ABSTRACT
For many years, clinical studies have suggested that blood levels of l-methionine and L-homocysteine correlate with health status or homocystinuria/hypermethioninemia. l-Methionine in a solution containing 0%, 10%, or 20% human serum was detected in 10–200 µM using l-methionine decarboxylase (MetDC). Spike and recovery tests showed that the enzymatic assay could accurately and reproducibly determine the increases in l-methionine in serum samples. These results suggest that our enzymatic method using MetDC is useful for primary screening of hypermethioninemia or homocystinuria based on serum l-methionine concentration. Additionally, we confirmed that l-methionine (100 nmol) in solution was degraded to less than the detection limit by incubation at 37ºC for 10 min using 2 U of MetDC. Therefore, l-homocysteine in serum samples can be detected with equivalent sensitivity using l-methionine γ-lyase (MGL), in solutions that either did not contain l-methionine or contained l-methionine preincubated with MetDC.
Abbreviations
DTT: dithiothreitol; IPTG: isopropyl-β-d-thiogalactopyranoside; KPB: potassium phosphate buffer; MBTH: 3-methyl-2-benzothiazolinonehydrazone; mdc: the gene coding l-methionine decarboxylase; MetDC: l-methionine decarboxylase; mgl: the gene coding l-methionine γ-lyase; MGL: l-methionine γ-lyase; PLP: pyridoxal 5ʹ-phosphate
Graphical Abstract
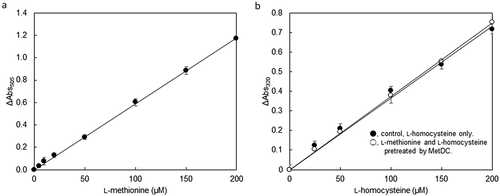
Standard curve for the L-methionine determination using MetDC (A) and L-Homocysteine determination by MGL after pretreatment of MetDC (B).
Methionine and homocysteine are amino acids, which have thiol and thiomethyl groups, respectively, indicating that these sulfur-containing amino acids are related to nitrogen and sulfur cycle in the human body. l-Methionine is an essential amino acid for humans, whereas the plasma methionine level is usually kept low (13–45 µM) and constant. l-Homocysteine is an amino acid generated as an intermediate in the l-methionine metabolism pathway, and its blood level is usually low (<15 µM) or below the detection threshold. Over many years, several enthusiastic and comprehensive clinical studies have suggested that blood levels of l-methionine and l-homocysteine correlate with health status [Citation1]. Accumulation of these sulfur-containing amino acids could be caused by a change in nutritional status or lifestyle habits, and the two congenital disorders of l-methionine metabolism – homocystinuria and hypermethioninemia [Citation2–Citation6]. Generally, patients with homocystinuria showing high l-methionine represent 50–100 µM or more than 100 µM of total homocysteine in blood [Citation2]. The concentration of l-methionine is in the range of 206–1,394 µM in patients with hypermethioninemia due to the deficiency of methionine adenosyltransferases I and III (MAT I/III) [Citation7].
The concentration of amino acid contained in blood specimens can be quantified by the tandem mass method using LC-MS [Citation8,Citation9]. HPLC and LC-MS/MS are also used for microdetermination due to high accuracy. In these chemical quantitative methods, each sample is measured via an analysis, and each analysis requires an enormous amount of time from several tens of minutes to hours. Enzymatic assays of amino acids have been studied and developed as high-throughput quantitative methods to complement the disadvantage of these chemical quantitative methods using chromatography systems [Citation10]. Therefore, it is beneficial to develop enzymatic assays that rapidly and easily detect and determine each amino acid in crude analyte from biological samples.
Our group reported an enzymatic assay for l-methionine using l-methionine γ-lyase (MGL, EC 4.4.1.11) [Citation11], and another research group reported an assay using l-methionine dehydrogenase and S-adenosyl-l-methionine (SAM) synthetase [EC 2.5.1.6] [Citation12,Citation13]. Recently we reported another l-methionine specific enzyme, l-methionine decarboxylase from Streptomyces sp. 590 (MetDC, EC 4.1.1.57) [Citation14]. PLP-dependent enzyme catalyzes the non-oxidative decarboxylation of l-methionine to produce 3-methylthiopropylamine and carbon dioxide. In this study, we constructed a novel enzymatic endpoint assay of l-methionine with colorimetric analysis using MetDC, copper amine oxidase and peroxidase ()). MGL has been used for the determination of the total amount of l-methionine and l-homocysteine in biological samples, such as blood and urine ()). Accordingly, we demonstrate that the MetDC can contribute to the fractional determination of l-methionine and l-homocysteine.
Materials and methods
Chemicals and enzymes
KOD plus neo and KOD Fx neo DNA polymerase for PCR were purchased from TOYOBO (Osaka, Japan). Restriction enzymes and TALON Metal Affinity Resin were purchased from Takara Bio Inc. (Shiga, Japan). Amicon Ultra-15 Centrifugal Filter Units for purification and concentration of enzymes were purchased from Merck Millipore (Darmstadt, Germany). PLP, l-methionine, 4-aminoantipyrine, and MBTH were provided by Nacalai Tesque (Kyoto, Japan). 3-Methylthiopropylamine and dl-homocysteine were purchased from Tokyo Chemical Industry Co., Ltd. (Tokyo, Japan) and Sigma-Aldrich (St. Louis, MO, USA), respectively. Normal human blood serum Control Serum I (Lot 1721 I), phenol, and horseradish peroxidase were provided by FUJIFILM Wako Pure Chemical Corporation (Osaka, Japan). MetDC and MGL were produced by recombinant Escherichia coli strains in our laboratory. Copper amine oxidase from Arthrobacter globiformis was prepared according to a previous report by Matsuzaki et al. [Citation15]. All other chemicals were of analytical grade.
Bacterial strains
E. coli Top10 and BL21(DE3) were purchased from Invitrogen (Kanagawa, Japan). E. coli JM109 was purchased from Novagen (Madison, WI, USA). E. coli Top10 or JM109 was used to reproduce or manipulate recombinant plasmids containing the MetDC gene (mdc) from Streptomyces sp. 590 or MGL gene (mgl) from Pseudomonas putida. E. coli BL21(DE3) and JM109 were used as host cells to heterogeneously express MetDC and MGL proteins, respectively.
Construction of plasmids
pET-28a(+) and pET-52b(+) were used for the construction of expression vectors. pET-52b-mdc coding recombinant MetDC-His-Tag10 fusion was constructed by amplifying mdc from pET-28a-mdc, as described previously [Citation14]. The following set of primers were used to amplify mdc: 5ʹ-AGGAGATATACCATGAGCCCGACCGCCTTCCCC-3ʹ (33 bp) and 5ʹ-CCGGTGATCCTGGGCGCACCCGCTCTGGTGCCACG-3ʹ (35 bp). The amplified fragment was inserted into pET-52b(+) after NcoI and SacI digestion to construct the plasmid harboring mdc (pET-52b-mdc) to express MetDC-His-Tag10 fusion. The plasmid containing mgl (pMGL1204) constructed previously [Citation11] was used to express MGL from Pseudomonas putida.
Expression and purification of MetDC and MGL
For the production of MetDC, E. coli BL21(DE3) harboring pET-52b-mdc was cultured aerobically in 50 mL of LB broth containing 100 μg/mL ampicillin at 230 rpm at 37ºC until the optical density at 600 nm reached 0.5–1.0. The culture was transferred into 200 mL of fresh modified terrific broth (TB) with 100 μg/mL ampicillin in 2% inoculation. The modified TB contained 1.2% polypeptone, 2.4% yeast extract, 1.25% K2HPO4, 0.23% KH2PO4, 2% glycerol, and 0.05% polypropylene glycol #2,000. After cultivation was conducted at 150 rpm at 37ºC until the optical density of the culture at 600 nm reached 0.4–0.6, gene expression was induced at 150 rpm at 25ºC for 15 h by the addition of IPTG at a final concentration of 1 mM. Cells were harvested by centrifugation at 6,000 × g at 4ºC for 15 min and rinsed twice with 100 mM potassium phosphate buffer (KPB, pH 7.5) containing 0.1 mM PLP.
Enzyme solutions were kept at 4ºC or on ice during purification. The cells were resuspended in buffer at a ratio of 10 times buffer volume to the weight of cells, sonicated at 150 W at 4ºC for 15 min, and centrifuged to remove cell debris. The resuspension buffer was made from 50 mM KPB (pH 7.5) containing 300 mM NaCl, 50 μM PLP, 10 mM imidazole, and 1 mM phenylmethylsulfonyl fluoride (PMSF). The supernatant was applied to an open column packed with 5 mL of TALON Metal Affinity Resin equilibrated with the resuspension buffer. Unbound proteins were eluted with 50 mM KPB (pH 7.5) containing 300 mM NaCl, 50 μM PLP, and 20 mM imidazole. His-tagged protein was eluted with 50 mM KPB (pH 7.5) containing 300 mM NaCl, 50 μM PLP, and 200 mM imidazole. The purified fractions were collected and concentrated by using Amicon Ultra-15 Centrifugal Filter Units, and the buffer was replaced with 100 mM MES (2-morpholinoethanesulfonic acid) buffer (pH 7.0) containing 0.1 mM PLP.
For the production of MGL, E. coli JM109 harboring pMGL1204 with mgl was cultured, and MGL was purified and concentrated as described previously [Citation16,Citation17]. The activities of MGL were determined by the MBTH method [Citation11,Citation18]. Protein concentration was determined by the Bradford method using a Bio-Rad Protein Assay kit with bovine serum albumin as a standard.
Enzyme assay and enzymatic L-methionine determination using MetDC
Enzyme activity of MetDC was determined by detecting hydrogen peroxide using color development method with copper amine oxidase [Citation15], phenol, 4-aminoantipyrine, and horseradish peroxidase ()). To measure the MetDC activity, 50 μL of the purified enzyme was added to 850 μL of the reaction solution containing 0.1 M KPB (pH 6.0), 40 µM PLP, and 50 mM l-methionine. After incubation at 45ºC for 5 min, the reaction mixture was heated to 80ºC for 5 min to stop the enzymatic reaction. The color-developing mixture (100 µL) containing 24 mM phenol, 150 mU/mL horseradish peroxidase, 7.5 mM 4-aminoantipyrine, and 940 mU/mL copper amine oxidase was added to the reaction mixture (900 µL) followed by incubation at 37ºC for 60 min. The absorbance was measured at 505 nm by a Shimadzu UVmini-1240 UV-VIS spectrophotometer (Shimadzu GLC Ltd., Tokyo, Japan). One unit of MetDC activity was defined as the amount of the enzyme that catalyzes the formation of 1 µmol of 3-methylthiopropylamine per minute.
Enzymatic determination of l-methionine using MetDC was carried out based on the determination of 3-methylthiopropylamine. The assay was performed in a final volume of 1.0 mL containing 100 mM KPB (pH 7.0), 40 µM PLP, 2.0 U/mL MetDC, 2.4 mM phenol, 15 mU/mL horseradish peroxidase, 750 µM 4-aminoantipyrine, 940 mU/mL copper amine oxidase, and substrate (l-methionine). Each assay cocktail (900 µL) was put into a standard disposable cuvette (semi-micro UV transparent cuvette), and then standard solutions or samples (100 µL) were added into each cuvette and incubated at 37ºC for 20 min. l-Methionine solutions (0.1, 0.2, 0.5, 1.0, 1.5, and 2.0 mM) were used as the standard solutions. The increase in absorbance at 505 nm resulting from the coupling reaction was measured by endpoint assay. The change in absorbance during reaction was monitored at 505 nm in an Eppendorf Bio Spectrophotometer kinetic (Hamburg, Germany) kept at 37ºC.
HPLC analysis of L-methionine concentrations
The concentration of l-methionine in the sample was analyzed using HPLC and FP-4020 detector (JASCO, Tokyo, Japan). Derivatization was carried out using the 4-fluoro-7-nitrobenzofurazan (NBD-F). Sample solution (150 µL) was added to 150 µL of the reaction buffer containing 100 mM potassium borate buffer (pH 8.0) and 40 mM ethylenediaminetetraacetic acid (EDTA), and 100 µL of 100 mM NBD-F solution. The mixture was heated at 60ºC for 2 min. After cooling, the reaction mixture was added to 400 μL of 40 mM HCl. The mixture was injected into an InertSustain® C18 column (3.0 µm, 3.0 × 250 mm), heated at 40ºC and eluted with Eluent A (20 mM potassium phosphate buffer, pH 2.5) and Eluent B (acetonitrile) according to the following gradient procedure: 80% Eluent A in 0 min; 80% Eluent A in 5 min; 70% Eluent A in 10 min; 50% Eluent A in 30 min; 80% Eluent A in 30.1 min; and 80% Eluent A in 45 min. The flow rate was 0.4 mL/min. Derivatized amino acids were detected at an excitation wavelength of 470 nm and an emission wavelength of 530 nm using an FP-4020 detector. l-Methionine was quantified by comparison of the external calibration curve with the standard.
Effect of blood serum on L-methionine quantification with MetDC
The reaction mixtures contained 2.0 U/mL MetDC, l-methionine (5–200 μM), 2.4 mM phenol, 750 μM 4-aminoantipyrine, 15 mU/mL peroxidase, 940 mU/mL copper amine oxidase, 10% or 20% (v/v) human blood serum Control Serum I (Lot 1721 I), and 40 μM PLP in 100 mM KPB (pH 7.0). Standard curves were constructed by assay mixtures containing no serum, and these were used to compare with the standard curves of serum-containing mixtures. The intrinsic l-methionine concentration in serum samples was calculated by subtracting the absorbance of the serum-containing reaction mixture with no l-methionine from that with l-methionine. Each measurement was repeated independently over three times, at least.
We investigated whether the process of l-methionine determination is performed in high-throughput by using a 96-well microtiter plate instead of conventional cuvettes. The reaction mixture (200 μL) contained 2.0 U/mL MetDC, l-methionine (10, 20, 50, 100, 150, 200, 300, 400, 500, or 600 µM), 2.4 mM phenol, 750 μM 4-aminoantipyrine, 15 mU/mL peroxidase, 940 mU/mL copper amine oxidase, 40 μM PLP, and 0–20% (v/v) of normal human blood serum in 100 mM KPB (pH 7.0). Absorbance of each solution in the microplate wells was measured at 492 nm using an EMax Plus Microplate Reader (Molecular Devices, CA, USA), in which the detection wavelength was preliminarily set. The light-pass length of a sample in each well in 96-well microtiter plate was calculated as about 9 mm because the amount of each sample was 200 μL, and the internal diameter of the well was 7 mm. The reaction mixtures (1 mL) in conventional cuvettes were measured at 505 nm in the Eppendorf Bio Spectrophotometer kinetic (Hamburg, Germany). Detectability of l-methionine was compared between solutions in the presence and absence of human blood serum. Every measurement was performed independently over three times, at least.
Elimination of L-methionine by MetDC
l-Methionine (100 nmol) was incubated with various units of MetDC in 1 mL of reaction mixture. The reaction mixture contained 40 μM PLP, 100 µM l-methionine, and MetDC (0, 0.50, 1.0, or 2.0 U/mL) in 100 mM sodium borate buffer (pH 8.0). After preheating at 37ºC for 5 min, 950 μL of the reaction solution was added to 50 μL of MetDC solution to start incubation at 37ºC for 10 min. After heating at 80ºC for 5 min to inactivate MetDC, the reaction mixture was added to 50 µL of MGL (0.5 U) and was incubated at 37ºC for 30 min. The enzyme solution of MGL (10 U/mL) was prepared by dilution with 100 mM KPB (pH 8.0) containing 0.01% DTT, 1 mM EDTA, 10 μM PLP, and 0.05% Tween 80. After the addition of 100 μL of 50% trichloroacetic acid for MGL inactivation, the keto acid produced from l-methionine was detected by the MBTH method. The absorbance of the solution was measured at 320 nm. The amount of l-methionine degraded by MetDC was calculated and standardized by comparison between the values of the absorbance of each solution incubated with and without MetDC. Each measurement was repeated independently over three times, at least.
Determination of L-homocysteine in mixture of L-methionine and L-homocysteine using MGL
The substrate solution contained (1) various concentrations of l-homocysteine, or (2) l-methionine and various concentrations of l-homocysteine. The final concentration of the substrate(s) in 1 mL of each substrate solution containing 40 µM PLP and 100 mM sodium borate buffer (pH 8.0) was shown as following: (1) 25, 50, 100, 150, or 200 µM l-homocysteine, or (2) 100 µM l-methionine and 25, 50, 100, 150, or 200 µM l-homocysteine.
After each substrate solution-(1) was heated at 80ºC for 5 min and incubated with MGL (0.5 U) at 37ºC for 30 min, the total amount of l-homocysteine was measured by the MBTH method. Each substrate solution-(2) was incubated at 37ºC for 10 min with MetDC in a final concentration of 2.0 U/mL. After heating at 80ºC for 5 min and incubation with 50 µL of MGL (0.5 U) at 37ºC for 30 min, the total amount of l-homocysteine and l-methionine was measured by the MBTH method. The absorbance of the solution was measured at 320 nm. The total amount of l-methionine and l-homocysteine detected by the MGL and MBTH method was determined by comparing each absorbance of the solution with and without MetDC incubation. Endpoint calibration of l-homocysteine by MGL was carried out after MetDC was thoroughly inactivated by heat; R2 values improved by eliminating the active MetDC. Each measurement was repeated independently over three times, at least.
Results
Development of new method for L-methionine determination
We developed a new method of enzyme assay using copper amine oxidase to detect MetDC activity [Citation14]. In this study, a colorimetric l-methionine assay was developed as the determining system by coupling copper amine oxidase with horseradish peroxidase, which produces a red chromogen from 4-aminoantipyrine and phenol depending on the 3-methylthiopropylamine produced from l-methionine by MetDC ()). In this assay, the produced chromogen was detected by monitoring absorbance at 505 nm. All the curves of absorbance at 505 nm reached a plateau in 20 min, due to substrate depletion ()). The enzymatic assay of l-methionine by MetDC resulted in a proportional color development giving a standard linear curve ()). This result demonstrates that an accurate concentration of l-methionine can be determined in the range of 5–200 μM by this colorimetric assay. The correlation between the enzymatic assay and HPLC analysis is shown in . The resulting plot gave a slope of nearly unity and zero intercept, and the relative error between enzymatic assay and HPLC was less than 0.5%.
Effect of blood serum on L-methionine quantification with MetDC
To verify whether the methionine assay by MetDC was appropriate for measurements in biological samples, spike and recovery tests were performed. These showed that MetDC detected 10–200 µM l-methionine, added to the solution with 10% or 20% of human serum as well as a solution without human serum ()). The standard curves represented similar slopes when the reaction solutions contained 10% or 20% of the human serum. This method for l-methionine determination was robust in the presence of human serum and could detect l-methionine rapidly and precisely in a crude solution containing 10% or 20% human serum. Blood specimen is normally diluted by several folds when the amino acid concentrations are assayed by diagnosis kit. Our l-methionine assay proved its potential utility as it was not disturbed by the addition of 10% to 20% (v/v) of human serum to the assay mixture.
Enzymatic assay for l-methionine by MetDC using a microplate reader could detect l-methionine in 10–600 µM and analyze multiple samples rapidly and simultaneously ()). The method using a microplate reader could detect l-methionine in the presence of 10% or 20% of human serum just like the method using a conventional spectrophotometer. The standard curves represented similar slopes when the reaction solutions contained 10% or 20% of human serum. A new process of l-methionine determination was performed in high-throughput by using a microtiter plate instead of conventional cuvettes.
Elimination of L-methionine by MetDC
Enzymatic assay using the MGL enzyme and MBTH method is a method to quantify the total amount of l-methionine and l-homocysteine in blood. Therefore, the amount of l-homocysteine cannot be determined separately in the presence of l-methionine. The reason is that l-methionine and l-homocysteine are degraded by MGL as good substrates and converted to α-ketobutyric acid, which is detected at 320 nm by the MBTH method ()). It was suggested that MetDC could decompose l-methionine into 3-methylthiopropylamine so that MGL could react with l-homocysteine only.
One unit of MetDC degraded 82% of l-methionine (100 nmol) at 37ºC for 10 min when 1 mL of 100 mM sodium borate buffer (pH 8.0) was used (). In addition, l-methionine (100 nmol) was degraded to less than the detection limit of the method by incubation at 37ºC for 10 min using 2.0 U of MetDC. These results suggested that l-homocysteine can be quantified using MGL after pretreatment with MetDC for decomposing l-methionine.
Determination of L-homocysteine in the mixture of L-methionine and L-homocysteine
The ability of the specific conversion and degradation of l-methionine from a mixed solution containing l-methionine and l-homocysteine was investigated. l-Homocysteine in each concentration was detected with equivalent intensities in either cases when the solution either did not contain l-methionine or contained l-methionine incubated with MetDC (). The range of detection of l-homocysteine was from 25 to 200 µM.
MetDC was able to degrade l-methionine to 3-methylthiopropylamine and carbon dioxide and eliminate the amino acid from the detection system using the MGL enzyme and MBTH methods. We report that MetDC degrades l-methionine to less than the detection limit, and that MGL determined l-homocysteine precisely from a mixture solution of l-homocysteine and l-methionine.
Discussion
In newborn screenings, blood specimens are quantified by the tandem mass method using LC-MS [Citation8,Citation9]. HPLC and LC-MS/MS are also used for microdetermination due to a high degree of accuracy. In these chemical quantitative methods based on chromatography, each sample is measured via an analysis, and each analysis requires an enormous amount of time from several tens of minutes to hours. In the primary neonatal screening, it is necessary to analyze many samples in a short time and at the same time with a high-throughput performance. Simple and rapid measurement of l-methionine and l-homocysteine in blood or urine has epidemiological and medical significance as the primary screening method for hypermethioninemia and homocystinuria. By implementing the 4-aminoantipyrine method with MetDC and the MBTH method with MGL, we determined the concentration of l-methionine and l-homocysteine, respectively (). A combination of MetDC and MGL was found to be a beneficial method for fractional determination of l-methionine and l-homocysteine in solution and human serum, with high-throughput performance. Also, our colorimetric assay system using MetDC was applicable for the determination of l-methionine with both a spectrophotometer and a microplate reader. The elimination of l-methionine by MetDC pretreatment played an important role in determining l-homocysteine by MGL from the mixture.
In addition to the l-methionine assay method we reported in this paper, several l-methionine assay methods using coupling enzymes such as MGL, mutant of l-methionine dehydrogenase (mutated l-phenylalanine dehydrogenase), and SAM synthetase have been reported. First, MGL enzymatic assay with the MBTH method detects α-keto acid. The MGL method with MBTH [Citation18] quantified l-methionine more selectively and easily. However, MGL enzyme degraded l-homocysteine as well as l-methionine and did not distinguish each sulfur-containing amino acid. Second, l-methionine dehydrogenase was developed through multiple mutations of l-phenylalanine dehydrogenase and showed high selectivity for branched-chained amino acids in human plasma as well as l-methionine [Citation12]. That required a pretreatment to remove the branched-chain amino acids from the analytes. Third, the SAM synthetase method was developed as a methionine-selective assay that prevents the inhibition by ammonium and the interference by branched-chained amino acids [Citation13]. The enzyme converted l-methionine and ATP to SAM, inorganic phosphate (Pi), and pyrophosphate (PPi). A PPi detection system was constructed by coupling SAM synthetase to recombinant pyruvate phosphate dikinase (PPDK; EC 2.7.9.1) from Propionibacterium freudenreichii NBRC 12426. Three types of detection methods, including a colorimetric assay system, were adopted for SAM synthetase assays as well as PPDK assays. High specificity toward l-methionine was expected because SAM synthetase reacts with its l-methionine at its thioether group. However, the methods of enzyme assay contained three steps of reaction, at least from l-methionine to each compound that can be detected.
In MetDC assay, the pretreatment to remove branched-chained amino acids was not necessary because the substrate specificity for l-methionine was relatively high [Citation14], as in SAM synthetase assay. MetDC is also expected to have high selectivity at its thioether group because the substrate specificity toward l-methionine was higher than that toward non-protein amino acid l-norleucine. The sulfur atom in the former compound is replaced with a carbon atom in the latter compound [Citation14].
The assay of 4-aminoantipyrine with MetDC contained only two steps of reaction from l-methionine to each compound that can be detected, while SAM synthetase assays contains three or four steps. Since the MetDC assay was composed of inexpensive reagents, as compared with the conventional enzymatic determination method of l-methionine, the running cost could be kept low.
To determine l-homocysteine from the mixture of l-methionine and l-homocysteine, we applied a tandem approach using MetDC and MGL. The interference of l-methionine with enzymatic assay using MGL was eliminated selectively by the enzymatic degradation of l-methionine using MetDC. This result suggested that MetDC was suitable for the degradation of l-methionine for the determination of l-homocysteine in a mixture of l-methionine and l-homocysteine using MGL.
Also, several l-homocysteine assay methods have been reported so far. For example, an enzymatic assay of l-homocysteine using homocysteine methyltransferase is commercialized as an assay kit [Citation19]. Although the method requires the presence of d-amino acid in each sample, the concentration of d-amino acids in the biologic samples could slightly increase in some diseases. Therefore, standard solutions of the corresponding d-amino acids are required as controls for each sample. For the l-homocysteine assay method, we report in this paper, pretreatment to degrade l-amino acids other than methionine was not necessary, because the substrate specificity of MGL for l-methionine and l-homocysteine was relatively high. The pretreatment to degrade l-methionine using MetDC could make the method of l-homocysteine determination by MGL available for the diagnosis of homocystinuria. Detectability of our l-homocysteine determination method is expected to be improved by the fluorescence method combined with MGL.
Our MetDC assay using a microplate reader was shown to determine l-methionine added in human serum in a wide range of concentrations, ranging from 10 to 600 µM ()). Microplate reader provided the advantage of treating a number of samples in a short time period, for example, taking up to an hour, including sample preparation time. MetDC assay may be used as a primary screening method for hypermethioninemia and/or homocystinuria. If concentrations of l-methionine were indicated by the enzyme assay to be far beyond or much lower than the normal levels, HPLC analysis could be employed to confirm the abnormality.
Furthermore, the tandem approach using MetDC and MGL are expected to be used as a selective l-homocysteine assay like the enzymatic assay for l-methionine using MetDC and copper amine oxidase shown in this study.
To conclude, we developed a novel method of l-methionine determination using MetDC and MetDC/MGL combination method for l-homocysteine determination. Our findings revealed the following: (1) MetDC could determine l-methionine concentration in a sample even though it was mixed with human serum. (2) MetDC was able to specifically degrade l-methionine (decompose to 3-methylthiopropylamine and carbon dioxide) from a mixture of l-methionine and l-homocysteine. Our study will contribute to the determination of these two sulfur-containing amino acids from biological samples such as human blood serum, which will help diagnose homocystinuria or hypermethioninemia in an early stage or help with easy health status monitoring in daily life.
Author contribution
Atsushi Okawa and Masaya Hayashi carried out the experiments. Toshihide Okajima, Takashi Tamura, and Junko Inagaki contributed analysis and discussion. Atsushi Okawa, Masaya Hayashi, and Kenji Inagaki prepared the manuscript.
Ethical approval
This article does not contain any studies with human participants or animals performed by any authors.
Disclosure statement
No potential conflict of interest was reported by the authors.
Data availability statement
The data described in this article are openly available in the Open Science Framework at DOI:10.17605/OSF.IO/TPA6U.
References
- Kumar A, Palfrey HA, Pathak R, et al. The metabolism and significance of homocysteine in nutrition and health. Nutr Metab. 2017;14:78.
- Homocystinuria Caused by Cystathionine Beta-Synthase Deficiency. GeneReviews® [Internet]; 2017 [cited 2018 Dec 18]. Available from: https://www.ncbi.nlm.nih.gov/books/NBK1524/.
- Jacques PF, Bostom AG, Williams RR, et al. Relation between folate status, a common mutation in methylenetetrahydrofolate reductase, and plasma homocysteine concentrations. Circulation. 1996;93:7–9.
- Perry IJ, Refsum H, Morris RW, et al. Prospective study of serum total homocysteine concentration and risk of stroke in middle-aged British men. Lancet. 1995;346:1395–1398.
- Christen WG, Ajani UA, Glynn RJ, et al. Blood levels of homocysteine and increased risks of cardiovascular disease: causal or casual? Arch Intern Med. 2000;160:422–434.
- Homocysteine Studies Collaboration. Homocysteine and risk of ischemic heart disease and stroke: a meta-analysis. J Am Med Assoc. 2002;288:2015–2022.
- Stabler SP, Steegborn C, Wahl MC, et al. Elevated plasma total homocysteine in severe methionine adenosyltransferase I/III deficiency. Metab Clin Exp. 2002;51:981–988.
- Refsum H, Grindflek AW, Ueland PM, et al. Screening for serum total homocysteine in newborn children. Clin Chem. 2004;50:1769–1784.
- Chace DH, Kalas TA, Naylor EW. Use of tandem mass spectrometry for multianalyte screening of dried blood specimens from newborns. Clin Chem. 2003;49:1797–1817.
- Wibrand F. A microplate-based enzymatic assay for the simultaneous determination of phenylalanine and tyrosine in serum. Clin Chim Acta. 2004;347:89–96.
- Takakura T, Mitsushima K, Yagi S, et al. Assay method for antitumor l-methionine γ-lyase: comprehensive kinetic analysis of the complex reaction with l-methionine. Anal Biochem. 2004;327:233–240.
- Yamasaki-Yashiki S, Tachibana S, Asano Y. Determination of l-methionine using methionine-specific dehydrogenase for diagnosis of homocystinuria due to cystathionine β-synthase deficiency. Anal Biochem. 2012;428:143–149.
- Kameya M, Himi M, Asano Y. Rapid and selective enzymatic assay for l-methionine based on a pyrophosphate detection system. Anal Biochem. 2014;447:33–38.
- Hayashi M, Okada A, Yamamoto K, et al. Gene cloning, recombinant expression, purification and characterization of l-methionine decarboxylase from Streptomyces sp. 590. J Biochem. 2017;161:389–398.
- Matsuzaki R, Fukui T, Sato H, et al. Generation of the topa quinone cofactor in bacterial monoamine oxidase by cupric ion-dependent autooxidation of a specific tyrosyl residue. FEBS Lett. 1994;351:360–364.
- Kudou D, Misaki S, Yamashita M, et al. Structure of the antitumour enzyme l-methionine γ-lyase from Pseudomonas putida at 1.8Å resolution. J Biochem. 2007;141:535–544.
- Kudou D, Misaki S, Yamashita M, et al. The role of cystein 116 in the active site of the antitumor enzyme l-methionine γ-lyase from Pseudomonas putida. Biosci Biotechnol Biochem. 2008;72:1722–1730.
- Soda K. Microdetermination of d-amino acids and d-amino acid oxidase activity with 3-methyl-2-benzothiazolone hydrazone hydrochloride. Anal Biochem. 1968;25:228–235.
- Matsuyama N, Yamaguchi M, Toyosato M, et al. New enzymatic colorimetric assay for total homocysteine. Clin Chem. 2001;47:2155–2157.