulation with collagen, the platelet aggregation was terminated by adding an ice-cold EDTA (10 mM) solution. The mixture was collected and centrifuged at 10,000 × g at 4°C for 2 min. The supernatant was collected for each ELISA and stored at −30°C. The pellet was washed twice with phosphate-buffered saline (PBS) and then lysed by boiling in a lysis buffer containing 62.5 mM Tris-HCL, pH 6.8, 2% sodium dodecyl sulfate (SDS), 50 μM dithiothreitol and 10% glycerol for a Western blot analysis.
Western blot analysis
Western blotting was performed as described previously [Citation37]. Briefly, SDS-polyacrylamide gel electrophoresis was performed according to Laemmli [Citation38] on a 10% or 12.5% polyacrylamide gel. The proteins were fractionated and transferred onto a polyvinylidene difluoride (PVDF) membrane, which was then blocked with 5% fat-free dry milk in PBS with 0.1% Tween 20 (PBS-T; 10 mM Na2HPO4, 1.8 mM KH2PO4, pH 7.4, 137 mM NaCl, 2.7 mM KCL and 0.1% Tween 20) for 2 h before incubation with phospho-specific HSP27 antibodies, HSP27 antibodies or GAPDH antibodies as primary antibodies. Peroxidase-labeled anti-mouse IgG antibodies, anti-goat IgG antibodies or anti-rabbit IgG antibodies were used as secondary antibodies. The primary and secondary antibodies were diluted to optimal concentrations with 5% fat-free dry milk in PBS-T. The peroxidase activity on the PVDF membrane was visualized on X-ray film by an ECL Western blotting detection system (GE Healthcare, Buckinghamshire, UK) as described in the manufacturer’s protocol. A densitometric analysis was performed using a scanner and an imaging software program (Image J version 1.48; NIH, Bethesda, MD, USA). The phosphorylated levels were calculated as follows: the background-subtracted intensity of each signal was normalized to the respective intensity of GAPDH and plotted as the fold increase in comparison to the control cells without stimulation.
ELISA for PDGF-AB, sCD40L and phosphorylated-HSP27
The levels of PDGF-AB, sCD40L or phosphorylated-HSP27 in the supernatant of the conditioned mixture after platelet aggregation were determined using ELISA kits for PDGF-AB, sCD40L and phosphorylated-HSP27 respectively, in accordance with the manufacturer’s protocols.
Statistical analysis
The data were analyzed by the T test and the Mann-Whitney U test with JMP version 13.0.0 (SAS Institune, Inc, Cary, NC, USA). p < 0.05 was considered to indicate a statistically significant difference. The data are presented as the mean ± standard error of the mean (SEM).
Results
Effects of HT and OLE on collagen-stimulated human platelet aggregation
It has been reported that HT and OLE inhibit human platelet aggregation [Citation20,Citation21]. Thus, we first confirmed the effect of HT or OLE on the human platelet aggregation induced by collagen. The representative pattern of HT-effect on the collagen-stimulated human platelet aggregation is shown in , and that of OLE-effect in . We found that HT markedly suppressed the collagen-stimulated platelet aggregation in a dose-dependent manner at doses of 30–100 μM (). In addition, OLE remarkably reduced the platelet aggregation induced by collagen. In a previous report [Citation20], 100 µM of HT and 100 µM of OLE inhibited the platelet aggregation. However, in our experimental condition, 100 µM of HT had little effect in some cases, and 100 µM of OLE had little effect on the collagen-stimulated platelet aggregation (data not shown). Therefore, we used the dose of HT and OLE up to 150 µM and 500 µM, respectively. With regard to the size of the platelet aggregates, HT at a dose of 100 μM significantly increased the formation of small aggregates (9–25 μm) but decreased the formation of large aggregates (50–70 μm) ( and ). Additionally, OLE at dose of 500 μM also increased the formation of small aggregates but decreased the formation of large aggregates ().
Table 1. Effect of hydroxytyrosol (HT) on the collagen-stimulated aggregation of human platelets.
Figure 1. Effect of hydroxytyrosol (HT) on the collagen-stimulated aggregation of human platelets.
PRP was pretreated with indicated doses of HT at 37°C for 15 min, and then stimulated by collagen for 5 min. The black line indicates the percentage of transmittance of each sample (isolated platelets recorded as 0%, and PPP recorded as 100%). The blue line indicates small aggregates (9–25 μm); green line, medium aggregates (25–50 μm); red line, large aggregates (50–70 μm). The lower panel presents the distribution (%) of aggregated particle size as measured by laser scattering. The representative result obtained from three healthy donors is shown.
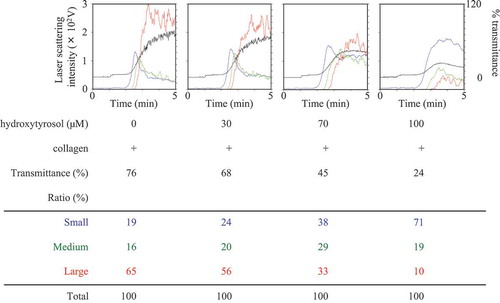
Figure 2. Effect of oleuropein (OLE) on the collagen-stimulated aggregation of human platelets.
PRP was pretreated with 500 μM of OLE or vehicle at 37°C for 15 min, and then stimulated by collagen for 5 min. The black line indicates the percentage of transmittance of each sample (isolated platelets recorded as 0%, and PPP recorded as 100%). The blue line indicates small aggregates (9–25 μm); green line, medium aggregates (25–50 μm); red line, large aggregates (50–70 μm). The lower panel presents the distribution (%) of aggregated particle size as measured by laser scattering. The representative result obtained from twelve healthy donors is shown.
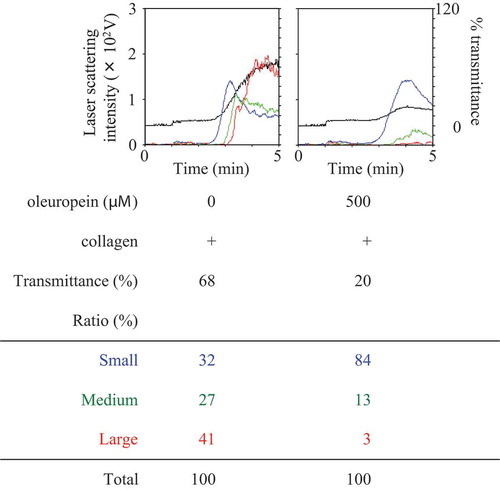
Effects of HT and OLE on the collagen-induced secretion of PDGF-AB and the release of sCD40L from human platelets
It is generally recognized that the human platelet activation induced by collagen leads to the secretion of PDGF-AB and the release of sCD40L.[Citation9,Citation13], Therefore, we investigated the effect of HT or OLE on the collagen-induced secretion of PDGF-AB and the release of sCD40L from human platelets. HT at dose of 100 μM, which by itself failed to affect PDGF-AB secretion or sCD40L release, significantly reduced the collagen-induced secretion of PDGF-AB and the release of sCD40L, and caused an approximately 60% and 55% reduction in the collagen-effect, respectively ()). OLE (500 μM), which alone had no effect on the secretion of PDGF-AB or the release of sCD40L, significantly suppressed the collagen-induced secretion of PDGF-AB and the release of sCD40L, and caused an approximately 80% and 90% reduction in the effect, respectively ()).
Figure 3. Effects of hydroxytyrosol (HT) on the collagen-induced secretion of PDGF-AB (a) or the release of sCD40L (b) from human platelets.
PRP was pretreated with 100 μM of HT or vehicle at 37°C for 15 min, and then stimulated by collagen or vehicle for 15 min. The reaction was terminated by addition of an ice-cold EDTA solution. The mixture was centrifuged at 10,000 × g at 4°C for 2 min, and the supernatant was then subjected to ELISA for PDGF-AB (a) and sCD40L (b). The results obtained from six (a) or seven (b) healthy donors are shown. Each value represents the mean ± SEM. *p < 0.05, compared to the value of control. **p < 0.05, compared to the value of collagen alone.
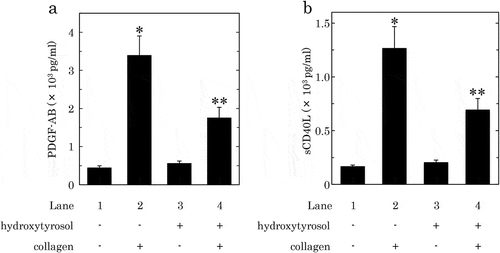
Figure 4. Effects of oleuropein (OLE) on the collagen-induced secretion of PDGF-AB (a) or the release of sCD40L (b) from human platelets.
PRP was pretreated with 500 μM of OLE or vehicle at 37°C for 15 min, and then stimulated by collagen or vehicle for 15 min. The reaction was terminated by addition of an ice-cold EDTA solution. The mixture was centrifuged at 10,000 × g at 4°C for 2 min, and the supernatant was then subjected to ELISA for PDGF-AB (a) and sCD40L (b). The results from five healthy donors are shown. Each value represents the mean ± SEM. *p < 0.05, compared to the value of control. **p < 0.05, compared to the value of collagen alone.
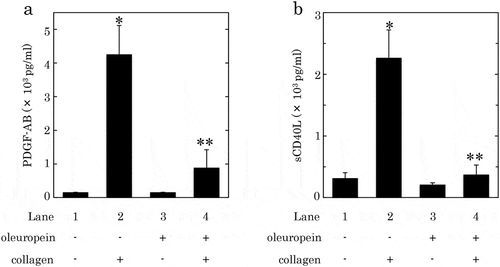
Effects of HT and OLE on the collagen-induced phosphorylation of HSP27 in human platelets
It has been reported that HT and OLE attenuate the 4-hydroxynonenal-induced phosphorylation of HSP27 in rat cardiomyocytes [Citation39]. Previous studies from our laboratories have demonstrated that the collagen-induced PDGF-AB secretion and sCD40L release are correlated with the phosphorylation of HSP27 in human platelets [Citation35]. Thus, we next examined the effect of HT or OLE on the collagen-induced phosphorylation of HSP27 in human platelets. HT at doses of 100–150 μM, which by itself hardly affected the phosphorylation of HSP27, significantly attenuated the collagen-induced phosphorylation of HSP27 ()). Additionally, OLE at a dose of 500 μM, which by itself had little effect on the phosphorylation of HSP27, significantly suppressed the collagen-induced phosphorylation of HSP27 ()).
Figure 5. Effects of hydroxytyrosol (HT) or oleuropein (OLE) on the collagen-induced phosphorylation of HSP27 in human platelets.
(a) PRP was pretreated with 100 μM (7 cases) or 150 μM (2 cases) of HT or vehicle at 37°C for 15 min, and then stimulated by collagen or vehicle for 120–300 s. (b) PRP was pretreated with 500 μM of OLE (4 cases) or vehicle at 37°C for 15 min, and then stimulated by collagen or vehicle for 120–150 s. The reaction was terminated by addition of an ice-cold EDTA solution. The lysed platelets were then subjected to Western blot analysis using antibodies against phospho-specific HSP27, HSP27, and GAPDH. The representative result of HT at a dose of 150 μM (a) or OLE at a dose of 500 μM (b) is presented. The histogram shows a quantitative representation of the collagen-induced levels obtained from a densitometric analysis of nine (a) or four (b) independent experiments. The phosphorylation is expressed as the fold increase compared to the basal levels, presented as lane 1. Each value was corrected by the level of GAPDH and represents the mean ± SEM. *p < 0.05, compared to the value of control. **p < 0.05, compared to the value of collagen alone.
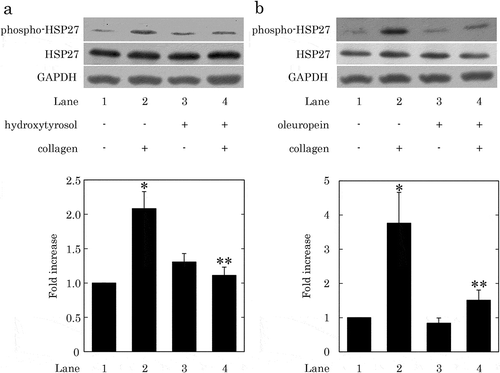
Effects of HT and OLE on the collagen-induced release of phosphorylated- HSP27 from human platelets
We have previously shown that phosphorylated HSP27 is released from human platelets accompanied with the collagen-induced phosphorylation of HSP27[Citation37]. Therefore, we further examined the effect of HT or OLE on the release of phosphorylated-HSP27 from the human platelets activated by collagen. HT (100 μM) significantly suppressed the collagen-induced release of phosphorylated-HSP27, and caused an about 75% reduction in the collagen-effect ()). OLE (500 μM) also significantly reduced the collagen-induced release of phosphorylated-HSP27, and caused an approximately 90% reduction in the effect ()).
Figure 6. Effects of hydroxytyrosol (HT) (a) or oleuropein (OLE) (b) on the collagen-induced release of phosphorylated-HSP27 from human platelets.
PRP was pretreated with 100 μM of HT (a), 500 μM of OLE (b) or vehicle at 37°C for 15 min, and then stimulated by collagen or vehicle for 15 min. The reaction was terminated by addition of an ice-cold EDTA solution. The mixture was centrifuged at 10,000 × g at 4°C for 2 min, and the supernatant was then subjected to ELISA for phosphorylated-HSP27. The results from eight (a) or five (b) healthy donors are shown. Each value represents the mean ± SEM. *p < 0.05, compared to the value of control. **p < 0.05, compared to the value of collagen alone.
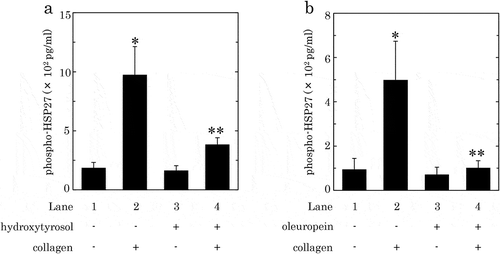
Discussion
In the present study, we investigated the effects of HT and OLE, which are major polyphenolic components of extra virgin olive oil [Citation2], on the collagen-induced activation of human platelets. It is well recognized that the activation of human platelets induces the secretion of PDGF-AB from α-granule and the release of sCD40L [Citation9,Citation13]. We herein demonstrated that both HT and OLE inhibited the secretion of PDGF-AB and the release of sCD40L from the human platelets activated by collagen. Using an aggregometer with laser scattering, we confirmed that both HT and OLE suppressed the collagen-stimulated aggregation of human platelets, which is in accordance with the previous reports [Citation20,Citation21]. In our present study, the doses of OLE and HT we used were higher than those used in a previous report [Citation20]. Both 500 µM OLE and 150 µM HT attenuated the collagen-induced platelet aggregation, whereas the formation of small aggregates was truly induced. Based on our findings, it is probable that the suppression by OLE or HT is due to the specific effect but not toxic effect on the platelet function. Therefore, it is most likely that HT and OLE attenuate the granule secretion of PDGF-AB and the release of sCD40L from the collagen-activated human platelets. It is firmly established that PDGF-AB is a potent mitogenic growth factor, which mainly acts on connective tissues including vascular smooth muscle cells and promotes atherosclerosis [Citation16]. On the other hand, the released sCD40L activates CD40 on vascular endothelium cells and neutrophils, and induces pro-inflammatory and pro-atherosclerotic responses [Citation17–Citation19]. High plasma levels of sCD40L are reportedly associated with an increased risk of cardiovascular events in patients with unstable coronary artery disease [Citation40]. Based on these findings including our present results, HT and OLE could be beneficial for prevention of atherosclerosis-related diseases such as acute coronary syndrome and stroke through reducing PDGF-AB secretion and sCD40L release from activated platelets.
We have previously shown that the collagen-induced phosphorylation of HSP27 in human platelets is closely related with the secretion of PDGF-AB and the release of sCD40L.[Citation35] In addition, we recently demonstrated that phosphorylated HSP27 is released from the collagen-stimulated human platelets accompanied with its phosphorylation [Citation37]. On the basis of our findings, we next examined the effects of HT and OLE on the collagen-induced HSP27 phosphorylation in human platelets. We demonstrated that both HT and OLE significantly suppressed the collagen-induced phosphorylation of HSP27 in human platelets. Additionally, both HT and OLE markedly reduced the collagen-stimulated release of phosphorylated-HSP27 from human platelets into plasma. Taking these findings into account, it is most likely that both HT and OLE attenuate the collagen-induced phosphorylation of HSP27 in human platelets, resulting in the reduction of the phosphorylated-HSP27 release into plasma. To the best of our knowledge, this is probably the first report showing the suppressive effect of olive polyphenol on the release of phosphorylated-HSP27 from human platelets. Further investigations are necessary to investigate the exact mechanism of olive polyphenols in human platelets.
Evidence is accumulating that HSP27 extracellularly acts especially as a potent modulator of inflammation in addition to intracellular functions as a molecular chaperone in proteostasis [Citation23,Citation25]. It has been shown that HSP27 activates the nuclear factor κB signaling pathway in macrophages, resulting in the up-regulated expression pro-inflammatory cytokines such as interleukin-1β and tumor necrosis factor-α, and the secretion [Citation34]. In addition, HSP27 released from human myocardium by global ischemia reportedly has pro-inflammatory effects through toll-like receptors in human coronary vascular endothelium cells [Citation41]. It is generally recognized that inflammation is closely related to the progression of atherosclerosis, and the trigger of platelet activation is tethering at the sites of vascular injury in chronic inflammatory atherosclerotic lesions, which expose subendothelial collagen [Citation9,Citation10]. Regarding the plasma concentration of OLE and HT in human, 270 nM of OLE and 30 nM of HT are reportedly detected after oral ingestion of olive leaf extract and olive oil, respectively, which are markedly lower than the doses used in our experiments [Citation42,Citation43]. Based on these findings, it seems likely that olive polyphenols such as HT and OLE possess the possibilities of anti-atherosclerotic effects through the suppression of HSP27 release from human platelets activated by collagen, in addition to the inhibition of PDGF-AB secretion and sCD40L release. Taking account of these findings as a whole, it is possible that olive oil consumption might reduce the risk of atherothrombotic diseases including acute coronary syndrome and stroke, at least in part, via the suppression of released HSP27 from activated human platelets. Further investigations are needed to clarify the precise roles of released HSP27 from human platelets.
Taken together, our findings strongly suggest that olive polyphenol reduces the collagen-stimulated phosphorylation of HSP27 in human platelets and the release of phosphorylated HSP27. Our present results may provide a novel anti-inflammatory effect of olive polyphenol.
Author contribution
K.T., H.T., S.O., H.I., T.I., and O.K. conceived and designed the experiments. D.M., T.O., A.K., K.U., D.N., T.D., Y.E., and R.M-N. performed the experiments. D.M., T.O., H.T., R.M-N., and O.K. analyzed the date. D.M., H.T., S.O., H.I., T.I., and O.K. wrote the paper. All authors read and approved the final manuscript.
Acknowledgments
We are very grateful to Mrs. Yumiko Kurokawa for her skillful technical assistance. This investigation was supported in part by a Research Funding for Longevity Science (19-21) from National Center for Geriatrics and Gerontology, Japan.
Disclosure statement
No potential conflict of interest was reported by the authors.
Additional information
Funding
References
- Rodriguez-Morato J, Xicota L, Fito M, et al. Potential role of olive oil phenolic compounds in the prevention of neurodegenerative diseases. Molecules. 2015;20:4655–4680.
- Visioli F, Galli C. Biological properties of olive oil phytochemicals. Crit Rev Food Sci. 2002;42(3):209–221.
- Vissers MN, Zock PL, Roodenburg AJ, et al. Olive oil phenols are absorbed in humans. J Nutr. 2002;132:409–417.
- Echeverria F, Ortiz M, Valenzuela R, et al. Hydroxytyrosol and cytoprotection: A projection for clinical interventions. Int J Mol Sci. 2017;18:E930.
- Barbaro B, Toietta G, Maggio R, et al. Effects of the olive-derived polyphenol oleuropein on human health. Int J Mol Sci. 2014;15:18508–18524.
- Rigacci S, Stefani M. Nutraceutical properties of olive oil polyphenols. An itinerary from cultured cells through animal models to humans. Int J Mol Sci. 2016;17:E843.
- Covas MI, Nyyssonen K, Poulsen HE, et al. The effect of polyphenols in olive oil on heart disease risk factors: a randomized trial. Ann Int Med. 2006;145:333–341.
- Estruch R, Ros E, Salas-Salvado J, et al. Primary prevention of cardiovascular disease with a mediterranean diet supplemented with extra-virgin olive oil or nuts. New Engl J Med. 2018;378:e34.
- Davi G, Patrono C. Platelet activation and atherothrombosis. New Engl J Med. 2007;357:2482–2494.
- Furie B, Furie BC. Mechanisms of thrombus formation. New Engl J Med. 2008;359:938–949.
- Nieswandt B, Watson SP. Platelet-collagen interaction: is GPVI the central receptor? Blood. 2003;102:449–461.
- Moroi M, Jung SM. Platelet glycoprotein VI: its structure and function. Thromb Res. 2004;114:221–233.
- Li Z, Delaney MK, O’Brien KA, et al. Signaling during platelet adhesion and activation. Arterio Thromb Vas Biol. 2010;30:2341–2349.
- Gibbins JM, Okuma M, Farndale R, et al. Glycoprotein VI is the collagen receptor in platelets which underlies tyrosine phosphorylation of the Fc receptor gamma-chain. FEBS Lett. 1997;413:255–259.
- Shattil SJ, Newman PJ. Integrins: dynamic scaffolds for adhesion and signaling in platelets. Blood. 2004;104:1606–1615.
- Heldin CH, Westermark B. Mechanism of action and in vivo role of platelet-derived growth factor. Physiol Rev. 1999;79:1283–1316.
- Henn V, Slupsky JR, Grafe M, et al. CD40 ligand on activated platelets triggers an inflammatory reaction of endothelial cells. Nature. 1998;391:591–594.
- Hermann A, Rauch BH, Braun M, et al. Platelet CD40 ligand (CD40L)-subcellular localization, regulation of expression, and inhibition by clopidogrel. Platelets. 2001;12:74–82.
- Andre P, Nannizzi-Alaimo L, Prasad SK, et al. Platelet-derived CD40L: the switch-hitting player of cardiovascular disease. Circulation. 2002;106:896–899.
- Petroni A, Blasevich M, Salami M, et al. Inhibition of platelet aggregation and eicosanoid production by phenolic components of olive oil. Thromb Res. 1995;78:151–160.
- Correa JA, Lopez-Villodres JA, Asensi R, et al. Virgin olive oil polyphenol hydroxytyrosol acetate inhibits in vitro platelet aggregation in human whole blood: comparison with hydroxytyrosol and acetylsalicylic acid. Brit J Nutr. 2009;101:1157–1164.
- Benjamin IJ, McMillan DR. Stress (heat shock) proteins: molecular chaperones in cardiovascular biology and disease. Circ Res. 1998;83:117–132.
- Bakthisaran R, Tangirala R, Rao CM. Small heat shock proteins: role in cellular functions and pathology. Biochim Biophys Acta. 2015;1854:291–319.
- Kampinga HH, Hageman J, Vos MJ, et al. Guidelines for the nomenclature of the human heat shock proteins. Cell Stress Chaperon. 2009;14:105–111.
- Mymrikov EV, Seit-Nebi AS, Gusev NB. Large potentials of small heat shock proteins. Physiol Rev. 2011;91:1123–1159.
- Landry J, Lambert H, Zhou M, et al. Human HSP27 is phosphorylated at serines 78 and 82 by heat shock and mitogen-activated kinases that recognize the same amino acid motif as S6 kinase II. J Biol Chem. 1992;267:794–803.
- Acunzo J, Katsogiannou M, Rocchi P. Small heat shock proteins HSP27 (HspB1), αB-crystallin (HspB5) and HSP22 (HspB8) as regulators of cell death. Int J Biochem Cell Biol. 2012;44:1622–1631.
- Kato K, Hasegawa K, Goto S, et al. Dissociation as a result of phosphorylation of an aggregated form of the small stress protein, hsp27. J Biol Chem. 1994;269:11274–11278.
- Kostenko S, Moens U. Heat shock protein 27 phosphorylation: kinases, phosphatases, functions and pathology. Cell Mol Life Sci. 2009;66:3289–3307.
- Merendino AM, Paul C, Vignola AM, et al. Heat shock protein-27 protects human bronchial epithelial cells against oxidative stress-mediated apoptosis: possible implication in asthma. Cell Stress Chaperon. 2002;7:269–280.
- Bruey JM, Ducasse C, Bonniaud P, et al. Hsp27 negatively regulates cell death by interacting with cytochrome c. Nature Cell Biol. 2000;2:645–652.
- Arrigo AP. The cellular “networking” of mammalian Hsp27 and its functions in the control of protein folding, redox state and apoptosis. Adv Exp Med Biol. 2007;594:14–26.
- Arrigo AP, Gibert B. HspB1, HspB5 and HspB4 in human cancers: potent oncogenic role of some of their client proteins. Cancers (Basel). 2014;6:333–365.
- Salari S, Seibert T, Chen YX, et al. Extracellular HSP27 acts as a signaling molecule to activate NF-κB in macrophages. Cell Stress Chaperon. 2013;18:53–63.
- Kato H, Adachi S, Doi T, et al. Mechanism of collagen-induced release of 5-HT, PDGF-AB and sCD40L from human platelets: role of HSP27 phosphorylation via p44/p42 MAPK. Thromb Res. 2010;126:39–43.
- Kato H, Takai S, Matsushima-Nishiwaki R, et al. HSP27 phosphorylation is correlated with ADP-induced platelet granule secretion. Arch Biochem Biophys. 2008;475:80–86.
- Tokuda H, Kuroyanagi G, Tsujimoto M, et al. Release of phosphorylated HSP27 (HSPB1) from platelets is accompanied with the acceleration of aggregation in diabetic patients. PLoS One. 2015;10:e0128977.
- Laemmli UK. Cleavage of structural proteins during the assembly of the head of bacteriophage T4. Nature. 1970;227:680–685.
- Bali EB, Ergin V, Rackova L, et al. Olive leaf extracts protect cardiomyocytes against 4-hydroxynonenal-induced toxicity in vitro: comparison with oleuropein, hydroxytyrosol, and quercetin. Planta Med. 2014;80:984–992.
- Heeschen C, Dimmeler S, Hamm CW, et al. Soluble CD40 ligand in acute coronary syndromes. New Engl J Med. 2003;348:1104–1111.
- Jin C, Cleveland JC, Ao L, et al. Human myocardium releases heat shock protein 27 (HSP27) after global ischemia: the proinflammatory effect of extracellular HSP27 through toll-like receptor (TLR)-2 and TLR4. Mol Med. 2014;20:280–289.
- Nikolaos L, Vassilis M, Maria H, et al. A novel bioanalytical method based on UHPLC-HRMS/MS for the quantification of oleuropein in human serum. Application to a pharmacokinetic study. Biomed Chromatogr. 2016;30:2016–2023.
- Antoni P, Jose R-M, Eulalia O, et al. Analysis of free hydroxytyrosol in human plasma following the administration of olive oil. J Chromatgr A. 2016;1437:183–190.