ABSTRACT
Two genes, aldA, and mnoA, encoding an NAD-dependent aliphatic dehydrogenase and N,N′-dimethyl-4-nitrosoaniline-dependent methanol dehydrogenase, respectively, are strongly expressed when Rhodococcus erythropolis N9T-4 is grown under oligotrophic conditions. In this study, we found a transcriptional regulator required for the transcription of both aldA and mnoA. The transcriptional regulator was also found to be essential for the oligotrophic growth of N9T-4.
We are screening natural environments for interesting bacteria named “super oligotrophs” that can grow on an inorganic minimum medium (BM medium) without the addition of any carbon and energy sources [Citation1]. Intriguingly, we observed that many super oligotrophs could be isolated easily from different environments using the BM medium, which suggested that such oligotrophs play an important role for the carbon cycle in the terrestrial ecosystem, because most of the natural environments are thought to be oligotrophic. Rhodococcus erythropolis N9T-4, isolated from crude oil in an oil stockpile in Japan, showed the best oligotrophy among the super oligotrophs that were isolated and could grow on a solid-state BM medium without any additional carbon source and also without additional nitrogen and sulfur sources [Citation2–Citation4]. Because this bacterium could not grow under CO2-limiting conditions and its growth was restored when CO2 gas was introduced, it appears that this bacterium utilizes CO2 as a carbon source for its oligotrophic growth. However, none of the genes encoding the key enzymes involved in any of the known biological CO2 fixation systems, such as RubisCO, were found in the N9T-4 genome. Although the metabolism of carbon in N9T-4 remains unknown, we believe that C2 metabolism is an important clue to understand the oligotrophy of N9T-4 because the glyoxylate shunt and gluconeogenesis are essential for the oligotrophic growth, as was revealed by random mutagenesis of the N9T-4 genome [Citation5]. Meanwhile, the oligotrophic nitrogen metabolism has been mostly elucidated in N9T-4 and this bacterium can utilize trace amounts of ammonia in the atmosphere as a nitrogen sources [Citation4]. We have also suggested that this bacterium can also utilize sulfur compounds present in the atmosphere [Citation4]
We proposed that super oligotrophs having the unique characteristics described, can provide a low-energy-input production systems for useful compounds and can also be useful for in situ bioremediation. The gene regulation for oligotrophy should be elucidated to control and apply the oligotrophic metabolism for the abovementioned purposes. Our previous transcriptomic analysis showed that the two genes, namely aldA and mnoA, encoding an aliphatic aldehyde dehydrogenase and N,N′-dimethyl-4-nitrosoaniline-dependent methanol dehydrogenase, respectively, were strongly expressed under oligotrophic conditions [Citation3,Citation6]. In this study, we identified the promoter regions of aldA and mnoA and the transcriptional regulator(s) that could be responsible for expression from these promoters. Because the aldA and the mnoA promoters are expected to be quite strong, this study could be promising for the construction of an oligotrophic expression system in Rhodococcus.
∆ligD constructed from the wild type of N9T-4, showing lower non-homologous recombination of exogenous genes [Citation5], was used as a control strain for each experiment. BM and Luria-Bertani (LB) media were used for the oligotrophic and heterotrophic cultures of N9T-4, respectively. First, reporter assay using luciferase was carried out to confirm the promoter regions upstream of aldA and mnoA. Five fragments of various lengths (1000, 800, 600, 400, and 200 bp) upstream of aldA were amplified by PCR and introduced to the upstream region of luxAB encoding a bacterial luciferase, in the pKLA1 vector [Citation7] using a Gibson assembly system (New England Biolabs Japan). Similarly, a 280-bp fragment upstream of mnoA was inserted into the pKLA1 vector. All the primers used for plasmid construction in this study are listed in Table S1. The ∆ligD strain was transformed with each of the constructed plasmids by electroporation, as described previously [Citation5]. Luciferase assay was carried out using the N9T-4 transformants grown on BM and LB plates containing kanamycin (20 μg/mL), following the method of Olsson et al. [Citation8]. A higher luminescence intensity was observed in all the transformants grown on BM plates (); however, the intensity in the transformants cultured on LB was very low, suggesting that an oligotrophy-responsive promoter exists within the 200-bp region upstream of aldA. Intriguingly, a relatively lower luminescence intensity was observed in the BM-grown cells that were transformed with plasmids having the upstream fragments of 600 bp or less regions upstream of the gene, suggesting that a transcriptional activator is involved in the region between 600- and 800-bp upstream of aldA (). We performed the same investigation for the upstream region of mnoA and observed an oligotrophy-responsive promoter within the 280-bp region upstream of mnoA (data not shown).
Figure 1. Identification of aldA promoter region.
Each transformant having each reporter plasmid was grown on BM and LB plates containing kanamycin (20 μg/mL) at 30°C for 6 and 2 days, respectively. The cells were collected by scraping from the plates, washed with 0.85% KCl, and suspended with the lux buffer (50 mN Na-phosphate, pH 7.0; 50 mM 2-mercaptoethanol; 2% bovine serum albumin) at an A600 of 10. Forty microliters of 0.1% (v/v) 1-decanal were added to 160 μL of the cell suspension and luminescence was measured immediately at 490 nm using a luminometer. Closed and open squares represent luminescence intensity of the cells grown on BM and LB, respectively. Error bars show standard deviations from biological triplicates. Statistical significance was calculated by the two-tailed t-test and one-way ANOVA. *p < 0.001; *p < 0.05; ns, not significant.
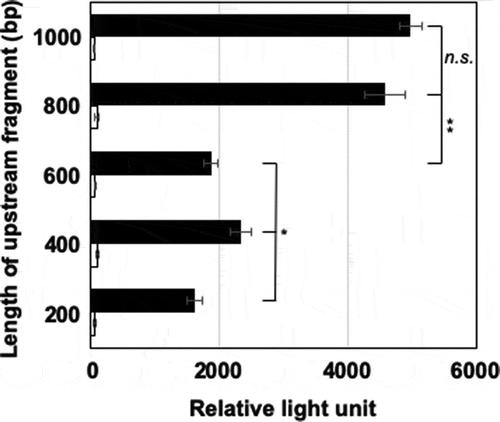
Next, based on the N9T-4 genome sequence, we tried to identify the transcriptional regulator(s) for the oligotrophy-responsive promoters of aldA and mnoA. The gene organization between aldA and mnoA are shown in . Two genes (aldR1 and aldR2) encoding possible transcriptional regulators were found in this region. A domain belonging to the AcoR super family, which is a transcriptional regulator of acetoin glycerol metabolism, is present in aldR1, and aldR2 has high homology with the mycofactocin system transcriptional regulator belonging to the TetR family. The ∆aldR1 and ∆aldR2 mutants were constructed by homologous recombination using a suicide plasmid vector, pK19sacB, as described previously [Citation5], and the oligotrophic growth of the gene-deletion mutants was evaluated using the spot test. A marked decrease in growth was observed in ΔaldR1 ()), whereas the growth of ΔaldR2 mutant was similar to that of ΔligD ()). ΔaldR1 transformed by a Rhodococus/E. coli shuttle vector, pNit-RC2 (Hokkaido System Science Co., Ltd, Japan) containing aldR1 and its upstream region showed a similar growth on BM as ΔligD (data not shown). These results suggested that aldR1 is a transcriptional regulator involved in the oligotrophic growth of N9T-4.
Figure 2. Gene organization between aldA and mnoA in R. erythropolis N9T-4.
The gene annotations (accession no. LC514289) for open reading frames in the figure are as follows: aldA, NAD-dependent aliphatic dehydrogenase; aldR1 and aldR2, transcriptional regulators examined in this study; mnoA, N,N’-dimethyl-4-nitrosoaniline-dependent methanol dehydrogenase; A, GMC family oxidoreductase; B, glycosyltransferase; C, peptidyl dipeptidase; D, HNH endonuclease; E, FadH/OYE family oxidoreductase; F, NAD-dependent oxidoreductase; G, FadH/OYE family oxidoreductase; H, heme/Flavin dehydrogenase; I, radical SAM maturase; J, mycofactocin binding protein; K, mycofactocin precursor; L, SAM methyltransferase; M, ABC permease; N, ABC ATP-binding protein; O, transcriptional regulator; P, response regulator; Q, sensor histidine kinase; R, Fe-alcohol dehydrogenase; S, ATPase. The gene products of the other open reading frames are annotated as hypothetical proteins.
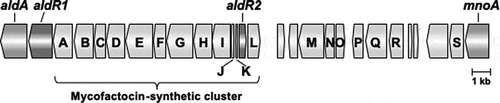
Figure 3. Oligotrophic and heterotrophic growth of ΔaldR1 (a) and ΔaldR2 (b).
Ten microliters of cell suspensions with the OD600 = 10−2-10−6 were spotted on BM and LB plates and cultivated at 30°C for 4 and 2 days, respectively. ΔligD was used as a control strain. The concentration of the spotting cell suspension was low from left to right in each photograph.
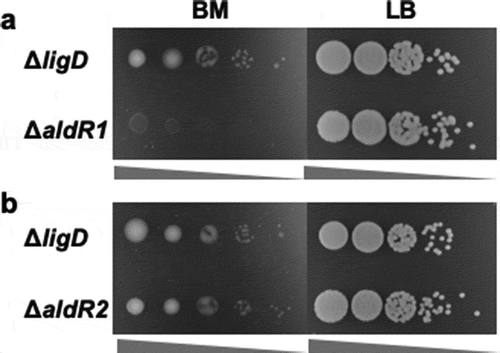
To confirm that aldR1 is a transcriptional regulator of aldA and/or mnoA, we performed luciferase assay for the aldA and mnoA promoters using the ΔligD and ΔaldR1 mutants. The pKLA1 vectors containing a 200-bp fragment upstream of aldA or a 280-bp upstream of mnoA were introduced into the ΔligD and ΔaldR1 mutants. Because ΔaldR1 did not grow on BM, the luminescence intensity of the cells grown in liquid BM medium containing 1% glucose was measured. We previously reported that glucose did not affect the gene expression of aldA and mnoA [Citation6]. The ΔligD cells showed the marked luminescence intensity, whereas the luminescence was almost absent in the ΔaldR1 cells, indicating that aldR1 is involved in the expression of both aldA and mnoA (Fig. S1).
In this study, we identified a transcriptional regulator involved in the oligotrophic growth of N9T-4. It is of interest to note that the AldR1 may regulate the expression of both aldA and mnoA, although there are no similarities between the upstream regions of these genes. Furthermore, there is no consensus sequence for the binding of transcriptional regulators in the upstream regions of aldA and mnoA. We would now examine the interaction of AldR1 with the upstream regulatory regions of these genes. As described above, AldR2 is expected to be a transcriptional regulator in the mycofactocin biosynthetic system. Recently, it was reported that mycofactocin is an electron acceptor for methanol dehydrogenase in the Gram-positive methylotroph, Mycobacterium smegmatis [Citation9]. All the genes involved in mycofactocin biosynthesis are found in the region between aldA and mnoA (), and aldR2 is located very close to the gene cluster. This suggests that the AldR2 regulates the expression of mycofactocin directly and the MnoA activity indirectly in N9T-4. The ΔaldR2 mutant did not show any phenotype in oligotrophic growth; this might be due to its nature as a negative regulator, since most of characterized TetR family regulators are repressors [Citation10]. Further investigation is needed to elucidate the relationship between mycofactocin and MnoA.
Author contribution
R.I., K.K., and N.Y. designed the experiments and discussed the results. R.I. and N.Y. prepared manuscript. R.I. and M.S. performed the experiment.
revised_FigS1_yoshida_191219.pdf
Download PDF (79.2 KB)TableS1_yoshida_191212.docx
Download MS Word (15.6 KB)Disclosure statement
No potential conflict of interest was reported by the authors.
Supplementary material
Supplemental data for this article can be accessed here.
References
- Yoshida N, Ohhata N, Yoshino Y, et al. Screening of carbon dioxide-requiring extreme oligotrophs from soil. Biosci Biotechnol Biochem. 2007;71:2830–2832.
- Yoshida N, Yagi K, Sato D, et al. Bacterial communities in petroleum oil in stockpiles. J Biosci Bioeng. 2005;99:143–149.
- Ohhata N, Yoshida N, Egami H, et al. An extremely oligotrophic bacterium, Rhodococcus erythropolis N9T-4, isolated from crude oil. J Bacteriol. 2007;189:6824–6831.
- Yoshida N, Inaba S, Takagi H. Utilization of atmospheric ammonia by an extremely oligotrophic bacterium, Rhodococcus erythropolis N9T-4. J Biosci Bioeng. 2014;117:28–32.
- Yano T, Yoshida N, Yu F, et al. The glyoxylate shunt is essential for CO2-requiring oligotrophic growth of Rhodococcus erythropolis N9T-4. Appl Microbiol Biotechnol. 2015;99:5627–5637.
- Yoshida N, Hayasaki T, Takagi H. Gene expression analysis of methylotrophic oxidoreductases involved in the oligotrophic growth of Rhodococcus erythropolis N9T-4. Biosci Biotechnol Biochem. 2011;75:123–127.
- Yamada A, Kishi H, Sugiyama K, et al. Two nearly identical aromatic compound hydrolase genes in a strong polychlorinated biphenyl degrader, Rhodococcus sp. strain RHA1. Appl Environ Microbiol. 1998;64:2006–2012.
- Olsson O, Koncz C, Szalay AA. The use of the luxA gene of the bacterial luciferase operon as a reporter gene. Mol Gen Genet. 1988;215:1–9.
- Dubey AA, Wani SR, Jain V. Methylotrophy in mycobacteria: dissection of the methanol metabolism pathway in Mycobacterium smegmatis. J Bacteriol. 2018;200:pii: e00288–18.
- Christen S, Srinivas A, Bahler P, et al. Regulation of the Dha operon of Lactococcus lactis: a deviation from the rule followed by the TetR family of transcription regulators. J Biol Chem. 2006;281:23129–23137.