ABSTRACT
The microbial TGase from Streptomyces mobaraensis has used in various food industries. However, the detailed substrate specificities of TGases from the Streptomyces species toward the natural peptides remains to be unclear. In this study, we conducted the comparison of two different TGases from Streptomyces mobaranensis (SMTG) and Streptomyces cinnamoneus (SCTG). To clarify the region associated with the characteristics of enzymes, we constructed a chimeric enzyme of CM, of which is consisted of N-terminal half of SCTG and C-terminal half of SMTG. To reveal the differences in the substrate specificity between SCTG and SMTG toward natural peptides, we investigated the time dependence of TGase activity on the productivity of cross-linking peptide with tryptic casein and lysine by using LC-MS. We identified two peptides of “VLPVPQK” and “AVPYPQR” as substrates for both of the TGases.
Graphical abstract
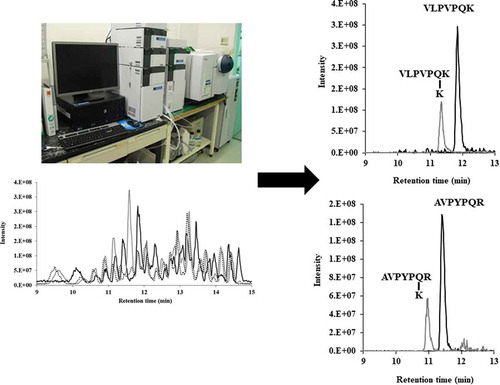
To reveal the mechanism of substrate recognition of Streptomyces transglutaminases, we constructed a chimeric enzyme. And we identified “VLPVPQK” and “AVPYPQR” as their substrates.
The protein cross-linking enzyme, transglutaminase (TGase), catalyzes an acyl transfer reaction between the γ-carboxyamide group of a glutamine residue on peptide as acyl donor and a several primary amines including lysine as acyl acceptor. Various organisms produce TGases, which are divided into two types of calcium dependent or independent of activity. The former type of TGase is distributed in plants and vertebrate, which have many physiological roles such as cell wall construction [Citation1] and blood clotting involves factor XIII [Citation2,Citation3], respectively. The latter type is microbial TGase first isolated from Streptomyces mobaraensis [Citation4].
Because bacterial TGase has calcium-independent activity, the microbial TGase from Streptomyces mobaraense has used in various food industry [Citation5], such as cheese and ice cream product [Citation6,Citation7], meat processing [Citation8,Citation9] and bakery products [Citation10]. In addition, a new detoxification method for allergen protein have attempted by cross-linking activity of TGase [Citation11–Citation13].
In three-dimensional structures of both TGase, they form different shapes, bacterial TGase is folded like a disk with a deep active cleft [Citation14] whereas that other targets such as human factor XIII and fish TGase is folded into four sequential domains: N-terminal β-sandwich domain, a catalytic core domain, and two β-barrels [Citation15,Citation16]. These structural differences likely affected not only the calcium dependent activity, but also the substrate specificity [Citation17]. Compared to vertebrate TGases, bacterial TGases show broad substrate specificity toward amine substrate as acyl acceptor. Although high similarity among the amino acid sequences in Streptomyces TGases, they show different activities and TGase from Streptomyces mobaraensis reported as a highly active enzyme [Citation18]. Taguchi et al., reported that several mutations in active site enhanced the substrate reactivity [Citation19]. However, the ideal natural peptide contained Gln residue for the Streptomyces TGase remains to be unclear. In addition, the region of TGase plays an important role in substrate recognition is also unclear. In this study, we conducted the comparison analysis for characteristics of two different TGases from Streptomyces mobaranensis and Streptomyces cinnamoneus.
Materials and methods
Materials, bacterial strains, and plasmids
Benzyloxy-carbonyl (CBZ) -Gln-Gly was purchased from the Peptide Institute, Inc. (Osaka). Qubit Protein Assay Kit was purchased from Thermo Fisher Scientific, Inc. (Waltham). The transglutaminases, SCTG from S. cinnamoneus NBRC13864, SMTG from S. mobaraensis NBRC13819 and a chimeric enzyme CM were expressed in Streptomyces lividans 1326 by using the expression vector, pTONA short, which was harboring a minimum region (3ʹ-terminal of 68bp) of SSMP promoter [Uraji, M et al., in preparation]. The plasmid pCR®-Blunt II-TOPO (Thermo Fisher scientific, Carlsbad, CA) was used for cloning of SCTG and SMTG genes.
Cloning of SMTG and SCTG genes
Genomic DNA was prepared from S. mobaraensis NBRC13819 and S. cinnamoneus NBRC13864 by the method of Hopwood et al., [Citation20]. SMTG gene was amplified by polymerase chain reaction (PCR) using the sense primer 5ˈ-CATATGCGCATACGCCGGAGAGC-3ˈ (the underlined region represents the NdeI site and includes the initiation codon) and the antisense primer 5ˈ-AAGCTTCACGGCCAGCCCTG CTTTAC-3ˈ (the underlined region represents the HindIII site and is adjacent to the stop codon). PCR was performed using the PrimeStar GXL DNA Polymerase system (Takara, Japan). The PCR product (1.2 kb) was cloned into the pCR-Blunt II-TOPO Vector system (Invitrogen, USA) and correct cloning was confirmed by sequencing by comparison with the registered sequence (Genbank accession No. AY615816).
Sequencing of the genomic DNA of S. cinnamoneus NBRC13864 was performed using a MinION next-generation sequencer (Oxford Nanopore Technologies, England). The sequence reads were assembled into a draft genome sequence (ca. 7.2 Mbp) using CLC Genomics Workbench (CLC Bio, Aarhus, Denmark). SCTG gene was identified by homology search with the GENETYX software (GENETYX Corp., Japan). SCTG gene was registered with the DDBJ data bank (Genbank accession No. AY615816). SCTG gene was included NdeI site (nucleotide number 48–53). To delete the inside NdeI site, we synthesized an artificial gene with a silent mutation by GeneArt service (Thermo Fischer SCIENTIFIC, Japan). The synthetic gene was cloned in the SfiI site of pMA vector (pMA/SCTG). The synthetic gene was added two restriction sites of NdeI, which was including the initiation codon, and HindIII, which was located downstream of the stop codon.
Construction of expression plasmids of chimeric enzyme
5ʹ terminal half of SCTG gene was amplified by PCR using the sense primer 5ˈ-CATATGCATCAACGTCGGAGATTG-3ˈ (the underlined region represents the NdeI site and includes the initiation codon) and the antisense primer 5ˈ-GCGTTTCCCCGGACCGGGGGCTGGT-3ˈ (the underlined region represents CpoI site. The PCR products were cloned using the Zero Blunt® TOPO® PCR Cloning kit (Thermo Fisher scientific, Carlsbad, CA). The cloned gene of extracting plasmid was confirmed by sequencing. The 5ʹ terminal half of SMTG gene in pCR-Blunt II-TOPO was exchanged with the NdeI-CpoI fragment SCTG gene. The resulting chimeric gene was cloned into the gap of NdeI-HindIII of pTONA short. A chimeric enzyme, CM was consisted of N-terminal half of SCTG and C-terminal half of SMTG. Another chimera, which was consisted of N-terminal half of SMTG and C-terminal half of SCTG, was also constructed, but this chimera was not expressed by using pTONA short and S. lividans 1326 strain. Thus, the present study was aimed to reveal the mechanism of substrate recognition Streptomyces TGases in comparison of SMTG, SCTG, and chimeras of CM.
Expression and purification of TGases
Streptomyces lividans 1326 harboring the expression vector for TGase production was cultivated at 30°C with shaking at 180 rpm for 5 days in 50 mL of expressive medium (2% glycerol, 0.8% potassium phosphate, 0.05% magnesium chloride, 0.5% yeast extract, and 0.5% polypeptone) containing 50 μg·mL−1 kanamycin. By using this medium, recombinant TGases were secreted as mature forms. The Streptomyces TGase was secreted as a zymogen with an additional prosequence at the N-terminus. This zymogen became active after proteolytic cleavage of the prosequence. After removal of the cells, the supernatant was brought to 70% saturation with ammonium sulfate. The resultant precipitate was dissolved in 20 mM potassium phosphate buffer (KP buffer) (pH 8.0) and then dialyzed against 20 mM KP buffer (pH 8.0). The dialyzate was loaded onto a Vivapure-Q spin column (Sartorius) equilibrated with 20 mM KP buffer (pH 8.0). The pass solution with high activity was used as the purified enzyme solution.
Enzyme assay
For the assay of transglutaminase activity, it was determined by using high-performance liquid chromatography (HPLC) and LC-mass spectrometry (MS). In the assay, 5 μL of 0.1 mg·mL−1 enzyme solution was added to 45 μL of a mixture containing of 182 mM KP buffer (pH 8.0), 100 mM hydroxylamine, 10 mM glutathione was added as an anti-oxidative reagent, and 20 mM CBZ-Gln-Gly in PCR tube at 37°C. The reaction mixture was incubated for 30 min at 37°C, and the reaction was stopped by heat treatment (95°C, 5 min).
Components of the reaction mixture were separated by HPLC with a Symmetry C18 column (3.5 mm, 2.1 mm× 50 mm; Waters; Milford, MA, USA) maintained at 40°C. The flow rate of the mobile phase, which consisted of (a) Milli-Q with 0.1% formic acid, and (b) acetonitrile with 0.1% formic acid, was 0.5 mL/min. The gradient profile was as follows: 0–5 min, 10% B; 5–8 min, 10–70% B; 8–10 min, 70–95% B; and 10.01–12 min, 10% B. The separated products were detected at 254 nm. The products were confirmed by using an ion trap MS apparatus (Amazon SL; BRUKER,) in the positive-ion mode.
Optimal pH and pH stability
Effect of pH on the activity toward CBZ-Gln-Gly as acyl donor and hydroxylamine as acyl acceptor were examined at 25°C in potassium phosphate (pH 6.0–8.0), Tris–HCl (pH 8.0–9.0), or carbonate–bicarbonate (pH 10.0). In assay of pH stability, the enzymes were treated with each pH buffer for 10 min at 37°C. Specific activities were measured under the condition described in the “Enzyme assay” subsection. The reaction mixture was incubated for 30 min, and the reaction was stopped by heat treatment (95°C, 5 min).
Thermal stability and optimal temperature
Thermal stability and temperature dependence of activity toward CBZ-Gln-Gly as acyl donor and hydroxylamine as acyl acceptor were examined in potassium phosphate buffer (pH 8.0) at 10–80°C. In assay of thermal stability, the enzymes are treated with each temperature for 10 min before addition to the mixture. We conducted to compare thermal stabirities of the three TGases by 10 min-incubation because thermal stabilities of SCTG and CM were lower than of SMTG. Specific activities were measured under the conditions described in the “Enzyme assay” subsection. The reaction mixture was incubated for 30 min, and the reaction was stopped by heat treatment (95°C, 5 min).
Enzyme kinetics
The Km and kcat values of TGases for the catalysis of lysine as acyl acceptor with CBZ-Gln-Gly as acyl donor were measured by conducting the reactions at different concentrations of lysine (5–40 mM) under the conditions described in subsection “Enzyme assay” substituted the acyl acceptor of hydroxylamine with lysine and constructing Lineweaver-Burk plots. The reaction mixture was incubated for 30 min at 37°C, and the reaction was stopped by heat treatment (95°C, 5 min). In experiments using CBZ-Gln-Gly or lysine, the Km and kcat values were estimated by plotting “s vs. s/v” or “1/s vs. 1/v,” respectively.
Activity of TGases toward tryptic casein
The tryptic casein dissolved in 50 mM NH4HCO3 (pH7.0) had been prepared in before study[Citation21] and the solution was used as a crude substrate containing various peptides in this study. 20 μL of 0.1 mg·mL−1 TGase solution was added to 80 μL of a mixture containing 55 μL of 200 mM potassium phosphate buffer (pH 8.0) and 20 μL of 10 mg·mL−1 tryptic casein solution and 5 μL of 200 mM lysine solution in a PCR tube at room temperature. After incubating the reaction mixture for 30 min to 360 min at 37°C, the mixture was diluted with 0.1% formic acid in water containing 0.02 mM 4-methoxybenzamide as internal standard[Citation22]. The appeared products were detected by LC ESI-MS with the TSKgel ODS-120H (3 μm, 2.0 mm × 15 cm; Tosoh Corporation, Japan) maintained at 50°C. The separated reaction solutions were analyzed using an ion trap MS apparatus (Amazon SL; BRUKER. The flow rate of the mobile phase, which consisted of (a) Milli-Q with 0.1% formic acid, and (b) acetonitrile with 0.1% formic acid, was 0.25 mL/min. The gradient profile was as follows: 0–5 min, 5% B; 5–15 min, 5–70% B; 15–15.1 min, 70–95% B; 15.1–20 min, 95% B; 20–20.1 min, 95–10% B; and 20.1–25 min, 10% B. In MS analyses, data were recorded in the 100–800 m/z range.
Results
Characterization of TGase activity
We investigated the effects of pH and temperature on the activities of SCTG, SMTG and CM. As shown in , different optimum pH was observed between SCTG and the others. The optimum pH of SCTG activity was at pH 8.0 whereas that SMTG and CM were shifted to the side of alkali at pH9.0 (). The pH of SMTG and CM also demonstrated similar profiles (). In contrast, optimal temperatures of SCTG and CM activities were at 40°C and 45.4°C, respectively, whereas that SMTG was higher than them at 50.9°C ()). In addition, the thermal stabilities of SCTG and CM showed similar Tm values of 45.8°C and 46.0°C, respectively, whereas that that of SMTG showed higher Tm value of 51.7°C ()). The Tm value is the temperature at which 50% of the activity when stored on ice is lost after heat treatment.
Figure 1. Optimum pHs of TGase activities.
(a), (b), and (c) show the results of SCTG, SMTG, and CM, respectively. Symbols: ○, KP buffer, ▴, Tris-HCl buffer, ●, Carbonate-bicarbonate buffer.
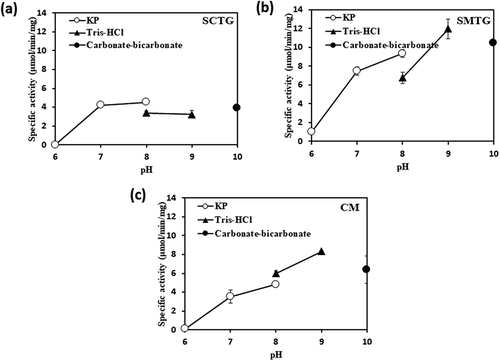
Kinetic study
As summarized in , the substrate affinities and the reaction rates toward substrates were increased SCTG, CM and SMTG, in that order. The Vmax of SCTG, SMTG and CM for CBZ-Gln-Gly were 36.6, 16.1, and 16.8 μmol·min−1·mg−1, respectively, indicating that the TGases have little differences in reaction rates. In contrast, the Km of SCTG for CBZ-Gln-Gly was 61.9 mM, approximately sixfold that of SMTG. Because of the low substrate affinity of SCTG, the activity toward to CBZ-Gln-Gly was likely less than that of SMTG. This result was agreed to before study[Citation18]. In addition, these enzymes showed the similar Km values for lysine each other.
Table 1. Kinetic parameters.
Substrate specificity of SCTG, SMTG, and CM in tryptic casein
To reveal the differences in the substrate specificity between SCTG and SMTG for natural peptide, we investigated the time dependence of TGase activity on the productivity of cross-linking peptide with tryptic casein by LC-MS ()). As shown in , we identified two pairs of substrate and its product peptides; 390.7 (charge +2; retention time 11.8 min) and 455.3 (charge +2; retention time 11.4 min), 415.7 (charge +2; retention time 11.4 min) and 480.2 (charge +2; retention time 11.0 min), which all peptides were detected as a divalent cation by MS analysis. The substrates with m/z of 390.7 and 415.7 gradually decreased, whereas the products with m/z of 455.3 and 480.2 gradually increased as the treatment time increased (). In before our study, we have identified the peptide with m/z of 390.7 as the amino acid sequence of allergen and bitter peptide VLPVPQK in the β-casein [Citation22]. In addition, we identified the peptide with m/z of 415.7 as AVPYPQR from the β-casein by comparison with the retention time of synthesized peptide AVPYPQR. These peptides were common substrate of both TGases SCTG and SMTG although the products of these peptides bound lysine are less in SCTG than in SMTG and CM. This result indicated that the region latter half of amino acid sequence of the TGase is associated with substrate recognition.
Figure 4. (a) Toal ion chromatographies of tryptic casein (black line), by using SCTG with L-Lys (grey line), and by using SMTG grey hached line).
(b) LC-MS analysis of substrate of VLPVPQK (black line) and its product (grey line). (c) LC-MS analysis of substrate of AVPYPQR (black line) and its product (grey line).
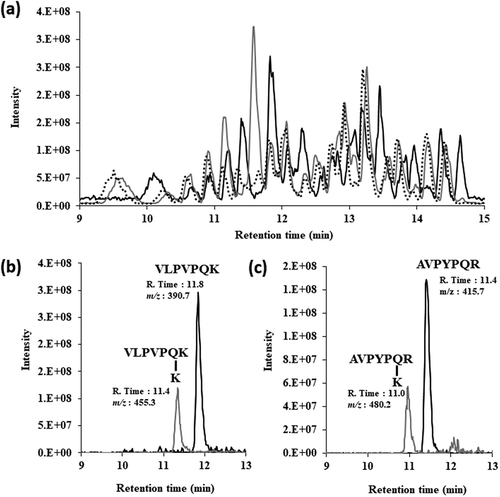
Discussion
Microbial TGases, especially Streptomyces TGases, have been useful in food processing [Citation23]. SMTG has been reported as the highest activity among Streptomyces TGases [Citation18]. And a TGase from Streptomyces mobaraensis strain S-8112 [Citation4] has been produced as a commercial enzyme of “Activa®”, and the enzyme has been used in food industry around the world.
In the present study, a newly cloned TGase gene from S. cinnamoneus NBRC13864 was very similar to another TGase from S. cinnamoneus NBRC12852 (accessetion No. AB085698) [Citation24] in the primary structures, which identity of mature forms was 93%. Thus, among S. cinnamoneus strains, TGases might be highly conserved. By comparison between SCTG and SMTG, SMTG was higher than SCTG in the activity in spite of highly similarity in their primary structures
It is similar to property of the chimeras of CM to SMTG in optimum pH, while the enyme indicated similar to SCTG in optimum temperature. From these results, we guess that the C-terminal half in Streptomyces TGases has an important role in optimum pH, and the Nterminal half was responsible in optimum temperature.
It has been known as a general method using a pair of CBZ-Gln-Gly as an acyl donor and hydroxylamine as an acyl acceptor for TGase activity. But, CBZ-Gln-Gly must be used at relatively low concentration in the method because of its slightly water-soluble. For instance, Langston et al., have used CBZ-Gln-Gly at a final concentration of 3 mM to compare 6 Streptomyces TGases[Citation18]. They have reported that a TGase from Streptomyces mobaraensis showed the highest activity (3.9 IU/mg), and one from Streptomyces cinnamoneus was the lowest (0.18 IU/mg)[Citation18]. In the present study, we have been using CBZ-Gln-Gly at a final concentration of 10 mM, and SCTG indicated low affinity toward CBZ-Gln-Gly compared with that of SMTG (). The chimeras of CM indicated middle properties between SCTG and SMTG on the Km value toward CBZ-Gln-Gly. Tagami et al., has reported that CBZ group was located in the near vestibule of the active cleft. Whereas Gly residue of the substrate was opposite in the front view of SMTG, where the left side wall of the cleft consisted of an N-terminal loop and the loop 276–288[Citation25]. In addition, they have speculated that the flexibility of the right side wall, which consisted of the loop 239–253, of the active cleft might decrease the streic hindrance between SMTG and CBZ-Gln-Gly[Citation25]. The loop of 239–253 and the loop of 276–288 were located in the C-terminal of SMTG and the chimera. In the two loops, SCTG possessed similar sequences to those of SMTG (). The obtained results considered, the fine conformation among two loops have an important role in Streptomyces TGase.
Figure 6. Comparison of primary structures between SCTG and SMTG on their mature forms.
The active Cys is indicated by an asterisk, and the underlined region represents CpoI site, which was used for construction of chimeric enzyme. Boxes indicate the loop of 239–253 and the loop of 276–288, which were located in the C-terminal of SMTG[Citation25]).
![Figure 6. Comparison of primary structures between SCTG and SMTG on their mature forms.The active Cys is indicated by an asterisk, and the underlined region represents CpoI site, which was used for construction of chimeric enzyme. Boxes indicate the loop of 239–253 and the loop of 276–288, which were located in the C-terminal of SMTG[Citation25]).](/cms/asset/d9f3ec82-e933-45b2-befc-64854187a087/tbbb_a_1697198_f0006_b.gif)
By using tryptic casein and L-Lys, we succeeded to identify the peptides of VLPVPQK and AVPYPQR as acyl donors for Streptomyces TGases. Interestingly, SMTG and chimeras of CM had almost the same action toward tryptic casein and L-Lys (). On the other hand, SCTG showed different from the two TGases. Namely, at the end of reaction, both of substrate peptides were disappeared by using three enzymes, however, the resulting cross-linked peptides by using SCTG was about half by using SMTG and CM in quantity. We guessed that cross-linking of VLPVPQK and AVPYPQR with other peptides might be occurred by using SCTG. Unfortunately, we could not identify these cross-linked peptides. From the results of kinetics study toward Lys as an acyl acceptor, the Km value of SCTG was the smallest among TGases, and that of CM was middle out of the three. These data leads that the N-terminal half of Streptomyces TGases might be responsible for the recognition of acyl acceptors. Further work is needed to identify the residue(s) responsible for the substrate recognition.
Author contribution
SH, MU, and TH conceived the project. SH, MU, and TH designed the experiments. SH, MU, and TH performed the experiments. SH, and TH wrote the manuscript.
Disclosure statement
No potential conflict of interest was reported by the authors.
Additional information
Funding
References
- Serafini-Fracassini D, Del Duca S. Transglutaminases: widespread cross-linking enzymes in plants. Ann Bot. 2008;102(2):145–152.
- Aeschlimann D, Paulsson M. Transglutaminases: protein cross-linking enzymes in tissues and body fluids. Thromb Haemost. 1994;71(4):402–415.
- Severina E, Nunez L, Baker S, et al. Factor XIIIa mediated attachment of S. aureus fibronectin-binding protein A (FnbA) to fibrin: identification of Gln103 as a major cross-linking site. Biochemistry. 2006;45(6):1870–1880.
- Ando H, Adachi M, Umeda K, et al. Purification and characteristics of a novel transglutaminase derived from microorganisms. Agric Biol Chem. 1989;53:2613–2617.
- Kieliszek M, Misiewicz A. Microbial transglutaminase and its application in the food industry. Rev Folia Microbiol (Praha). 2014;59(3):241–250.
- Taghi Gharibzahedi SM, Koubaa M, Barba FJ, et al. Recent advances in the application of microbial transglutaminase crosslinking in cheese and ice cream products: A review. Int J Biol Macromol. 2018;107(Pt B):2364–2374.
- Temiz H, Dağyıldız K. Effects of microbial transglutaminase on physicochemical, microbial and sensorial properties of kefir produced by using mixture cow’s and soymilk. Korean J Food Sci Anim Resour. 2017;37(4):606–616.
- Li Q, Gui P, Huang Z, et al. Effect of transglutaminase on quality and gel properties of pork and fish mince mixtures. J Texture Stud. 2018;49(1):56–64.
- Hu Y, Shao Y, Wu C, et al. γ-PGA and MTGase improve the formation of ε-(γ-glutamyl) lysine cross-links within hairtail (Trichiurus haumela) surimi protein. Food Chem. 2018;242:330–337.
- Heil A, Ohsam J, van Genugten B, et al. Microbial transglutaminase used in bread preparation at standard bakery concentrations does not increase immunodetectable amounts of deamidated gliadin. J Agric Food Chem. 2017;65(32):6982–6990.
- Lauber S, Henle T, Klostermeyer H. Relationship between the crosslinking of caseins by transglutaminase and the gel strength of yoghurt. Eur Food Res Technol. 2000;210(5):305–309.
- Ozera B, Kirmacia HA, Oztekinb S, et al. Incorporation of microbial transglutaminase into non-fat yogurt production. Int Dairy J. 2007;17(3):199–207.
- Marino M, Casale R, Borghini R, et al. The effects of modified versus unmodified wheat gluten administration in patients with celiac disease. Int Immunopharmacol. 2017;47:1–8.
- C K M, Hertel TC, Pietzsch M. Random mutagenesis of a recombinant microbial transglutaminase for the generation of thermostable and heat-sensitive variants. J Biotechnol. 2008;136(3–4):156–162.
- Melino G, Piacentini M. Tissue’ transglutaminase in cell death: a downstream or a multifunctional upstream effector? FEBS Lett. 1998;430(1–2):59–63.
- Casadio R, Polverini E, Mariani P, et al. The structural basis for the regulation of tissue transglutaminase by calcium ions. Eur J Biochem. 1999;262(3):672–679.
- Ohtsuka T, Ota M, Nio N, et al. Comparison of substrate specificities of transglutaminases using synthetic peptides as acyl donors. Biosci Biotechnol Biochem. 2000;64(12):2608–2613.
- Langston J, Blinkovsky A, Byun T, et al. Substrate specificity of Streptomyces transglutaminases. Appl Biochem Biotechnol. 2007;136(3):291–308.
- Taguchi S, Nishihama KI, Igi K, et al. Substrate specificity analysis of microbial transglutaminase using proteinaceous protease inhibitors as natural model substrates. J Biochem. 2000;128(3):415–425.
- Hopwood DA, Bibb MJ, Chater KF, et al. Genetic manipulation of Streptomyces: a laboratory manual. Norwich: The John Inns Foundation; 1985. p. 70–84.
- Wan K, Uraji M, Tokai S, et al. Enzymatic degradation of allergen peptides from bovine casein by a combination of Streptomyces aminopeptidases. Appl Biochem Biotechnol. 2018;187(2):570–582.
- Gundersen MT, Keillor JW, Pelletier JN. Microbial transglutaminase displays broad acyl-acceptor substrate specificity. Appl Microbiol Biotechnol. 2014;98(1):219–230.
- Zhu Y, Rinzema A, Tramper J, et al. Icrobial transglutaminase-a revew of its production and application in food processing. Appl Microbiol Biotechnol. 1995;44:277–282.
- Taguchi S, Arakawa K, Yokoyama K, et al. Overexpression and purification of microbial pro-transglutaminase from Streptomyces cinnamoneum and in vitro processing by Streptomyces albogriseolus proteases. J Biosci Bioeng. 2002;94(5):478–481.
- Tagami U, Shimba N, Nakamura M, et al. Substrate specificity of microbaial transglutaminase as revealed by three-dimensional docking simulation and mutagenesis. Protein Eng Des Select. 2009;22:747–752.