ABSTRACT
Chitosan (CHT) induces stomatal closure and thus plays a crucial role in plants to adapt to the adverse environments. Our previous results of a SA-deficient mutant nahG suggest that endogenous salicylic acid (SA) is involved in the CHT signaling in guard cells. Here in order to make the involvement definite, we examined stomatal responses to CHT of another SA-deficient mutant, sid2, and an SA receptor mutant, npr1-3. The sid2 mutation impaired CHT-induced stomatal closure and reactive oxygen species production and both impairments were complemented with exogenous SA application. Moreover, the CHT-induced stomatal closure is disrupted in the npr1-3 mutant. These results suggest that endogenous SA is involved in the CHT-induced stomatal closure via the SA receptor, NPR1.
Abbreviations
SA: salicylic acid; ABA: abscisic acid; ROS: reactive oxygen species; NPR1: nonexpresser of pathogenesis-related genes1; CHT: chitosan; DAB: 3,3′-diaminobenzidine
Graphical abstract
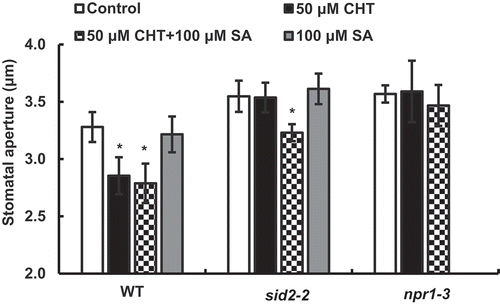
Impairment of chitosan-induced stomatal closure in SA-deficient mutant, sid2, and an SA receptor mutant, npr1, and the complementation of the impairment with exogenous SA application.
The plant leaves have stomatal apertures which are formed by guard cells. Guard cells in response to a variety of biotic and abiotic stimuli (e.g., elicitors and plant hormones) regulate the stomatal apertures. The stomatal closure prevents entry of pathogens into the plants via stomatal pores [Citation1] and reduces loss of water through transpiration [Citation2].
Elicitors mimic microbial attack and results in physiological changes in plants [Citation3]. Chitosan (CHT) is an elicitor that is a deacylated derivative of chitin and present mainly in the cell wall of fungi [Citation4]. Chitosan induces stomatal closure in plants [Citation5–Citation7]. In comparison with hormone signaling (e.g., abscisic acid (ABA) signaling and salicylic acid (SA) signaling) in guard cells, less information is available on the molecular mechanism of CHT signaling in guard cells. It has recently been reported that endogenous SA is involved in the CHT-induced stomatal closure [Citation8]. However, it remains to be clarified how endogenous SA primes CHT-induced stomatal closure.
Nonexpresser of pathogenesis-related genes1 (NPR1) is a well-known SA receptor [Citation9], which is essential for the SA-induced stomatal closure as well as the stomatal closure in response to coronatine-deficient Pseudomonas syringae pv tomato bacteria [Citation10,Citation11]. Hence, it is expected that SA receptor play role in the CHT signaling in guard cells.
Reactive oxygen species (ROS) act as second messengers in both hormone signaling and elicitor signaling in guard cells. Production of ROS is mediated by plasma membrane NAD(P)H oxidases in both ABA signaling and methyl jasmonate signaling and by salicylhydroxamic acid-sensitive peroxidases in both SA signaling and CHT signaling and is followed by activation of plasma membrane Ca2+-permeable nonselective cation channels and consequent cytosolic Ca2+ elevation [Citation6,Citation7,Citation12–Citation15]. The generation of ROS is crucial for the signal integration in guard cells [Citation16–Citation19]. Chitosan-induced stomatal closure is accompanied by the ROS production that is mediated by salicylhydroxamic acid-sensitive peroxidases [Citation5–Citation7].
To elucidate the regulation of CHT signaling by endogenous SA in guard cells, we analyzed CHT-induced stomatal closure and apoplastic ROS production in the SA-deficient mutant, sid2-2, and also analyzed CHT-induced stomatal closure in the SA receptor mutant, npr1-3.
Materials and methods
Plants and their growth conditions
Arabidopsis ecotype Columbia-0 (Col-0) was used as a wild type (WT). The sid2-2 [Citation20] and npr1-3 mutants were grown in controlled growth conditions. The growth conditions were soil:vermiculite (v/v) 1:1 mixture under a 16-h-light/8-h-dark photoperiod with photon flux density of 80 µmolm−2s−1. The temperature was 21°C and relative humidity was 70% in the growth chamber. Hyponex (0.1%) nutrient solution was provided to the plants twice per week. Four-week-old plants were used for experiments.
Analysis of stomatal apertures
Stomatal apertures were measured according to the method of Munemasa et al. [Citation21]. Rosette leaves were floated on a buffer solution containing 5 mM KCl, 50 µM CaCl2, and 10 mM MES (pH 6.15) for 2 h in the light for stomatal opening. Then CHT (50 µg/mL) and SA (50 µM and 100 µM) were added to the buffer solution followed by 2 h incubation in the light. The irradiated leaves were then blended in a commercial blender for 28 s and the epidermal tissues were collected using a 100 µm nylon mesh. The collected tissues were mounted on slides for microscopic observation. The widths of 20 stomatal apertures were measured for each experiment and the experiment under the same condition was repeated at least three times.
Measurement of apoplastic ROS production
Apoplastic ROS production was measured using 3,3′-diaminobenzidine (DAB) [Citation22]. Fully expanded leaves were floated on an infiltration buffer (5 mM KCl, 50 µM CaCl2, 10 mM MES, and 0.1% Tween 20 (pH 6.15) in the light for 3 h. Then 1 mg/mL DAB was added to the buffer. Vacuum infiltration was imposed on the DAB-treated leaves for 2 h. Chitosan was then added to the above buffer solution with or without SA and again incubated for 2 h. The leaves were depigmented in boiled ethanol. Hydrogen peroxide (H2O2) was visualized as reddish-brown color and quantified using Image-J software (National Institutes of Health, USA).
Statistical analysis
The significance of differences of mean values of stomatal apertures and ROS production were assessed by the t-test. Differences were considered significant at p < 0.05.
Results
Chitosan induced stomatal closure in the sid2-2 in the presence of SA but not in the absence of SA
Chitosan at 50 µg/mL significantly induced stomatal closure in the WT ()), which is in agreement with the previous results [Citation6–Citation8,Citation23], whereas 50 µg/mL CHT did not induce stomatal closure in the sid2-2 ()). In the presence of 100 µM SA, 50 µg/mL CHT significantly induced stomatal closure in the sid2-2 ()). These results suggest that endogenous SA is involved in CHT-induced stomatal closure. However, 50 µg/mL CHT did not significantly induce stomatal closure in the sid2-2 in the presence of 50 µM SA ()). Note that SA at 50 µM and 100 µM did not induce stomatal closure in either WT or in the sid2-2 ()).
Figure 1. Chitosan (CHT)-induced responses of stomatal apertures in Arabidopsis wild type (WT) and sid2-2 mutant. (a) CHT (50 µg/mL) induced stomatal closure in WT but not in the sid2-2 plants. (b) Stomatal closure induced by co-treatment with CHT (50 µg/mL) and SA (100 µM) in WT and the sid2-2 plants. (c) SA at 50 µM and 100 µM did not induce stomatal closure in WT and the sid2-2 plants. Each bar shows average of stomatal apertures of 80 stomata (n = 4, where each experiment is from 20 stomata data). Error bars represent SE. * indicates significant at p < 0.05.
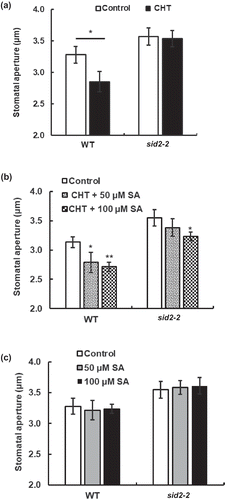
Chitosan elicited apoplastic ROS production in the sid2-2 in the presence of SA but not in the absence of SA
To test whether endogenous SA is required for CHT signaling in guard cells, we examined the effects of exogenous SA on apoplastic ROS production in the sid2-2 mutants. Histochemical staining using DAB (1 mg/mL) revealed that CHT did not induce apoplastic ROS production in the sid2-2 mutant in the absence of SA ()) but induced apoplastic ROS production in the presence of 100 µM SA ()). These results suggest that endogenous SA is required for the CHT-elicited apoplastic ROS production. Note that SA at 100 µM did not induce apoplastic ROS production either in WT or in the sid2-2 ()). These results are consistent with the stomatal assay data shown in .
Figure 2. Chitosan (CHT)-elicited apoplastic ROS production in wild type (WT) and sid2-2 mutant. (a), CHT (50 µg/mL) induced apoplastic ROS production in WT but not in the sid2-2 mutant. (b) Apoplastic ROS production induced by co-treatment with CHT (50 µg/mL) and SA (100 µM) in WT and the sid2-2 plants. (c) SA at 100 µM did not induce apoplastic ROS production both in WT and the sid2-2 plants. Each bar shows averages of ROS production from 16 leaves (n = 4). The vertical scale shows the relative values of pixel intensity of the DAB brown color of the H2O2 formation when the values of leaves treated with CHT, SA, and both CHT and SA are normalized to control value taken as 100 for each experiment. Error bars stand for SE. * indicates significant at p < 0.05.
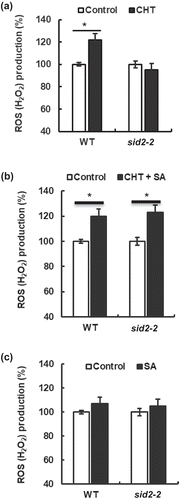
Chitosan-induced stomatal closure is impaired in the NPR1-disruption mutant npr1-3
To investigate whether the NPR1 receptor is involved in CHT signaling in guard cells, we examined stomatal response to CHT in the npr1-3 mutant. The npr1-3 mutation completely inhibited CHT-induced stomatal closure ()), suggesting NPR1 is involved in CHT-induced stomatal closure. Moreover, co-treatment with CHT (50 µg/mL) and SA (100 µM) did not induce stomatal closure in the npr1-3 mutant ()).
Figure 3. Impairment of chitosan (CHT)-induced stomatal closure in NPR1-disruption mutant npr1-3. (a) CHT at 50 µg/mL induced stomatal closure in WT, but not in the npr1-3 in the absence of SA. (b) 50 µg/mL CHT in the presence of 100 µM SA did not induce stomatal closure in the npr1-3 mutant. Each bar represents averages of stomatal apertures from at least total 60 stomata. Stomatal apertures from three independent experiments (n = 3) are shown. Error bars stand for SE. * indicates significant at p < 0.05.
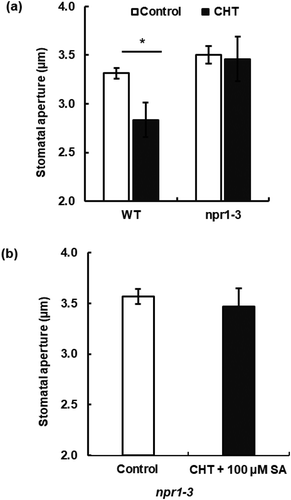
Figure 4. A scheme of interaction between chitosan (CHT) signaling and salicylic acid (SA) signaling in guard cells. (a) Salicylic acid (SA)-deficient condition; the chitosan signal pathway is not activated by SA. Consequently, chitosan cannot induce stomatal closure. (b) Lower SA condition; the chitosan signal pathway is activated by SA, which is mediated by NPR1. As a consequence, chitosan induces ROS production, resulting in stomatal closure. (c) Higher SA condition; SA activates both the SA signal pathway and the chitosan signal pathway. As a result, SA induces stomatal closure.
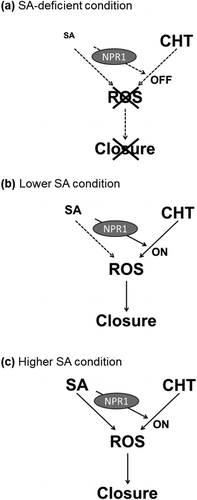
Discussion
Chitosan induces stomatal closure, which plays a vital role in plants under the adverse conditions. Significant efforts have been devoted to elucidate hormone signaling (e.g., ABA and SA) in guard cells. In comparison, very few studies have focused on elicitor signaling such as CHT signaling in guard cells [Citation5–Citation7]. Based on the results of stomatal response to CHT of nahG, it has recently been reported that endogenous SA is required for CHT signaling in guard cells [Citation8], where catechol, to which nahG catalyzes the degradation from SA, did not affect stomatal apertures [Citation8,Citation24]. However, the molecular mechanism remains unclear. Here, we confirmed the involvement of SA in CHT-induced stomatal closure and CHT-elicited apoplastic ROS production in guard cells using the SA biosynthetic pathway-disruption mutant sid2-2 as another SA-deficient mutant and revealed the involvement of the SA receptor NPR1 in CHT-induced stomatal closure.
The impairment of CHT-induced stomatal closure and the dysfunction of CHT-induced apoplastic ROS production in the SA-deficient mutant sid2-2 were complemented by exogenous SA ( and ). These results indicate that endogenous SA is involved in the CHT-induced stomatal closure and CHT-elicited apoplastic ROS production. These results are in agreement with the previous results [Citation8]. Both nahG and sid2-2 results suggest that the SA priming is required for the CHT signaling in Arabidopsis guard cells.
Previous reports suggested that priming by endogenous ABA is required for the methyl jasmonate-induced stomatal closure [Citation25] but not for the CHT-, yeast elicitor-, or SA-induced stomatal closure in Arabidopsis [Citation23]. Likewise, priming by endogenous jasmonic acid is not necessary for the CHT-, yeast elicitor-, or SA-induced stomatal closure [Citation23]. Our previous study using the nahG plants has revealed that endogenous SA priming is required for CHT signaling in guard cells [Citation8], which is supported by this study using the sid2-2. Taken together, CHT signaling is strongly dependent on endogenous SA but not on endogenous ABA or jasmonates.
The SA receptor, NPR1, is a key regulatory component that is located at the junction of multiple defense signaling pathways. The npr1-1 plants do not respond to SA or to its analogue benzothiadizole [Citation26] in terms of defense or plant growth [Citation27]. Arabidopsis NPR1 is required for SA-induced PR gene expression and resistance against pathogens [Citation28,Citation29]. The previous reports have shown that NPR1 plays a role in guard cell SA signaling [Citation10,Citation11]. In this study, the complete impairment of CHT-induced stomatal closure in the npr1-3 mutant indicates that NPR1 is necessary for the CHT-induced stomatal closure (). NPR1 has five paralogs in Arabidopsis such as NPR2, NPR3, NPR4, BLADE ON PETIOLE 1 (BOP1), and BOP2. The NPR2 [Citation30], NPR3 and NPR4 act as SA receptors [Citation9,Citation31]. Fu et. al [Citation31]. and Wu et. al [Citation9]. have published conflicting models for how SA regulates the activity of NPR1.
Fu et al. [Citation31] have proposed a complex model, where SA binds NPR3 and NPR4 rather than NPR1. These receptors have different binding affinities to SA. NPR3 is suggested as a low-affinity SA receptor and NPR4 is as a high-affinity one [Citation31]. These receptors functioned as E3 ubiquitin ligases to mediate ubiquitination of NPR1 and its subsequent degradation [Citation31]. In contrast, Wu et al. [Citation9] reported that NPR1 bound SA with high affinity. The regulation of NPR1 activity is not mediated by protein degradation and there is no requirement for the NPR3 and NPR4 proteins to achieve full activation of defense gene expression [Citation9].
Like NPR1, NPR2 acts as an SA receptor [Citation30]. NPR2 is the only paralogue able to partially complement an npr1-1 mutation but the other NPRs did not [Citation30]. In the presence of NPR1, NPR3 and NPR4 act as competitors to NPR1 [Citation30]. However, NPR3 and NPR4 do not as strongly contribute to SA signaling as NPR1 or NPR2 although they perceive SA.
In conclusion, NPR1 is involved in SA-dependent CHT signaling in Arabidopsis guard cells (). Although NPR2, NPR3, and NPR4 perceive SA, they are less effective in producing signals in guard cells. NPR1 mutation impaired the CHT-induced stomatal closure suggesting NPR1 and NPR2 are not function redundantly in the CHT signaling in guard cells. This result supports the critical role of NPR1 in the CHT signaling in guard cells. Therefore, NPR1 is the master regulator of SA-dependent CHT signaling in guard cells.
Author contributions
M. Y. P. and M.I. performed the experiments. S. M. and Y. N. assisted with the experiments and contributed to discussions. M. Y. P. and Y. M. wrote the manuscript.
Data availability statement
The data described in this article are openly available in the Open Science Framework at DOI:10.17605/OSF.IO/TPA6U.
Disclosure statement
No potential conflict of interest was reported by the authors.
References
- Arnaud D, Hwang I. A sophisticated network of signaling pathways regulates stomatal defenses to bacterial pathogens. Mol Plant. 2015;8:566–581.
- Lawson T, Blatt MR. Stomatal size, speed, and responsiveness impact on photosynthesis and water use efficiency. Plant Physiol. 2014;164:1556–1570.
- Zhao J, Davis LC, Verpoorte R. Elicitor signal transduction leading to production of plant secondary metabolites. Biotechnol Adv. 2005;23:283–333.
- Bartnicki-Garcia S. Cell wall composition and other biochemical markers in fungal phylogeny. In: Harborne JB, editor. Phytochemical Phylogeny. London: Academic Press; 1970. p. 81–101.
- Lee S, Choi H, Suh S, et al. Oligogalacturonic acid and chitosan reduce stomatal aperture by inducing the evolution of reactive oxygen species from guard cells of tomato and Commelina communis. Plant Physiol. 1999;121:147–152.
- Khokon MAR, Uraji M, Munemasa S, et al. Chitosan-induced stomatal closure accompanied by peroxidase-mediated reactive oxygen species production in Arabidopsis. Biosci Biotechnol Biochem. 2010;74:2313–2315.
- Salam MA, Jammes F, Hossain MA, et al. MAP kinases, MPK9 and MPK12, regulate chitosan-induced stomatal closure. Biosci Biotechnol Biochem. 2012;76:1785–1787.
- Prodhan MY, Issak M, Nakamura T, et al. Chitosan signaling in guard cells requires endogenous salicylic acid. Biosci Biotechnol Biochem. 2017;81:1536–1541.
- Wu Y, Zhang D, Chu Jee Y, et al. The arabidopsis NPR1 protein is a receptor for the plant defense hormone salicylic acid. Cell Rep. 2012;1:639–647.
- Zeng W, He SY. A prominent role of the flagellin receptor FLAGELLIN-SENSING2 in mediating stomatal response to Pseudomonas syringae pv tomato DC3000 in Arabidopsis. Plant Physiol. 2010;153:1188–1198.
- Zeng W, Brutus A, Kremer JM, et al. A genetic screen reveals Arabidopsis stomatal and/or apoplastic defenses against Pseudomonas syringae pv tomato DC3000. PLoS Pathog. 2011;7:e1002291.
- Pei ZM, Murata Y, Benning G, et al. Calcium channels activated by hydrogen peroxide mediate abscisic acid signaling in guard cells. Nature. 2000;406:731–734.
- Klüsener B, Young JJ, Murata Y, et al. Convergence of calcium signaling pathways of pathogenic elicitors and abscisic acid in Arabidopsis guard cells. Plant Physiol. 2002;130:2152–2163.
- Kwak JM, Mori IC, Pei ZM, et al. NADPH oxidase Atrboh D and Atrboh F genes function in ROS-dependent ABA signaling in Arabidopsis. Embo J. 2003;22:2623–2633.
- Ye W, Muroyama D, Munemasa S, et al. Calcium-dependent protein kinase CPK6 positively functions in induction by yeast elicitor of stomatal closure and inhibition by yeast elicitor of light-induced stomatal opening in Arabidopsis. Plant Physiol. 2013;163:591–599.
- Mori IC, Murata Y, Uraji M. Integration of ROS and hormone signaling. In: Del Río LA, Puppo A, editors. Reactive oxygen species in plant signaling, signaling and communication in plants. Berlin Heidelberg: Springer-Verlag; 2009. p. 25–42.
- Song Y, Miao Y, Song C-P-P. Behind the scenes: the roles of reactive oxygen species in guard cells. New Phytol. 2014;201:1121–1140.
- Murata Y, Mori IC, Munemasa S. Diverse stomatal signaling and the signal integration mechanism. Annu Rev Plant Biol. 2015;66:369–392.
- Prodhan MY, Munemasa S, Nahar MNN, et al. Guard cell salicylic acid signaling is integrated into abscisic acid signaling via the Ca2+/CPK-dependent pathway. Plant Physiol. 2018;178:441–450.
- Wildermuth MC, Dewdney J, Wu G, et al. Isochorismate synthase is required to synthesize salicylic acid for plant defence. Nature. 2001;414:562–565.
- Munemasa S, Oda K, Watanabe-Sugimoto M, et al. The coronatine-insensitive1 mutation reveals the hormonal signaling interaction between abscisic acid and methyl jasmonate in Arabidopsis guard cells: specific impairment of ion channel activation and second messenger production. Plant Physiol. 2007;143:1398–1407.
- Thordal-Christensen H, Zhang Z, Wei Y, et al. Subcellular localization of H2O2 in plants. H2O2 accumulation in papillae and hypersensitive response during the barley-powder mildew interaction. Plant J. 1997;11:1187–1194.
- Issak M, Okuma E, Munemasa S, et al. Neither endogenous abscisic acid nor endogenous jasmonate is involved in salicylic acid-, yeast elicitor-, or chitosan-induced stomatal closure in Arabidopsis thaliana. Biosci Biotechnol Biochem. 2013;77:1111–1113.
- Yamamoto S, katagiri M, Maeno H, et al. Salicylate hydroxylase, a monooxygenase requiring flavin adenine dinucleotide. J Biol Chem. 1965;240:3408–3413.
- Hossain MA, Munemasa S, Uraji M, et al. Involvement of endogenous abscisic acid in methyl jasmonate-induced stomatal closure in Arabidopsis. Plant Physiol. 2011;156:430–438.
- Lawton KA, Friedrich L, Hunt M, et al. Benzothiadiazole induces disease resistance in Arabidopsis by activation of the systemic acquired resistance signal transduction pathway. Plant J. 1996;10:71–82.
- Canet JV, Dobo´n A, Iba´ñez F, et al. Resistance and biomass in Arabidopsis: a new model for salicylic acid perception. Plant Biotech J. 2010;8:126–141.
- Cao H, Bowling SA, Gordon AS, et al. Characterization of an Arabidopsis mutant that is nonresponsive to inducers of systemic acquired resistance. Plant Cell. 1994;6:1583–1592.
- Delaney TP, Friedrich L, Ryals JA. Arabidopsis signal transduction mutant defective in chemically and biologically induced disease resistance. Proc Natl Acad Sci. 1995;92:6602–6606.
- Castelló MJ, Medina-Puche L, Lamilla J, et al. NPR1 paralogs of Arabidopsis and their role in salicylic acid perception. Plos One. 2018;13:e0209835.
- Fu ZQ, Yan S, Saleh A, et al. NPR3 and NPR4 are receptors for the immune signal salicylic acid in plants. Nature. 2012;486:228–232.