ABSTRACT
As a respiratory disease with high morbidity and mortality, pulmonary fibrosis (PF) has been a serious threat to people’s health. Hederagenin (HDG) is a pentacyclic triterpenoid saponin widely distributed in various plants. This study explored the role of HDG in Bleomycin (BLM)-induced PF and the molecular mechanism. The results showed that HDG reduced BLM-induced pulmonary dysfunction, pathological damage in a dose-dependent manner. Besides, HDG reduced BLM-induced collagen deposition by decreasing the levels of α-SMA, Collagen I and hydroxproline. Furthermore, HDG reduced the levels of inflammatory cytokines (TNF-α and IL-6), TGF-β1 and connective tissue growth factor (CTGF) in bronchoalveolar lavage fluid (BALF) or serum. Further mechanism analysis indicated that HDG inhibited the expression of Ras and phosphorylation of JNK and NFAT4 in a dose-dependent manner. However, the JNK pathway activator Anisomycin reversed this inhibitory effect. In conclusion, these findings suggest that HDG may be a potential target drug for PF therapy.
GRAPHICAL ABSTRACT
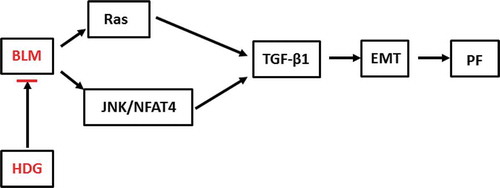
Hederagenin alleviates bleomycin-induced pulmonary fibrosis by regulating Ras/JNK/NFAT4 pathway in rats
Pulmonary fibrosis (PF) is an irreversible respiratory disease with high morbidity and mortality that poses a serious threat to human health [Citation1,Citation2]. Studies have shown that PF can cause excessive deposition of collagen, thickening of lung tissue and decrease of alveolar ventilation, eventually leading to organ dysfunction and failure [Citation3]. PF is a progressive pulmonary disease [Citation4]. At present, glucocorticoids and immunosuppressants are commonlyused in the treatment of PF, but the therapeutic effect is not satisfactory and there aresserious side effects [Citation5]. Therefore, it is very important to explore the pathogenesis of PF and find new anti-fibrotic drugs for the early intervention and treatment of PF.
Hederagenin (HDG, C30H48O4) is a pentacyclic triterpenoid saponin widely distributed in various plants, such as Dipsacaceae, Caprifoliaceae, Ranunculaceae, Araliaceae and Valerianaceae. Studies have shown that HDG has many pharmacological activities, such as anti-lipid peroxidation [Citation6], anti-inflammatory [Citation7], hyperlipidemia therapy [Citation8], anti-edema [Citation7], anti-platelet aggregation [Citation9] and liver protection [Citation8]. Previous studies have shown that HDG has a protective effect on vascular walls, and has a good preventive and therapeutic effect on atherosclerosis [Citation10]. Besides, HDG is also involved in the treatment of liver fibrosis as one of the ingredients of the Fuzheng Huayu formula [Citation11]. However, whether HDG improves PF and the underlying molecular mechanisms remains unknown.
Alveolar epithelial type 2 cells can develop into pulmonary myofibroblasts through epithelial-mesenchymal transition (EMT). Subsequently, deposition of extracellular matrix (ECM) promotes the transformation of myofibroblasts into idiopathic PF. Ras is a key protein in the PTKs-mediated signaling pathway. Cumulative studies have shown that PF is involved in the Ras/ERK1/2 signaling pathway [Citation12,Citation13]. However, different upstream regulators make Ras/ERK1/2 have different effects on PF. For example, fibroblast growth factor 2 (FGF2) inhibited PF by stimulating the activation of Ras/ERK1/2 and negatively regulating TGF-β1-induced EMT [Citation14]. Conversely, epidermal growth factor receptor (EGFR) enhanced the TGFβ-induced profibrotic response by inducing the expression of ZEB1 and downstream tissue plasminogen activator (tPA) through activating Ras/ERK1/2 pathway [Citation13]. As a fibroticmedium, JNK plays an important role in the process of fibrosis [Citation15]. IGFBP-5 promoted PF by modulating the production of ROS via regulating MEK/ERK and JNK signaling pathways [Citation16]. Besides, JNK also activated T cell nuclear factor 4 (NFAT4) to participate in the regulation of related gene expressions [Citation17]. However, whether HDG can regulate PF through Ras/JNK/NFAT4 axis remains unknown.
This study investigated the role of HDG in PF rats and its underlying molecular mechanisms. These findings suggested that HDG alleviated pulmonary dysfunction and reduced fibrosis level in PF rats via Ras/JNK/NFAT4 axis, suggesting that HDG may be a potential target drug for PF therapy.
Material and methods
Animal model
Animal procedures were approved by the Institutional Animal Care and Use Committee of Hospital of Chengdu University of Traditional Chinese Medicine. Sixty adult SD rats (male, weight 220 ± 20 g) were purchased from the Animal Center of the Hospital of Chengdu University of Traditional Chinese Medicine. Rats were housed in an environment with a constant temperature (22–25°C) and constant humidity (45–60%) and given a 12 h light/dark cycle and random access to food and water. HDG was obtained from Sigma Aldrich (St. Louis, Missouri, USA). 1 mg HDG was dissolved in 1 mL dimethyl sulfoxide and then diluted to different concentrations (10 mg/kg, 20 mg/kg and 50 mg/kg). The rats were randomly divided into 6 groups (n = 10): Control group (Rats were intratracheally infused with 0.9% saline), PF group (Rats were intratracheally infused with 5 mg/kg 5% BLM), HDG (10 mg/kg) group (PF rats were orally administered with 10 mg/kg HDG), HDG (20 mg/kg) group (PF rats were orally administered with 20 mg/kg HDG), HDG (50 mg/kg) group (PF rats were orally administered with 50 mg/kg HDG) and HDG + Anisomycin group (PF rats were orally administered with 50 mg/kg HDG and 5 mg/kg Anisomycin). On the 2nd day after modeling, rats in the control group and the PF group were given 1 mL/100 g of 0.9% saline once a day, while rats in the HDG groups were given different doses of HDG (10 mg/kg, 20 mg/kg and 50 mg/kg) daily. The alveolitis and PF scores were assessed using the SZAPIEL method [Citation18], and rats were anesthetized with 1% pentobarbital sodium (45 mg/kg) intraperitoneally after 28 days. The trachea was tracheotomized, intubated and connected to the lung function test system for lung function analysis (BUXCO, USA). After that, the rats were sacrificed. Bronchoalveolar lavage fluid (BALF), serum and lung tissue were harvested according to previously described methods and stored at −20°C [Citation19].
Pathological analysis
The left lung tissue was fixed with paraformaldehyde (40 g/L) and embedded in paraffin. The lung tissue was then cut into sections (5 μm). Pathological damage of lung tissues was detected by hematoxylin & eosin (HE) staining and Masson trichrome staining, respectively. Briefly, paraffin-embedded lung tissue sections were dewaxed and hydrated. For HE staining, sections were orderly stained with hematoxylin for 5 min and eosin for 3 min. For MASSON staining, the nuclei were orderly stained with Regaud hematoxylin dye for 8 min and Masson Ponceau Red Acid Fuchsin solution for 10 min. Whereafter, the sections were developed for 5 min with a 1% aqueous solution of phosphomolybdic acid and stained with an aniline blue or light green dye solution for 5 min.
Determination of hydroxyproline
Total lung collagen was analyzed by determination of hydroxyproline. In brief, the left lung tissue was placed in liquid nitrogen overnight and stored at −70 oC. The hypothermic lung tissue was ground into homogenate and dissoloved in HCl (2 mol/L). Subsequently, the lung homogenate was exposed to 110 °C overnight and then cooled to room temperature. After adding chloramine T solution for 20 min, the mixture and Ehrlich solution were co-incubated at 65°C for 20 min. The absorbance at 550 nm was measured with a spectrophotometer.
ELISA
Levels of TNF-α and IL-6 in BALF and serum were measured using ELISA kits according to the manufacturer’s instructions. Besides, levels of TGF-β1 and connective tissue growth factor (CTGF) in serum were also measured using ELISA kits.
Western blotting
The left lung tissue was placed in liquid nitrogen overnight and stored at −70 oC. The hypothermic lung tissue was ground into homogenate. Then, the protein in the lung homogenate were separated by SDS-PAGE and transferred to PVDF membrane. Subsequently, the membrane and primary antibodies anti-Ras (ab52939, 1:5000, Abcam, Cambridge, UK), anti-JNK (ab208035, 1:5000, Abcam, Cambridge, UK), anti-phospho JNK (ab219584, 1:5000, Abcam, Cambridge, UK), anti-NFAT4 (ab93628, 1:5000, Abcam, Cambridge, UK), anti-phospho NFAT4 (ab182649, 1:5000, Abcam, Cambridge, UK) and anti-β-actin (ab8227, 1:5000, Abcam, Cambridge, UK) were incubated at 4 °C overnight. Thereafter, the membranes were co-cultured with Goat Anti-Rabbit IgG H&L (HRP, ab205718) and visualized using a chemiluminescent system (GE Healthcare, Beijing, China).
Statistical analysis
Data in the study were expressed as mean ± SD. one-way ANOVA and post hoc test was employed to compare the differences among groups. P < 0.05 were regarded as statistically significant.
Results
HDG reversed BLM-induced pulmonary functionthe impairment in PF rats
PF is often accompanied by loss of pulmonary function. This study constructed BLM-induced PF SD rat model. As shown in , BLM treatment significantly reduced dynamic compliance, maximum vital capacity, mean expiratory flow, and maximum inspiratory volume in SD rats. However, HDG treatment, especially at doses of 20 mg/kg and 50 mg/kg, dose-dependently reversed BLM-induced pulmonary dysfunction. These findings indicated that HDG reversed BLM-induced pulmonary function impairment in PF rats.
Figure 1. HDG reversed BLM-induced pulmonary functionthe impairment in PF rats.
Rats were randomly divided into 5 groups (n = 10): Control group, PF group, HDG (10 mg/kg) group, HDG (20 mg/kg) group and HDG (50 mg/kg) group. a. Dynamic compliance. b. Mean MID expiratory flow. c. Forced vital capacity. d. Inspiratory capacity. (** p < 0.01 vs control group; # p < 0.05, ## p < 0.01 vs PF group)
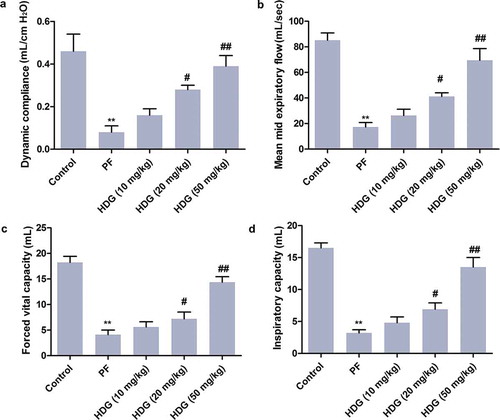
HDG improved the pathological lesion induced by BLM in PF rats
First, alveolitis and PF scores indicated that HDG treatment inhibited BLM-induced alveolitis and PF (). Besides, the pulmonary lesions in rats was further examined by HE staining. As shown in ), complete and clear alveoli, normal stroma and fewer inflammatory cells were observed in the control group. However, the alveoli were destroyed in BLM-treated PF rats, accompanied by a large number of inflammatory cell infiltration and pulmonary interstitial thickening. Notably, HDG treatment improved BLM-induced lung injury in a dose-dependent manner. These findings revealed that HDG improved BLM-induced pathological lesion of lung tissue in PF rats.
Figure 2. HDG improved the pathological lesion induced by BLM in PF rats.
Rats were randomly divided into 5 groups (n = 10): Control group, PF group, HDG (10 mg/kg) group, HDG (20 mg/kg) group and HDG (50 mg/kg) group. a. Alveolitis score. b. Fibrosis score. c. Pathological lesion was assessed by HE staining. (** p < 0.01 vs control group; # p < 0.05, ## p < 0.01 vs PF group)
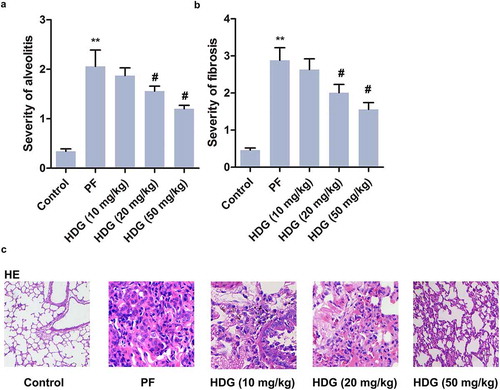
HDG alleviated collagen deposition induced by BLM in PF rats
Similarly, Masson staining revealed that alveolar structure was intact and stroma was normal in the control group. BLM treatment resulted in destruction of alveolar structures, interstitial thickening, and a significant increase in collagen fibers. Interestingly, HDG treatment improved BLM-induced PF in a dose-dependent manner ()). Besides, total lung collagen in this study was identified by hydroxyproline analysis. The results indicated that BLM treatment dramatically increased the content of hydroxyproline. Conversely, HDG treatment reversed the BLM-induced increase in hydroxyproline in a dose-dependent manner ()). In addition, western blotting showed that BLM treatment promoted the expression of α-SMA and Collagen I in PF rats. However, HDG treatment abolished the promotional effect of BLM on α-SMA and Collagen I expression in a dose-dependent manner ()). In summary, these findings suggested that HDG alleviated BLM-induced collagen deposition in the lungs of PF rats.
Figure 3. HDG alleviated collagen deposition induced by BLM in PF rats.
Rats were randomly divided into 5 groups (n = 10): Control group, PF group, HDG (10 mg/kg) group, HDG (20 mg/kg) group and HDG (50 mg/kg) group. a. Fibrosis level was assessed by MASSON staining. b. The level of Hydroxyproline. c. The protein levels of α-SMA and Collagen I was measured by western blotting. (** p < 0.01 vs control group; # p < 0.05 vs PF group)
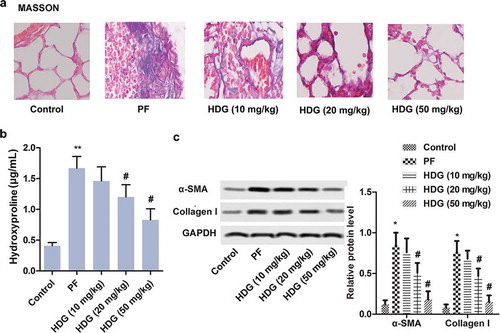
HDG reduced the levels of inflammatory cytokines in PF rats
The levels of inflammatory factors in BALF and serum were measured by ELISA. As shown in ), BLM treatment dramatically increased the levels of TNF-α and IL-6 in BALF and serum. It is worth noting that HDG treatment (especially at doses of 20 mg/kg and 50 mg/kg) counteracted the increase in inflammatory cytokines induced by BLM in a dose-dependent manner. Besides, the levels of TGF- β 1 and CTGF in serum of rats were also examined. As shown in , BLM treatment dramatically increased the levels of TGF-β1 and CTGF in serum. As expected, HDG treatment (20 mg/kg and 50 mg/kg) counteracted BLM-induced increases in TGF-β1 and CTGF levels in a dose-dependent manner. In short, these findings demonstrated that HDG reduced the levels of inflammatory cytokines in PF rats.
Figure 4. HDG reduced the levels of inflammatory cytokines in PF rats.
Rats were randomly divided into 5 groups (n = 10): Control group, PFgroup, HDG (10 mg/kg) group), HDG (20 mg/kg) group and HDG (50 mg/kg) group. a. The level of TNF-α in BALF. b. The level of IL-6 in BALF. c. The level of TNF-α in serum. d. The level of IL-6 in serum. e-f. The levels of TGF-β1 and CTGF in serum. (** p < 0.01 vs control group; # p < 0.05, ## p < 0.01 vs PF group)
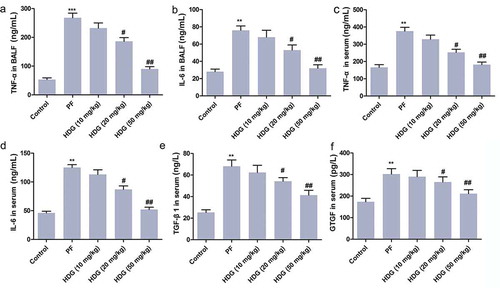
HDG improved BLM-induced fibrosis the level of fibrosis induced by BLM by regulating the Ras/JNK/NFAT4 pathway
Western blotting was used to further investigate the underlying molecular mechanisms of HDG on PF. As shown in ), BLM treatment significantly promoted the expression of Ras and phosphorylation of JNK and NFAT4 in the lung tissue of PF rats compared with the control group. Interestingly, HDG treatment significantly reversed BLM-induced Ras/JNK/NFAT4 pathway activation in a dose-dependent manner. Notably, the JNK activator Anisomycin (5 mg/kg) reversed the inhibitory effect of HDG on the Ras/JNK/NFAT4 pathway ()). Further functional analysis indicated that Anisomycin also reversed the inhibitory effect of HDG on hydroxyproline, forced vital capacity, TNF-α in BALF, and TGF-β1 in serum (). Taken together, these findings suggested that HDG improved BLM-induced fibrosis by regulating the Ras/JNK/NFAT4 pathway ().
Figure 5. HDG improved BLM-induced fibrosis the level of fibrosis induced by BLM by regulating the Ras/JNK/NFAT4 pathway.
a. Rats were randomly divided into 5 groups (n = 10): Control group, PF group, HDG (10 mg/kg) group, HDG (20 mg/kg) group and HDG (50 mg/kg) group. The protein levels of Ras, JNK, p-JNK, NFAT4 and p-NFAT4 were measured by western blotting. b-f. Rats were randomly divided into 4 groups (n = 10): Control group, PF group, HDG (50 mg/kg) group and HDG + Anisomycin group. b. The protein levels of Ras, JNK, p-JNK, NFAT4 and p-NFAT4 were measured by western blotting. c. The level of Hydroxyproline. d. Forced vital capacity. e. The level of TNF-α in BALF. f. The level of TGF-β1 in serum. (** p < 0.01 vs control group; ## p < 0.01 vs PF group, & p < 0.01 vs HDG group)
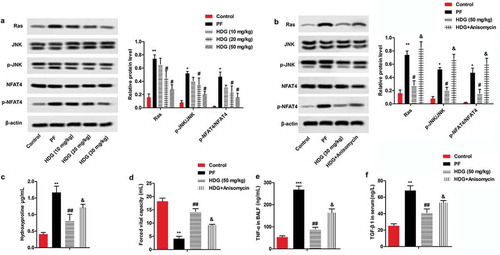
Discussion
As a respiratory disease with high morbidity and mortality, PF poses a serious threat to people’s life [Citation2]. PF-triggered collagen deposition, lung tissue thickening and decreased alveolar ventilationcan lead to organ dysfunction and failure [Citation20]. BLM is a recognized drug that triggers PF [Citation21]. Therefore, this study used BLM to establish PF rat model. Further studies have shown that BLM destroyed the lung tissue structure of rats and caused extensive inflammatory cell infiltration. Besides, BLM treatment also increased fibrosis levels in rats, and significantly increased the levels of hydroxyproline, collagen I and α-SMA. These results are consistent with those reported by previous researchers [Citation22]. Previous studies have shown that PF was associated with inflammatory response [Citation23], EMT [Citation24], fibroblast transformation, and imbalance of matrix metalloproteinase (MMPs)/matrix metalloproteinase inhibitor (TIMP) ratio [Citation25]. Likewise, this study found that the levels of TNF-α and IL-6 in BALF and serum of BLM-treated rats were increased dramatically. TGF-β1 is one of the most widely studied pro-fibrotic factors and plays a crucial role in the progression of PF [Citation26]. CTGF is a key factor in organ dysfunction and complete loss of chronic fibrosis and proliferative diseases [Citation27]. As expected, this study found that BLM treatment also dramatically increased the levels of TGF-β1 and CTGF in rat serum. At present, the therapeutic effect of PF is poor, with serious side effects [Citation5]. This study found that HDG, as one of active ingredients of various plants, could be used as a potential target drug for PF therapy.
As a natural active ingredient, HDG has been found to have a variety of pharmacological effects, such as anti-tumor, anti-inflammation and anti-fibrosis. Fang et al. found that HDG inhibited the proliferation of cervical cancer cells and promoted apoptosis by inhibiting the activation of STAT3 signaling pathway [Citation28]. Besides, Kim et al suggested that HDG prevented liver damage from alcohol by regulating AKT and mitogen-activated protein kinase (MAPK) signaling pathways to inhibit inflammatory response and apoptosis [Citation29]. Similarly, Lu et al. revealed that HDG prevented and treated atherosclerosis by inhibiting the IKKβ/NF-κB signaling pathway and reducing the release of inflammatory factors [Citation8]. HDG is one of the traditional Chinese medicine ingredients against fibrosis, which can improve the level of liver fibrosis by increasing the expression of PI3K and inhibiting the expression of apoptotic proteins [Citation11]. However, the effect of HDG on PF remains unknown. In this study, HDG ameliorated BLM-induced pulmonary dysfunction and pathological damage in PF rats in a dose-dependent manner. Further analysis showed that HDG could improve PF by inhibiting the expression of α-SMA and Collagen. Similarly, this study found that HDG reversed BLM-induced increases in inflammatory cytokines in a dose-dependent manner. Taken together, these findings suggest that HDG may be a potential target drug for PF therapy.
EMT is an important process for the formation of pulmonary myofibroblasts [Citation30]. TGF-β1 is involved in the transformation of alveolar epithelial type 2 cells into pulmonary myofibroblasts throught EMT [Citation31]. Previous studies have shown that the Ras/ERK1/2 signaling pathway regulated PF by regulating the TGF-β1-induced EMT processes [Citation12]. FGF2 inhibited PF by negatively regulating TGF-β1-induced EMT via activating the Ras/ERK1/2 pathway [Citation14]. However, in Yao et al.’s study, the expression of EGFR-induced transcription factor ZEB1 and downstream tPA stimulated the activation of Ras/ERK1/2, thereby enhancing the TGFβ-induced profibrotic response [Citation13]. On this basis, this study found that HDG could regulate PF through Ras/ERK1/2 axis and JNK. Besides, NFAT4 is also involved in the regulation of PF by HDG. In addition, this study found that HDG down-regulated the levels of TGF-β1, CTGF and inflammatory factors, reduces collagen fiber content in PF rats, and improved pulmonary dysfunction in a dose-dependent manner. However, the effect of HDG on PF-regulated target proteins remains to be further explored.
In conclusion, this study explored the effect of HDG on PF in rats and the underlying molecular mechanisms (). Our study revealed that HDG attenuated BLM-induced pulmonary dysfunction and pulmonary fibrosis in a dose-dependent manner. Further mechanism analysis indicated that HDG plays a role by regulating the Ras/JNK/NFAT4 axis. Taken together, these findings suggest that HDG may be a potential target drug for PF therapy.
Authors’ contributions
WM did all the experiments, analyzed all data and was a major contributor in writing the manuscript. QH, GX, LD and YH did some experiment work. All authors read and approved the final manuscript.
Ethics approval and informed consent
Ethical approval was obtained from the Ethics Committee of Hospital of Chengdu University of Traditional Chinese Medicine.
Disclosure statement
No potential conflict of interest was reported by the authors.
Data availability statement
The data described in this article are openly available in the Open Science Framework at DOI:10.17605/OSF.IO/TPA6U.
References
- Richeldi L, Collard HR, Jones MG. Idiopathic pulmonary fibrosis. Lancet. 2017 May 13;389(10082):1941–1952.
- Noble PW, Barkauskas CE, Jiang D. Pulmonary fibrosis: patterns and perpetrators. J Clin Invest. 2012 Aug;122(8):2756–2762.
- Du MY, Duan JX, Zhang CY, et al. Psoralen attenuates bleomycin-induced pulmonary fibrosis in mice through inhibiting myofibroblast activation and collagen deposition. Cell Bio Int. 2019 Jul 22;44:98–107.
- Zuo S, Zhu Z, Liu Y, et al. CXCL16 induces the progression of pulmonary fibrosis through promoting the phosphorylation of STAT3. Can Respir J. 2019;2019:2697376.
- Du Bois RM. Strategies for treating idiopathic pulmonary fibrosis. Nat Rev Drug Discov. 2010 Feb 9;9(2):129–140.
- Choi L, Han YN, Lee KT, et al. Anti-lipid peroxidative principles from the stem bark of kalopanax pictus nakai. Arch Pharm Res. 2001 Dec;24(6):536–540.
- Lee CW, Park SM, Zhao R, et al. Hederagenin, a major component of clematis mandshurica ruprecht root, attenuates inflammatory responses in RAW 264.7 cells and in mice. Int Immunopharmacol. 2015 Dec;29(2):528–537.
- Lu SH, Guan JH, Huang YL, et al. Experimental study of antiatherosclerosis effects with hederagenin in rats. Evid Based Complement Alternat Med. 2015;2015:456354.
- Jin JL, Lee YY, Heo JE, et al. Anti-platelet pentacyclic triterpenoids from leaves of campsis grandiflora. Arch Pharm Res. 2004 Apr;27(4):376–380.
- Lu S-H, Guan J-H, Huang Y-L, et al. Experimental study of antiatherosclerosis effects with hederagenin in rats. Evid Based Complement Alternat Med. 2015;2015:456354.
- Wu R, Dong S, Cai FF. Active compounds derived from fuzheng huayu formula protect hepatic parenchymal cells from apoptosis based on network pharmacology and transcriptomic analysis. Molecules. 2019 Jan 18;24(2):338.
- Liu X, Gao S, Xu H. lncRNAPCAT29 inhibits pulmonary fibrosis via the TGFbeta1regulated RASAL1/ERK1/2 signal pathway. Mol Med Rep. 2018 Jun;17(6):7781–7788.
- Yao L, Conforti F, Hill C, et al. Paracrine signalling during ZEB1-mediated epithelial-mesenchymal transition augments local myofibroblast differentiation in lung fibrosis. Cell Death and Differentiation. 2019 May;26(5):943–957.
- Watanabe-Takano H, Takano K, Hatano M, et al. DA-Raf-Mediated suppression of the Ras–ERK pathway is essential for TGF-beta1-induced epithelial-mesenchymal transition in alveolar epithelial type 2 cells. PloS One. 2015;10(5):e0127888.
- Walker NM, Mazzoni SM, Vittal R, et al. c-Jun N-terminal kinase (JNK)-mediated induction of mSin1 expression and mTORC2 activation in mesenchymal cells during fibrosis. J Biol Chem. 2018 Nov 2;293(44):17229–17239.
- Yasuoka H, Garrett SM, Nguyen XX, et al. NADPH oxidase-mediated induction of reactive oxygen species and extracellular matrix deposition by insulin-like growth factor binding protein-5. Am J Physiol Lung Cell Mol Physiol. 2019 Apr 1;316(4):L644–l655.
- Chow CW, Rincon M, Cavanagh J, et al. Nuclear accumulation of NFAT4 opposed by the JNK signal transduction pathway. Science. 1997 Nov 28;278(5343):1638–1641.
- Shariati S, Kalantar H, Pashmforoosh M, et al. Epicatechin protective effects on bleomycin-induced pulmonary oxidative stress and fibrosis in mice. Biomed Pharmacothe. 2019;114:108776.
- Huang C, Li Y, Fan X, et al. [Effects of combination of Salvia and Ligustrazine on TNF-alpha and TGF-beta1 in serum and BALF of rats with pulmonary fibrosis]. Xi Bao Yu Fen Zi Mian Yi Xue Za Zhi. 2013 Jul;29(7):673–676.
- Nho RS, Polunovsky V. Translational control of the fibroblast-extracellular matrix association: an application to pulmonary fibrosis. Transl (Austin, Tex). 2013;1(1):e23934.
- Liu T, De Los Santos FG, Phan SH. The bleomycin model of pulmonary fibrosis. Methods Mol Biol. 2017;1627:27–42.
- Meng L, Zhang X. Yangyin Yiqi mixture ameliorates bleomycin-induced pulmonary fibrosis in rats through inhibiting TGF-beta1/smad pathway and epithelial to mesenchymal transition. Evid-Based Compl Alt. 2019;2019:2710509.
- Beach TA, Johnston CJ, Groves AM, et al. Radiation induced pulmonary fibrosis as a model of progressive fibrosis: contributions of DNA damage, inflammatory response and cellular senescence genes. Exp Lung Res. 2017 Apr;43(3):134–149.
- Hill C, Li J, Liu D, et al. Autophagy inhibition-mediated epithelial-mesenchymal transition augments local myofibroblast differentiation in pulmonary fibrosis. Cell Death Dis. 2019 Aug 7;10(8):591.
- Zhang Q, Guo Y, Dong R, et al. Suppressor of cytokine signaling 1-modulated metalloproteinases and tissue inhibitor of metalloproteinase in pulmonary fibrosis. Mol Med Rep. 2015 Sep;12(3):3855–3861.
- Prashanth Goud M, Bale S, Pulivendala G, et al. Therapeutic effects of Nimbolide, an autophagy regulator, in ameliorating pulmonary fibrosis through attenuation of TGF-beta1 driven epithelial-to-mesenchymal transition. Int Immunopharmacol. 2019 Aug 1;75:105755.
- Zhao W, Yue X, Liu K, et al. The status of pulmonary fibrosis in systemic sclerosis is associated with IRF5, STAT4, IRAK1, and CTGF polymorphisms. Rheumatol Int. 2017 Aug;37(8):1303–1310.
- Fang L, Liu M, Cai L. [Hederagenin inhibits proliferation and promotes apoptosis of cervical cancer CaSki cells by blocking STAT3 pathway]. Xi Bao Yu Fen Zi Mian Yi Xue Za Zhi. 2019 Feb;35(2):140–145.
- Kim GJ, Song DH, Yoo HS, et al. Hederagenin supplementation alleviates the pro-inflammatory and apoptotic response to alcohol in rats. Nutrients. 2017 6;9(1):Jan.
- Kim KH, Lee S, Lee H, et al. A standardized herbal extract PM014 ameliorates pulmonary fibrosis by suppressing the TGF-beta1 pathway. Sci Rep. 2018 Nov 15;8(1):16860.
- Zheng S, Wang Q, D’Souza V, et al. ResolvinD1 stimulates epithelial wound repair and inhibits TGF-beta-induced EMT whilst reducing fibroproliferation and collagen production. Lab Invest. 2018 Jan;98(1):130–140.