ABSTRACT
In this study, we investigated the effects of dietary supplementation of Citrus tumida hort. ex Tanaka on food intake, body and fat tissue weights, and metabolic profiles of plasma and liver in mice. Supplementation with 5% (w/w) of peels of immature C. tumida (PIC) for 4 weeks significantly suppressed body weight gain and decreased adipose tissue weight in epididymal, perirenal, and subcutaneous fats. Metabolome analyses showed that 2-hydroxyvaleric acid levels were reduced in the blood plasma of mice fed with PIC. PIC supplementation significantly elevated dipeptide (Thr-Asp, Ser-Glu, and Ala-Ala), glucuronic acid, and S-methylglutathione levels, and significantly reduced betaine aldehyde levels in the liver. In conclusion, PIC supplementation affects the metabolism of fatty acids, pectin, glutathione, and choline, showing potential beneficial effects for metabolic syndrome and obesity. PIC may be developed as a functional food and used in the treatment of these diseases.
Graphical Abstract
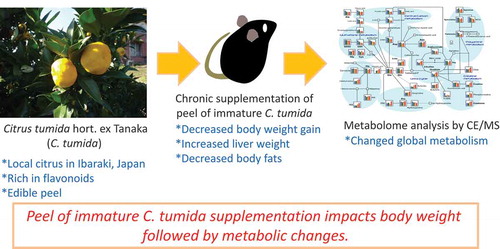
Peel of immature C. tumida supplementation impacts body weight followed by metabolic changes.
Preventive and alternative medicines have shown to improve the quality of life, especially in the elderly population. Good health conditions may be attained by life habits, such as regular exercise, good sleep, and a proper diet. The effects of food on the quality of life have been extensively studied, and various functional foods and supplements are recommended to preserve or improve health. Fruits contain potent ingredients that affect our health. Citrus fruits are popular in several countries and have various health benefits [Citation1]. The dried peels of Citrus unshiu and C. reticulata, which are used as natural medicines in Japan and China, show beneficial effects, including improved brain function [Citation2]. The peel extract of C. depressa helps prevent obesity in mice fed with a high-fat diet [Citation3]. Moreover, supplements of C. unshiu peel extract have been found to restore adenocarcinoma-induced weight loss in mice [Citation4]. Citrus peels contain high amounts of flavonoids, which contribute to the health-beneficial effects [Citation2]. Hesperidin and nobiletin flavonoids found in several citrus fruits show beneficial effects against some features of metabolic syndrome [Citation1]. Interestingly, nobiletin shows a protective effect against metabolic syndrome by enhancing circadian rhythms [Citation5], and oral administration of hesperidin reduces the levels of inflammatory markers in patients with metabolic syndrome [Citation6]. In addition, the peel of immature citrus fruits contains relatively high levels of flavonoids and antioxidants than those in mature fruit peels [Citation7,Citation8].
C. tumida hort. ex Tanaka is a native citrus found around Mt. Tsukuba in Ibaraki prefecture, Japan [Citation9]. C. tumida contains high levels of hesperidin and nobiletin than those in other species of citrus fruits [Citation10]. However, the potential health benefits of C. tumida have not been fully investigated. Therefore, we aimed to elucidate the health benefits of C. tumida, especially for preventing obesity and depression, which are linked to inflammation. Recently, we reported the significant functions including anti-obesity and anti-depression in the peel of immature C. tumida (PIC) using the rodent models [Citation11,Citation12]. In this study, we tried to clarify the global effects of dietary PIC on the metabolism to understand the potentials of C. tumida. We first carried out a comprehensive metabolite analysis for mice fed PIC under a normal diet (AIN-93G) but not a high fat diet, because this was the first trial for analyzing the effects of dietary PIC on the metabolomics in mice. Omics approaches are considered valuable tools to study the effects of food and farm products on health [Citation13]. Metabolomics is frequently employed to elucidate food-induced alterations in global metabolism in humans and other animals. To follow the our previous study showing the dietary PIC impacts on the lipid metabolism in mice [Citation11], we focused on the food intake (FI), body weight gain (BWG), and the metabolome of the plasma and liver under the normal diet feeding with PIC.
Materials and methods
Animals and plant materials
This study was approved by the Animal Care and Use Committee of Ibaraki University and conforms to the guidelines of the Ministry of Education, Culture, Sports, Science, and Technology (MEXT), Japan (Notification No. 71).
Male C57BL/6JJmsSlc (B6) mice (7-week-old) obtained from SLC Japan (Shizuoka, Japan) were housed at the animal facility of the College of Agriculture, Ibaraki University under a 12-h light-dark cycle (light on at 8:00 am). Prior to the experiments, the mice were individually housed in cages (143 × 293 × 148 mm, Charles River Laboratories Japan, Kanagawa, Japan) with wood chips. The mice were fed with a semi-purified diet (AIN-93G, Oriental Yeast, Tokyo, Japan). Food and water were available ad libitum and were weighed to monitor the daily consumption. Body weight was also determined daily to calculate BWG.
Immature C. tumida was harvested at an orchard in the eastern foothill of Mt. Tsukuba, Ibaraki prefecture, Japan, in early October 2015. Peels containing the outer orange layer and the inner white layer were manually collected and freeze-dried using a freeze-dryer (FDU-1110, TOKYO RIKAKIKAI, Tokyo, Japan). Dried peels were powdered using a centrifugal mill (ZM-1, Retsch technology GmbH, Haan, Germany). Peel powder was stored at room temperature (23–26°C) until use.
Detection of flavonoids by high-performance liquid chromatography
To determine the flavonoid levels in PIC, concentrations of nobiletin, narirutin, geosmin, hesperidin, and tangeretin were simultaneously analyzed by high-performance liquid chromatography (HPLC) (Hitachi Chromaster System, Hitachi, Tokyo, Japan). Flavonoid standards were purchased from Wako Pure Chemical Industries, Ltd (Osaka, Japan). Dried PIC powder (100 mg) was mixed and extracted with 4 mL methanol:dimethyl sulfoxide (1:1) with agitation for 12 h at room temperature using a shaker (NR-10, Taitec, Tokyo, Japan). After the eluted solution was centrifuged at 1000 g for 5 min, the supernatant was collected and filtered through a membrane filter (Millex-GS 0.22 μm, Merck Millipore, Darmstadt, Germany). Samples were stored at −80°C until analysis. A 10-μL sample aliquot was injected into the HPLC apparatus and analyzed with a photodiode array detector. ZORBAX SB-C8 (150 × 3.0 mm i.d.) (Agilent Technologies, Tokyo, Japan) was used as a separation column, and Agilent Hardware Kit High Press was used as a guard column (Agilent Technologies). The temperature of the column oven was set at 40°C and spectra from 200 to 450 nm were obtained. The linear gradient elution program consisted of an initial 20 min (mobile phase, from 80% and 20% to 0% and 100% of formic acid and methanol, respectively) followed by 5 min of 100% methanol at a flow rate of 1.0 mL/min. Concentrations of the compounds were calculated from integrated peak areas of the sample and the corresponding authentic standards.
Experimental design
After acclimatization to the environment of the animal facility in Ibaraki University for one week, the B6 mice were divided into two groups: control (n = 6) and PIC-fed (n = 7). The PIC-fed group was fed 5% (w/w) PIC powder in the AIN93G powder diet, whereas the control group was fed only AIN93G powder for four weeks.
Tissue sampling
After fasting from 9:00 am to 12:00 am, the mice were sacrificed by decapitation and trunk blood was collected in a tube on ice. Final concentration was set at 0.13% EDTA-2K. The sample was centrifuged at 1,200 g at 23°C for 10 min. The supernatant blood plasma was collected and stored at −80°C until use. Approximately 50 mg from the left lobe of the liver was removed and immediately frozen in liquid nitrogen and stored at −80°C until use. Epididymal, perirenal, and subcutaneous fats were collected and weighed. Tissue weight was normalized to body weight (BW) on the day of sampling.
Metabolomic analysis of plasma and liver
Nine representative mice were selected for the metabolome analysis. The plasma and liver samples (n = 5 in the control group, n = 4 in PIC-fed group) were subjected to metabolomic analysis as previously reported [Citation14]. Sample preparation and metabolome analysis were carried out by HMT (Human Metabolome Technology Inc. Tsuruoka, Japan). Capillary electrophoresis time-of-flight mass spectrometry (CE-TOFMS) analysis of the metabolome was performed using an Agilent CE Capillary Electrophoresis System equipped with an Agilent 6210 Time of Flight mass spectrometer (Agilent Technologies, Waldbronn, Germany) at HMT following previously described protocols [Citation14–Citation17]. Briefly, approximately 50 mg frozen liver sample was immersed in 1800 μL 50% acetonitrile in Milli-Q water (Millipore-Japan, Tokyo, Japan) containing internal standards (H3304-1002, HMT). The tissue was homogenized using the BMS-M10N21 homogenizer (BMS, Tokyo, Japan) and then centrifuged at 2300 g for 5 min at 4°C. Next, 800 μL of the upper layer was filtered by centrifugation using an HMT 5-kDa cutoff filter (UFC3LCCNB-HMT, HMT) at 9100 g for 120 min at 4°C. The filtrate was resuspended in 50 μL Milli-Q water for CE-MS analysis.
For plasma, 50 μL sample was added to 450 μL methanol containing internal standards (H3304-1002, HMT). The solution was mixed with 500 μL chloroform and 200 μL Milli-Q water and centrifuged at 2300 g for 5 min at 4°C. Next, 400 μL of the upper layer was filtered through an HMT 5-kDa cutoff filter as described above. The filtrate was then resuspended in 25 μL Milli-Q water for CE-MS analysis.
The identified metabolites from the metabolome library were assigned to the Kyoto Encyclopedia of Genes and Genomes (KEGG), facilitating the search for the corresponding metabolic pathways [Citation18].
Statistical analysis
BWG, FI, water intake (WI), and tissue weights were analyzed by an unpaired two-tailed Student’s t-test. Data were analyzed using Excel (Microsoft, WA) and are shown as mean ± SEM. For metabolomic analyses, Welch’s t-tests were used to compare the “supplementation” factor. To control for P-values of multiple comparisons, the false discovery rate was determined based on previously published studies [Citation19,Citation20]. The significance threshold was set to Q < 0.1.
Results
Concentrations of major flavonoids in PIC
We analyzed the major flavonoids, including nobiletin, narirutin, geosmin, hesperidin, and tangeretin, in PIC using HPLC (). Unfortunately, nobiletin and narirutin could not be separated under the HPLC conditions described above. Previous data showed that the concentration of nobiletin is approximately 3 times that of narirutin in C. tumida [Citation21]; hence, nobiletin is relatively a major flavonoid in PIC.
Table 1. Flavonoid content in peels of immature Citrus tumida (100 mg).
BWG, FI, WI, and feed efficiency
We measured cumulative BWG, FI, and WI to evaluate the effect of PIC supplementation. Mice from the PIC-fed group showed lower BWG (control: 4.45 ± 0.47 g vs. PIC: 3.20 ± 0.22 g, P = 0.0267, )) and a tendency for lower FI (control: 126.18 ± 3.69 g vs. PIC: 117.76 ± 1.70 g, P = 0.0517, )) when compared to control mice. No significant difference was observed in WI (control: 121.40 ± 4.52 g vs. PIC: 125.10 ± 1.90 g, P = 0.4470, )). Mice from the PIC-fed group also showed a tendency to have a lower feed efficiency than that in control mice (control: 0.035 ± 0.0036 vs. PIC: 0.027 ± 0.0020, P = 0.0706, )).
Figure 1. Effects of supplementation of the peels of immature Citrus tumida (PIC) on (a) cumulative body weight gain, (b) food intake, (c) water intake, and (d) feed efficiency (n = 6–7 in each group).
Control: mice fed with AIN93G diet; PIC: mice fed with AIN93G supplemented with 5% PIC (w/w) diet. Data are expressed as mean ± SEM. †P < 0.10, *P < 0.05, **P < 0.01 versus control.
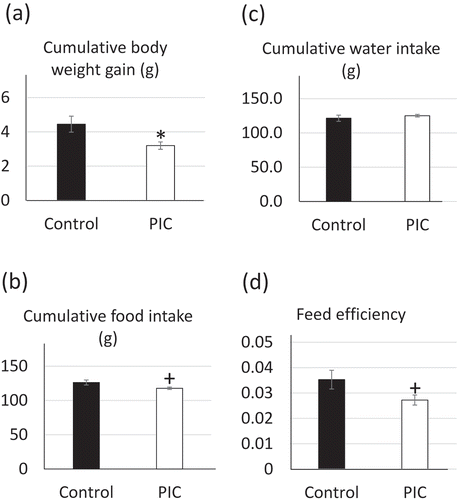
Liver and fat weights
As shown in ), liver weight (as % of BW) of PIC-fed mice was higher than that of control mice (control, 4.01 ± 0.13% vs. PIC, 4.57 ± 0.057%, P = 0.0015). The weight of epididymal, perirenal, and subcutaneous fats (as % BW) in PIC-fed mice was significantly lower than that in control mice (epididymal fats – control: 1.85 ± 0.15% vs. PIC: 1.40 ± 0.047%, P = 0.0104; perirenal fat – control: 0.60 ± 0.067% vs. PIC: 0.35 ± 0.010%, P = 0.0021; subcutaneous fat – control, 0.32 ± 0.026% vs. PIC, 0.22 ± 0.029%, P = 0.0404) (–)).
Figure 2. Effects of supplementation of the peels of immature Citrus tumida (PIC) on (a) cumulative liver weight, (b) epididymal fat weight, (c) perirenal fat weight, and (d) subcutaneous fat weight (n = 6–7 in each group).
Control: mice with fed AIN93G diet; PIC: mice fed with AIN93G containing 5% PIC (w/w) diet. Data are expressed as mean ± SEM. *P < 0.05, **P < 0.01 versus control.
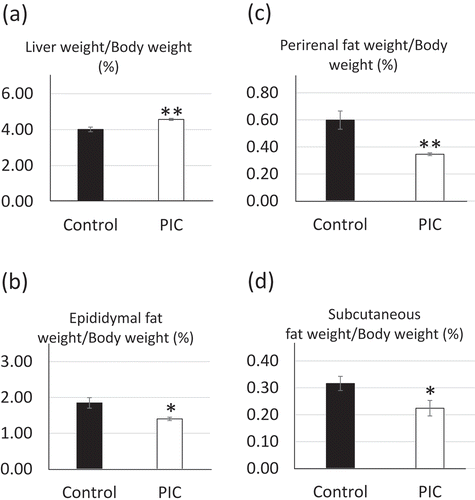
Metabolomics
CE-TOFMS revealed 191 metabolites in the plasma. A single metabolite, 2-hydroxyvaleric acid, showed significantly (P < 0.05 and Q < 0.1) lower levels in PIC-fed mice than in control mice ().
Table 2. Metabolites affected by ingestion of peels of immature Citrus tumida (Q < 0.1).
A total of 250 metabolites were detected by CE-TOFMS in the liver. As shown in , 5 metabolites showed significant differences in levels between PIC-fed and control mice (P < 0.05 and Q < 0.1). The relative amounts of Thr-Asp (and/or Ser-Glu), daminozide (and/or Ala-Ala), glucuronic acid (and/or galacturonic acid-2), and S-methylglutathione were significantly higher in PIC-fed mice than in control mice. In contrast, the relative amount of betaine aldehyde was significantly lower in PIC-fed mice than in control mice.
Discussion
A local citrus fruit, C. tumida has relatively higher levels of potential flavonoids compared to C. unshiu and C. junos [Citation21]. In this study, we investigated the health benefits of C. tumida and focused on the dietary effects of PIC, which contains flavonoids, such as nobiletin and hesperidin. As shown in , PIC contained high amounts of nobiletin and narirutin compared to other flavonoids, although the peaks of nobiletin and narirutin could not be separated under the conditions described in the Methods. Supplementation of PIC significantly suppressed BWG compared to that observed with the control diet (P< 0.05, )); although FI and feed efficiency were reduced slightly, the difference was not statistically significant (P< 0.1, ,)). In addition, PIC intake significantly decreased the weight of adipose tissues (P< 0.05, –)). Similarly, our previous report described that dietary PIC decreased adipose tissues following blood triglyceride and total cholesterol in the mouse model of acute obesity [Citation11]. These effects may result from the presence of flavonoids in PIC, which can affect lipid metabolism. In particular, C. tumida contains high levels of hesperidin, nobiletin, and tangeretin [Citation10]. Hesperidin has several biological and pharmacological properties, such as anti-inflammatory, anti-carcinogenic, anti-oxidative, vascular protective, and lipid-lowering activities [Citation22–Citation24]. Moreover, nobiletin and hesperidin repress the expression of genes related to lipid synthesis, such as stearoyl-CoA desaturase [Citation25]. In general, several citrus fruit-flavonoids have anti-inflammatory, insulin-sensitizing, and lipid-lowering activities [Citation1]. Therefore, the flavonoids in PIC may reduce body fat deposition and suppress BWG in mice. On the other hand, the other factors may contribute this action, because the test diet used in this study had relatively lower concentrations of the flavonoids.
Metabolomics is widely used in food science and nutrition research [Citation26], and the use of CE-TOFMS reveals the global effects of food on metabolism [Citation13]. Actually, metabolomics is a reasonable way to understand the comprehensive effects of food functions [Citation13,Citation26,Citation27]. In this study, the plasma and liver metabolites of PIC-fed and control mice were analyzed and compared comprehensively using CE-TOFMS, and significant differences were detected, suggesting that this approach may provide important metabolic information about the effects of oral PIC supplementation. In humans, the metabolomic analysis of the effects of diets, including citrus fruits, was similarly successful [Citation27].
The blood plasma levels of 2-hydroxyvaleric acid (2-hydroxypentanoic acid) decreased in PIC-fed mice (P< 0.05, Q < 0.1, ). 2-Hydroxyvaleric acid is present in human fluids and is related to the pathophysiological metabolites in acidosis [Citation28]. The biological function of 2-hydroxyvaleric acid in animal tissues is unclear. Non-obese diabetic (NOD) mice are widely used in type 1 diabetes studies, and they are divided into progressor and non-progressor groups depending on whether or not the animal shows disease progression [Citation29]. The authors showed that plasma levels of 2-hydroxyvaleric acid are lower in non-progressor than in progressor NOD mice, and therefore, 2-hydroxyvaleric acid may be a good predictor of type 1 diabetes. In addition, plasma levels of 2-hydroxyvaleric acid in humans are decreased by simvastatin, a statin that reduces LDL-cholesterol levels and the risk of cardiovascular disease [Citation30]. It is possible that simvastatin, or a metabolite of the drug, inhibits an enzyme that produces 2-hydroxyvaleric acid. Although the precise mechanism underlying these effects is unknown, PIC may modify the fatty acid metabolism and downregulate the 2-hydroxyvaleric acid synthesis pathway, resulting in lower levels in the plasma.
The levels of glucuronic and UDP-glucuronic acids increased in the liver after PIC supplementation (P< 0.05, , Table S2). Because both of them play a pivotal role in the elimination of toxic substances [Citation31], increased levels of glucuronic and UDP-glucuronic acids by PIC may contribute to enhanced detoxification in the liver.
PIC also increased the levels of galacturonic acid in the liver (P< 0.05, Q < 0.1, ). Galacturonic acid is the main component of pectin [Citation32], which is digested by several fibrolytic enzymes produced by intestinal microorganisms [Citation33]. Pectin is a major component of citrus peels. Therefore, the digestion of PIC produces galacturonic acid in the mouse intestine, and this metabolite is possibly transported to and accumulated in the liver. It has been reported that orally fed pectin and galacturonic acid inhibit hepatic lipogenesis in rats [Citation34]. Furthermore, dietary pectin inhibited intestinal cholesterol absorption in apoE−/- mice [Citation35] and suppressed body weight gain and calorie intake in high fat diet-induced obese rats [Citation36]. Lipid metabolism and food intake regulation should be studied in PIC-fed mice to clarify these and other issues. The functions of other dietary fibers in PIC should also be investigated.
Reduced glutathione (GSH), a major protectant against oxidative stress, is methylated in the SH group of its cysteine moiety and converted to S-methylglutathione. Levels of S-methylglutathione increased in PIC-fed mice compared to those in control mice (P< 0.05, Q < 0.1, ). In addition, oxidized glutathione (GSSG) levels in the liver of PIC-fed mice were slightly higher than those in control mice (P< 0.1, Table S4). PIC may thus be able to increase anti-oxidative stress activity via glutathione metabolism in the liver. In fact, chronic administration of C. unshiu extracts reduces oxidative stress in the liver of diabetic rats by increasing glutathione levels [Citation37]. Future studies are necessary to assess the function of S-methylglutathione in the liver and the effects of increased S-methylglutathione levels induced by PIC on the liver function.
Supplementation of PIC downregulated choline metabolism in the liver (, Table S2, ). GPCho is a product of the breakdown of phosphatidylcholine and is converted to choline by GPCho phosphodiesterase. Choline dehydrogenase produces betaine aldehyde from choline [Citation38], and glycine betaine is converted from betaine aldehyde by aldehyde dehydrogenase [Citation39]. The mechanism underlying the downregulation of choline metabolism in the liver by PIC supplementation remains unclear. Future studies will focus on evaluating the expression of genes related to choline metabolism in the liver of PIC-fed mice, and critical ingredients in PIC downregulating choline metabolism should be discovered.
Figure 3. Choline-related metabolites in the liver.
The pathway of choline-related metabolites in the liver is shown. Welch’s t-tests were used to compare “supplement,” using both peels of immature Citrus tumida (PIC)-fed mice (n = 4) and control mice (n = 5). Control: mice fed with AIN93G diet; PIC: mice fed with AIN93G containing 5% PIC (w/w) diet. Data are expressed as mean ± SEM.
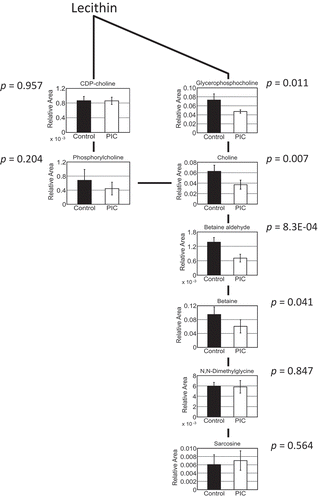
In summary, we evaluated the effects of PIC supplementation on BWG, FI, WI, adipose tissue weight, and metabolomics of blood plasma and liver in mice and concluded that PIC influences body and adipose tissue weights and promotes metabolic alterations. Especially, plasma 2-hydroxyvaleric acid and liver choline metabolism including betaine aldehyde were significantly decreased by dietary PIC. In the future, we will focus on elucidating the mechanisms by which PIC supplementation reduces body fat and changes the levels of some metabolites in mice. This study used a small cohort and might have missed some critical metabolites that are significantly altered by dietary PIC. Therefore, in future studies we will try to carry out metabolomic analyses using larger cohorts of mice and humans to elucidate PIC functions, especially in fat metabolism. These studies will contribute to establish the effects of PIC on human and animal health and provide a better understanding of the health benefits of citrus fruits.
Authors contribution
A. Toyoda, M. Sato, and T. Goto designed the studies. A. Toyoda, M. Sato, M. Muto, Y. Miyaguchi, and E. Inoue performed the experiments. A. Toyoda and T. Goto analyzed the data. A. Toyoda wrote the manuscript.
Supporting_information.docx
Download MS Word (32 KB)Supplementary_Table_5..xlsx
Download MS Excel (12.1 KB)Supplemantary_Table_4-h.xlsx
Download MS Excel (87.6 KB)Supplementary_Table_3-h.xlsx
Download MS Excel (72 KB)Table_S2-h.xlsx
Download MS Excel (12.7 KB)Table_S1-h.xlsx
Download MS Excel (12.1 KB)Acknowledgments
We thank H. Shimonishi and T. Fujii (Ibaraki University) for valuable technical assistance.
Disclosure statement
No potential conflict of interest was reported by the authors.
Data availability statement
The data described in this article are openly available in the Open Science Framework at DOI:10.17605/OSF.IO/TPA6U.
Supplementary material
Supplemental data for this article can be accessed here.
Additional information
Funding
References
- Mulvihill EE, Burke AC, Huff MW. Citrus flavonoids as regulators of lipoprotein metabolism and atherosclerosis. Annu Rev Nutr. 2016;36:275–299.
- Ito A, Shin N, Tsuchida T, et al. Antianxiety-like effects of chimpi (dried Citrus peels) in the elevated open-platform test. Molecules. 2013;18:10014–10023.
- Lee Y-S, Cha B-Y, Saito K, et al. Effects of a Citrus depressa Hayata (shiikuwasa) extract on obesity in high-fat diet-induced obese mice. Phytomedicine. 2011;18:648–654.
- Kim A, Im M, Gu MJ, et al. Citrus unshiu peel extract alleviates cancer-induced weight loss in mice bearing CT-26 adenocarcinoma. Sci Rep. 2016;6:24214.
- He B, Nohara K, Park N, et al. The small molecule nobiletin targets the molecular oscillator to enhance circadian rhythms and protect against metabolic syndrome. Cell Metab. 2016;23:610–621.
- Rizza S, Muniyappa R, Iantorno M, et al. Citrus polyphenol hesperidin stimulates production of nitric oxide in endothelial cells while improving endothelial function and reducing inflammatory markers in patients with metabolic syndrome. J Clin Endocrinol Metab. 2011;96:E782–92.
- Choi S-Y, Ko H-C, Ko S-Y, et al. Correlation between flavonoid content and the NO production inhibitory activity of peel extracts from various citrus fruits. Biol Pharm Bull. 2007;30:772–778.
- Barreca D, Bellocco E, Caristi C, et al. Flavonoid composition and antioxidant activity of juices from Chinotto (Citrus x myrtifolia Raf.) fruits at different ripening stages. J Agric Food Chem. 2010;58:3031–3036.
- Tanaka C. Species problem in citrus a critical study of wild and cultivated units of citrus, based upon field studies in their native homes. Jpn Soc Promotion Sci. 1954;9:139. Tokyo: Japanese Society for the Promotion of Science.
- Kawaii S, Tomono Y, Katase E, et al. Quantitation of flavonoid constituents in citrus fruits. J Agric Food Chem. 1999;47:3565–3571.
- Sato M, Goto T, Inoue E, et al. Dietary intake of immature Citrus tumida hort. ex Tanaka peels suppressed body weight gain and fat accumulation in an acute mouse model of obesity. J Nutr Sci Vitaminol. 2019;65:19–23.
- Sato M, Okuno A, Suzuki K, et al. Dietary intake of the citrus flavonoid hesperidin affects stress-resilience and brain kynurenine levels in a subchronic and mild social defeat stress model in mice. Biosci Biotech Biochem. 2019;83:1756–1765.
- Takahashi S, Saito K, Jia H, et al. An integrated multi-omics study revealed metabolic alterations underlying the effects of coffee consumption. PLoS One. 2014;9(9):e91134.
- Goto T, Kubota Y, Toyoda A. Plasma and liver metabolic profiles in mice subjected to subchronic and mild social defeat stress. J Proteome Res. 2015;14:1025–1032.
- Soga T, Heiger DN. Amino acid analysis by capillary electrophoresis electrospray ionization mass spectrometry. Anal Chem. 2000;72:1236–1241.
- Soga T, Ohashi Y, Ueno Y, et al. Quantitative metabolome analysis using capillary electrophoresis mass spectrometry. J Proteome Res. 2003;2:488–494.
- Soga T, Ueno Y, Naraoka H, et al. Simultaneous determination of anionic intermediates for Bacillus subtilis metabolic pathways by capillary electrophoresis electrospray ionization mass spectrometry. Anal Chem. 2002;74:2233–2239.
- Kanehisa M, Goto S, Sato Y, et al. Data, information, knowledge and principle: back to metabolism in KEGG. Nucleic Acids Res. 2014;42:D199–D205.
- Benjamini Y, Hochberg Y. Controlling the false discovery rate: a practical and powerful approach to multiple testing. J R Stat Soc. 1995;289–300. DOI:10.2307/2346101
- Storey JD, Tibshirani R. Statistical significance for genomewide studies. Proc Natl Acad Sci U S A. 2003;100:9440–9445.
- Sakai S, Nakagawa R. Studies on the functional ingradients in the farm products in Ibaraki prefecture. Bulletins of industrial technology innovation center of Ibaraki prefecture. 2011. 40. Available from: http://www.itic.pref.ibaraki.jp/periodical/reseach/40/vol40-04.pdf (in Japanese).
- Miyake Y, Yamamoto K, Tsujihara N, et al. Protective effects of lemon flavonoids on oxidative stress in diabetic rats. Lipids. 1998;33:689–695.
- Chiba H, Uehara M, Wu J, et al. Hesperidin, a citrus flavonoid, inhibits bone loss and decreases serum and hepatic lipids in ovariectomized mice. J Nutr. 2003;133:1892–1897.
- Morand C, Dubray C, Milenkovic D, et al. Hesperidin contributes to the vascular protective effects of orange juice: a randomized crossover study in healthy volunteers. Am J Clin Nutr. 2011;93:73–80.
- Nichols LA, Jackson DE, Manthey JA, et al. Citrus flavonoids repress the mRNA for stearoyl-CoA desaturase, a key enzyme in lipid synthesis and obesity control, in rat primary hepatocytes. Lipids Health Dis. 2011;10:36.
- Wishart DS. Metabolomics: applications to food science and nutrition research. Trends Food Sci Technol. 2008;19:482–493. . Elsevier
- May DH, Navarro SL, Ruczinski I, et al. Metabolomic profiling of urine: response to a randomised, controlled feeding study of select fruits and vegetables, and application to an observational study. Br J Nutr. 2013;110:1760–1770.
- Asano K, Miyamoto I, Matsushita T, et al. Succinic acidemia: a new syndrome of organic acidemia associated with congenital lactic acidosis and decreased NADH-cytochrome c reductase activity. Clin Chim Acta. 1988;173:305–312.
- Grapov D, Fahrmann J, Hwang J, et al. Diabetes associated metabolomic perturbations in NOD mice. Metabolomics. 2015;11:425–437.
- Trupp M, Zhu H, Wikoff WR, et al. Metabolomics reveals amino acids contribute to variation in response to simvastatin treatment. Oresic M, editor. PLoS One. 2012;7:e38386.
- Rowland A, Miners JO, Mackenzie PI. The UDP-glucuronosyltransferases: their role in drug metabolism and detoxification. Int J Biochem Cell Biol. 2013;45:1121–1132.
- Mohnen D. Pectin structure and biosynthesis. Curr Opin Plant Biol. 2008;11:266–277.
- Matsuura Y. Pectic acid degrading enzymes from human feces. Agric Biol Chem. 1991;55:885–886.
- Suzuki M, Kajuu T. Suppression of hepatic lipogenesis by pectin and galacturonic acid orally-fed at the separate timing from digestion-absorption of nutrients in rat. J Nutr Sci Vitaminol. 1983;29:553–562.
- Chen Y, Xu C, Huang R, et al. Butyrate from pectin fermentation inhibits intestinal cholesterol absorption and attenuates atherosclerosis in apolipoprotein E-deficient mice. J Nutr Biochem. 2018;56:175–182.
- Adam CL, Gratz SW, Peinado DI, et al. Effects of dietary fibre (pectin) and/or increased protein (casein or pea) on satiety, body weight, Aadiposity and caecal fermentation in high fat diet-induced obese rats. PLoS One. 2016;11:e0155871.
- Sugiura M, Ohshima M, Ogawa K, et al. Chronic administration of Satsuma mandarin fruit (Citrus unshiu Marc.) improves oxidative stress in streptozotocin-induced diabetic rat liver. Biol Pharm Bull. 2006;29:588–591.
- Yilmaz JL, Bülow L. Enhanced stress tolerance in Escherichia coli and Nicotiana tabacum expressing a betaine aldehyde dehydrogenase/choline dehydrogenase fusion protein. Biotechnol Prog. 2002;18:1176–1182.
- Chern MK, Pietruszko R. Human aldehyde dehydrogenase E3 isozyme is a betaine aldehyde dehydrogenase. Biochem Biophys Res Commun. 1995;213:561–568.