ABSTRACT
We previously found that equol, a metabolite of intestinal bacterial conversion from soy isoflavone daidzein, has female-specific anorectic effects. In the present study, we used seven-week-old female ovariectomized (OVX) Sprague Dawley rats to test the hypothesis that the anorectic effect of dietary daidzein may be attributed to delayed gastric emptying. Results suggest that dietary daidzein delays gastric emptying and that it has an anorectic effect with residual gastric contents, but not without gastric contents. Dietary equol significantly decreased daily food intake in the OVX rats without sleeve gastrectomy, but not in those with sleeve gastrectomy, suggesting that the accumulation of food in the stomach is required for the anorectic effect of equol to occur. These results support the hypothesis that the anorectic effect of dietary daidzein is attributed to delayed gastric emptying.
GRAPHICAL ABSTRACT
Dietary daidzein delays gastric emptying, and has an anorectic effect with residual gastric contents, but not without gastric contents.
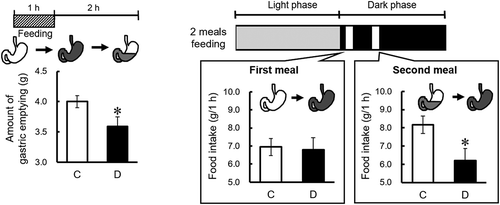
KEYWORDS:
Obesity is a serious global public health concern, contributing to multiple human diseases. Current strategies to treat obesity are unsatisfactory. Behavior modification and pharmacotherapy have been found to be effective only short term [Citation1,Citation2]. While surgical treatment induces satisfactory long-term weight loss, its application is very limited because of the substantial risks and complications involved [Citation3,Citation4]. Thus, the main clinical focus is the development of new approaches to induce satisfactory long-term weight loss.
Epidemiological research suggests regular dietary isoflavone intake has a protective effect against obesity and metabolic disorders in postmenopausal women [Citation5,Citation6]. In our previous study, daily intake or intubation of isoflavone aglycone-rich fermented soybean extract decreased food intake in both ovariectomized (OVX) and non-OVX female rats, but not in male rats, suggesting that the bitter taste of soy isoflavone was not the cause of the reduced food intake in female rats and that the observed reduction was a post-absorptive effect [Citation7]. Isoflavones are reported to have estrogenic activity [Citation8]. The anorectic effect of 17β-estradiol is more pronounced in male and OVX female rats than in non-OVX female rats, probably because of low endogenous estrogen levels [Citation7]. By contrast, dietary isoflavones decreased food intake in female rats with and without ovariectomy, but not in male rats [Citation7]. Thus, the female-specific anorectic effect of dietary isoflavones is probably not attributable to an estrogenic function.
Daidzein and genistein are the predominant aglycones of soy isoflavones. We found that dietary daidzein, but not dietary genistein, induces the anorectic effect by changing the gene expression of appetite-mediating neuropeptides in the hypothalamus, an important appetite control center that integrates peripheral hormone signals and interacts with other brain regions [Citation9,Citation10]. The information obtained by investigating mechanisms underlying the anorectic effect of dietary daidzein may be useful in the development of new approaches to induce satisfactory long-term suppression of appetite and weight loss.
Intestinal bacteria convert daidzein to equol in both humans and animals [Citation11]. Rachoń et al. reported that dietary equol reduces weight gain and intra-abdominal fat accumulation [Citation12]. We found that continuous intake of daidzein at the nutritional level induced the accumulation of equol in enterohepatic circulation to far higher levels than that of daidzein itself, and a small portion of equol escaped from enterohepatic circulation and it reached to systemic circulation [Citation13]. In addition, continuous intake of daidzein at nutritional doses for at least several days was required for equol to exert its anorectic effect [Citation13]. The accumulation of equol in enterohepatic circulation is possibly required for equol to exert its anorectic effect.
In our previous study, we used the thrice-daily feeding method to determine whether the daidzein-induced anorectic effect is directly mediated by gene expressions of appetite-mediating neuropeptides in the hypothalamus and small intestine [Citation10]. Dietary daidzein decreased food intake at the second meal session, but not at the first meal session. One possibility is that dietary daidzein may have an anorectic effect specifically with residual gastric contents, but not without gastric contents. According to clinical and basic studies, delayed gastric emptying may lead to early satiety and reduced food intake [Citation14–Citation17]. In experiment 1, to test this hypothesis, we first examined whether dietary daidzein delays gastric emptying by measuring the amount of gastric emptying in time restricted-fed rats. Next, in experiment 2, we examined whether dietary daidzein has an anorectic effect with residual gastric contents, but not without gastric contents. Lastly, in experiment 3, we examined whether delayed gastric emptying is responsible for the anorectic effect of equol using rats that cannot accumulate food in their stomachs as a result of sleeve gastrectomy [Citation18]. In the present study, we chose female OVX rats to examine the effects of daidzein because they are a good model for obesity caused by overeating [Citation7,Citation19].
Methods and materials
Animals and diet
All experiments were conducted on 6-week-old female Sprague Dawley rats, which were purchased from SLC (Hamamatsu, Japan) and raised in stainless wire mesh cages in a room controlled by a 12-h light-dark cycle at a constant temperature (23 ± 1°C) and humidity (55–65%). Bilateral ovariectomy was performed using the double dorsolateral method of Park et al. [Citation20] under inhalation anesthetic isoflurane 2.0–2.5% (FUJIFILM Wako Pure Chemical, Osaka, Japan). This experimental protocol was approved by the Laboratory Animal Care Committee of Ehime University, and the rats were maintained in accordance with the Guidance for the Care and Use of Laboratory Animals of Ehime University.
The rats were fed one of three diets based on AIN-76: control (C) diet, daidzein (D) diet, or equol (E) diet. The C diet contained (in g/kg): casein (New Zealand Dairy Board, Wellington, New Zealand), 200; corn oil (J-Oil Mills, Inc., Tokyo, Japan), 50; mineral mixture, 40; vitamin mixture, 10; sucrose (Nippon Beet Sugar Manufacturing, Tokyo, Japan), 350; L-cystine (Nacalai Tesque, Kyoto, Japan), 3; and α-corn starch (Nihon Shokuhin Kako, Tokyo, Japan), 347. Daidzein (>99% purity) and racemic equol (>99% purity) were purchased from LC Laboratories (MA, USA). The daidzein (D) diet included 0.3 g/kg of daidzein replacing α-corn starch in experiments 1 and 2, and the racemic equol (E) diet included 0.15 g/kg of equol replacing α-corn starch in experiment 3. In our previous study, serum and bile S-equol concentrations in rats fed S-equol diet (0.15 g/kg diet) were significantly higher than those in rats fed daidzein diet (0.15 g/kg diet) [Citation13]. In addition, we have preliminarily observed that food intake in OVX rats fed diet containing 50–150 mg/kg S-equol or 100–150 mg/kg R-equol was significantly lower than that in rats fed equol-free diet. Thus, we chose this dietary concentration of racemic equol in the present study. Food intake was measured after each feeding session. The food was replenished after measuring. The body weight of each rat was measured every morning.
Experimental protocol
Experiment 1
Five days after undergoing ovariectomies, rats were given the C diet for 3, 4, or 6 h per day from 8:30 to 11:30, 12:30, or 14:30, respectively. The time restricted-feeding schedule is shown in . Training on this schedule lasted 8 days. Rats (n= 8/group) were given either the C or D diet based on AIN-76. Daily changes in food intake were measured for 5 days. On day 6 of the test, the rats were decapitated, and the stomach contents were collected 2 hours after a one-hour feeding. The stomach contents were freeze-dried. The amount of gastric emptying was calculated by the following equation: amount of gastric emptying = (food intake for 1 h) − (dry gastric contents 2 h after one-hour feeding), as previously described [Citation21,Citation22].
Figure 1. Diagram for feeding schedules.
Time restricted-fed groups in experiment 1 were given meals from 8:30 to 11:30, 12:30, or 14:30 (3 h, 4 h, or 6 h, respectively). The twice-daily fed group in experiment 2 was given meals two times per day for 1 h each session (9:00‒10:00 and 12:00‒13:00). The black zone shows the dark phase without food availability; the white zones show food availability during the dark phase; the gray zone shows the light phase without food availability.
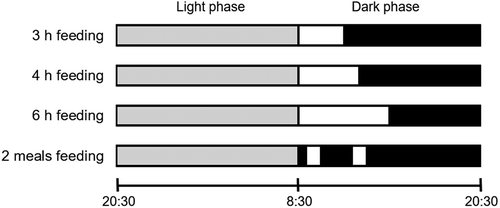
Experiment 2
Four days after undergoing ovariectomies, rats were given the C diet twice a day for 1 h each session (9:00‒10:00 and 12:00‒13:00). The feeding schedule is shown in . Training on this feeding schedule lasted 7 days. Rats (n = 10/group) were given either the C or D diet based on AIN-76. Daily changes in food intake were measured. On day 5 of the test, the rats were decapitated, and the stomach contents were collected 2 hours after a one-hour feeding. The stomach contents were freeze-dried. The amount of gastric emptying was calculated by the following equation: amount of gastric emptying = (food intake for 1 h) − (dry gastric contents 2 h after one-hour feeding).
Experiment 3
After the ovariectomies and 11 days of acclimatization, 40 rats underwent sleeve gastrectomy (SG). SG was performed as previously detailed [Citation23]. Rats fasted 24 h prior to surgery and were anesthetized with isoflurane 2.0–2.5% by inhalation (FUJIFILM Wako Pure Chemical, Osaka, Japan). A laparotomy incision was made in the abdominal wall. Related blood vessels, including the right and left gastroepiploic arteries and veins in the greater curvature, were ligated and transected. The esophageal arteries and veins were ligated. Loose gastric connections to the spleen and liver were released along the greater curvature. The stomach was isolated outside the abdominal cavity and placed on saline-moistened gauze pads. The gastric fundus and a large portion of the gastric body (50% of the total stomach) were resected. The residual stomach was stitched with a 6–0 nylon suture. The gastric sleeve was then reintegrated into the abdominal cavity. Finally, the abdominal wall was closed in layers.
After surgery, rats received single subcutaneous injections of Prazosin (10 mg/mL), a gastric acid secretion inhibitor, and intramuscular injections (0.05 mL/rat/day) of mycillin sol (meiji) for 3 days. Rats fasted for 24 h and were allowed access to 5% glucose solution until the food was returned 48 h after surgery. SG rats were excluded from the study if they lost more than 20% of body weight during a 10-day recovery period. Non-SG and SG rats were fed either the C or E diet with free access to water during the seven-day experimental period. Two groups of eight non-SG rats were fed either the C diet or the E diet, and two groups of 6 SG rats were fed either the C diet or the E diet. The food intake of each group was measured every morning. At the end of the experiments, rats were decapitated, and blood samples were collected to check equol concentrations.
Isoflavone level measurement
Sample preparation
Equol serum concentration was measured using the method of Janning et al. [Citation24]. Frozen samples of rat serum (100 μL) were mixed with 100 μL of hydrolysis buffer [0.1 mol/L sodium acetate, pH 5; 0.1% (w/v) ascorbic acid; 0.01% (w/v) EDTA], 8 μL of glucuronidase, and 4 μL of sulfatase. Reaction mixtures of serum samples were allowed to hydrolyze to glucuronide and sulfate metabolites at 37°C for at least 15 h. Subsequently, 10 μL of an internal standard (genistein, 5 μg/mL in dimethyl sulfoxide), 120 μL of water, 75 μL of ammonium acetate buffer (1 mmol/L, pH 7), and 85 μL of triethylammonium sulfate buffer (3 mol/L, pH 7) were added. The mixtures were heated to 60°C for 10 min to facilitate the dissociation of isoflavones from proteins and then centrifuged. Deproteinized samples were passed through 0.5-g Sep-Pak C18 cartridges (Nihon Waters, Tokyo, Japan) that had been previously washed with 5 mL of ammonium acetate buffer (10 mmol/L, pH 5) and 5 mL of water at room temperature. The absorbed isoflavones were eluted with 1.5 mL of methanol. The methanol effluent was evaporated to dryness under a gentle stream of nitrogen at 45°C and dissolved in 100 μL of methanol/1% aqueous acetic acid (40:60 v/v).
HPLC methods
The serum equol concentration was analyzed by high-performance liquid chromatography (HPLC) as detailed previously [Citation20]. A 5-μL aliquot of the sample was applied to a reverse-phase HPLC column (2.0 × 150 mm, CAPCELL PAK C18, particle size 5 μm; Shiseido, Tokyo, Japan). The mobile phase was a potassium phosphate buffer, 40% comprised a mixture of methanol and acetonitrile (3:2 v/v), at 40°C with a flow rate of 0.2 mL/min. Equol and genistein were detected using an electrochemical detector (Electrochemical Detector 3005; Shiseido, Tokyo, Japan) with a glassy carbon working electrode and an applied voltage of 800 mV.
Statistical analysis
All data are presented as the mean ± standard error (SEM). Statistical analysis was performed with an unpaired Student’s t-test. All statistical tests were performed using the IBM SPSS Statistics software package (SPSS Japan, Inc., an IBM company). Statistically, significant differences are defined at the 95% confidence interval.
Results and discussion
We first examined whether dietary daidzein delays gastric emptying by measuring the amount of gastric emptying in 3, 4, or 6 h time restricted-fed rats. We observed significant decreases in daily food intake after continuous daidzein intake for 3 or 4 days, regardless of the length of feeding time ()). The results of our previous study indicated that continuous intake of daidzein for at least several days induces accumulation of S-equol in enterohepatic circulation and was required to exert an anorectic effect, suggesting that accumulation of S-equol in enterohepatic circulation was needed to exert its anorectic effect [Citation13]. After 5 days of feeding, dietary daidzein did not alter food intake for 1 h after refeeding, regardless of the length of feeding time ()). A significant decrease in the amount of gastric emptying was observed only in the 3 h time restricted-fed group ()). It is speculated that the longer the fasting time is, the more appetite can be stimulated. Dietary daidzein did not affect the wet weight of gastric tissue (g/kg body weight) in 3, 4, or 6 h time restricted-fed rats (data not shown). These results indicate that dietary daidzein delays gastric emptying.
Figure 2. Effects of dietary daidzein (0.3 g/kg diet) on daily food intake (a-c), food intake for 1 h before sampling of gastric contents (d-f), and the amount of gastric emptying (g-i) in time restricted-fed ovariectomized rats (experiment 1).
On the final day of the experiment (day 6), the gastric content was collected 2 h after a one-hour feeding. Amount of gastric emptying was calculated using the following equation: amount of gastric emptying = (food intake for 1 h) − (dry gastric contents 2 h after a one-hour feeding). Each value represents the mean ± SEM (n= 8). Asterisks show significant difference relative to the control group, determined by an unpaired Student’s t-test (*P< 0.05; **P< 0.01). C, control group; D, daidzein group.
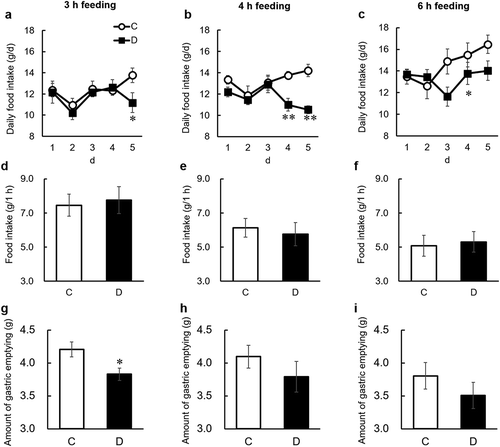
Next, we examined whether dietary daidzein has an anorectic effect with residual gastric contents, but not without gastric contents, in twice-daily fed rats. Dietary daidzein significantly decreased daily food intake at the second meal after continuous daidzein intake for 3 days but did not decrease food intake at the first meal during the experimental period ()). After 4 days of feeding, dietary daidzein significantly decreased the amount of gastric emptying 2 h after the end of the first meal session ()). These results suggest that dietary daidzein has an anorectic effect with residual gastric contents, but not without gastric contents. Therefore, it is likely that the anorectic effect of daidzein may be attributed to the delayed gastric emptying.
Figure 3. Effects of dietary daidzein (0.3 g/kg diet) on food intake during the first session (a), second session (b), and daily food intake (c) during the experimental period in twice-daily fed ovariectomized rats (experiment 2).
Each value represents the mean ± SEM (n= 10). Asterisks show significant difference relative to the control group, determined by an unpaired Student’s t-test (*P< 0.05). C, control group; D, daidzein group.
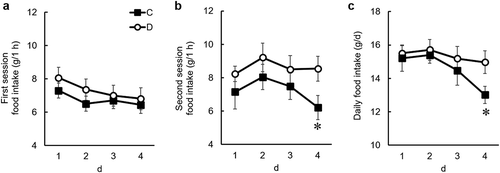
Figure 4. Diagram of the time schedule on the final day of the experiment (a), and effects of dietary daidzein (0.3 g/kg diet) on food intake for 1 h (b) and the amount of gastric emptying (c) on the final day of the experiment in twice-daily fed-ovariectomized rats (experiment 2).
On the final day (day 5) of the experiment, the gastric content was collected 2 h after a one-hour feeding. Amount of gastric emptying was calculated using the following equation: amount of gastric emptying = (food intake for 1 h) − (dry gastric contents 2 h after a one-hour feeding). Each value represents the mean ± SEM (n= 10). Asterisks show significant difference relative to the control group, determined by an unpaired Student’s t-test (*P< 0.05). C, control group; D, daidzein group.
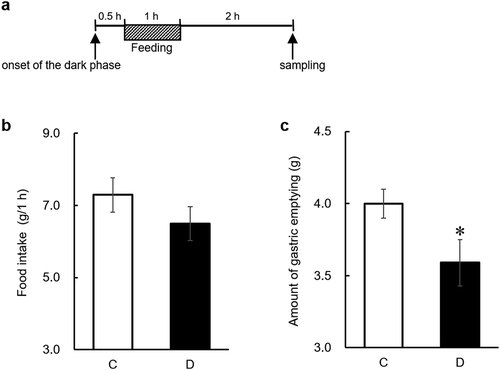
Lastly, we examined this hypothesis using SG rats that cannot accumulate food in their stomachs. Equol, but not daidzein, was administrated through diet supplementation because of a concern that SG may affect the intestinal bacterial conversion of daidzein to equol. Throughout the experimental period, daily food intake was significantly lower in the SG control group than the non-SG control group ()). However, daily food intake in the SG control group was decreased to the same extent as the twice-daily fed-control group in experiment 2 ()). Thus, we may be able to examine the anorectic effect of equol in SG and non-SG rats. Dietary equol significantly decreased daily food intake in non-SG rats but not in SG rats ()). The serum equol concentration was lower in SG rats than in non-SG rats (). In our previous study, serum equol concentration did not change between before and after meals in thrice-daily fed-intact female rats [Citation10]. In addition, we showed that continuous daidzein intake for several days induces the accumulation of S-equol in both systemic and enterohepatic circulations [Citation13]. Thus, the accumulation amount of equol in the body of SG rats may be less than that of non-SG rats, regardless of eating conditions. However, equol concentration in SG rats was increased to the same level in rats that had decreased food intake due to isoflavone intake in our previous study [Citation7]. It is suggested that the capacity to accumulate food in the stomach is required for the anorectic effect of equol to occur. While these results support the hypothesis that the anorectic effect of dietary daidzein may be attributed to delayed gastric emptying, the mechanisms involved are unclear. Further studies are needed to determine these mechanisms.
Table 1. Effect of dietary equol (0.15 g/kg diet) on serum isoflavone concentration in ovariectomized rats with and without sleeve gastrectomy.
Figure 5. Effect of dietary equol (0.15 g/kg diet) on daily food intake in ovariectomized rats with and without sleeve gastrectomy (SG) (experiment 3).
Each value represents the mean ± SEM (intact groups, n= 8; SG groups, n= 6). Asterisks show significant difference relative to the control group, determined by an unpaired Student’s t-test (*P< 0.05; **P< 0.01). C, control group; E, equol group; non-SG, intact groups; SG, sleeve gastrectomy operated groups.
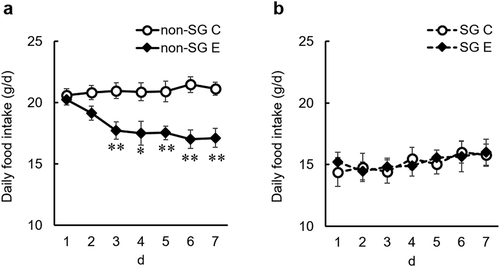
We previously examined the dynamics of hypothalamic and gastrointestinal appetite-mediated gene expression and the serum concentrations of leptin and insulin when daidzein feeding suppressed food intake in thrice-daily fed-intact female rats. The results showed that dietary daidzein decreased corticotrophin-releasing factor (CRF) mRNA in the hypothalamus and cholecystokinin (CCK) mRNA in the upper small intestine [Citation10]. It has been reported that central CRF inhibits gastric emptying [Citation22,Citation25]. Moran et al. proposed that circulating CCK has a satiety effect in its physiological range when it leads to gastric distention by restricting the flow of food from the stomach through the pylorus [Citation26]. Thus, it is possible that CRH and CCK contribute to delayed gastric emptying due to daidzein feeding. However, we have also observed that dietary daidzein significantly decreased food intake in both female Long-Evans Tokushima Otsuka and Otsuka Long-Evans Tokushima Fatty (CCK1R-deficient) rats, suggesting that CCK signaling is not essential for the anorectic effects of dietary daidzein [Citation27]. Whether an upregulation of CRF expression in the hypothalamus is essential for the inhibitory effects of dietary daidzein on food intake and gastric emptying need to be addressed in future studies.
We have shown that dietary isoflavones markedly increased serum equol concentration, but did not affect uterus weight in OVX rats [Citation7]. However, selective activation of estrogen receptor β (ERβ) by S-equol [Citation11] might be considered. It has been demonstrated that ERα-selective agonist propylpyrazoletriol (PPT), but not ERβ-selective agonist diarylpropionitrile (DPN), increases uterine wet weight and endometrial cell proliferation [Citation28]. On the other hand, both PPT and DPN inhibited the ACh-induced contraction of the single gastric smooth muscle cells, suggesting that both ERs may participate in inhibitory effects on gastric emptying [Citation29]. Further studies are needed to determine whether dietary daidzein delays gastric emptying via S-equol-induced ERβ activation in gastric smooth muscle.
In conclusion, it is suggested that the capacity to accumulate food in the stomach is required for the anorectic effect of equol to occur. Our findings suggest that dietary daidzein may be a potential alternative approach to the treatment of obesity.
Author contributions
Sudhashree Adhikari, Keshab Bhattarai, Yasuhiro Abe, Mayu Kira, Mina Fujitani, Tomihiro Miyada, and Taro Kishida designed the experiments. Yasuhiro Abe and Mayu Kira carried out the experiments. Tomihiro Miyada assisted in the experiments. Sudhashree Adhikari and Keshab Bhattarai analyzed the data, interpreted the results, prepared figures, and wrote the manuscript. Mina Fujitani and Taro Kishida contributed to analysis and interpretation of data and assisted in the preparation of the manuscript.
Acknowledgments
This work was supported by Japan Society for the Promotion of Science (Grant Number 16K07738) and by a grant from the Fuji Foundation for Protein Research. It was also supported by the Rotary Yoneyama Memorial Foundation scholarship and the Ministry of Education, Culture, Sports, Science, and Technology, Japan, under the Monbukagakusho scholarship.
Disclosure statement
No potential conflict of interest was reported by the authors.
Data availability statement
The data described in this article are openly available in the Open Science Framework at DOI:10.17605/OSF.IO/TPA6U.
Additional information
Funding
References
- Dickey RA, Bartuska DG, Bray GW, et al. AACE/ACE position statement on the prevention, diagnosis, and treatment of obesity. Endocr Pract. 1998;4:297–330.
- Bray GA, Greenway FL. Current and potential drugs for treatment of obesity. Endocr Rev. 1999;20(6):805–875.
- Brolin RL, Robertson LB, Kenler HA, et al. Weight loss and dietary intake after vertical banded gastroplasty and Roux-en-Y gastric bypass. Ann Surg. 1994;220(6):782–790.
- Sagar PM. Surgical treatment of morbid obesity. Br J Surg. 1995;82(6):732–739.
- Goodman-Gruen D, Kritz-Silverstein D. Usual dietary isoflavone intake is associated with cardiovascular disease risk factors in postmenopausal women. J Nutr. 2001;131(4):1202–1206.
- Goodman-Gruen D, Kritz-Silverstein D. Usual dietary isoflavone intake and body composition in postmenopausal women. Menopause. 2003;10(5):427–432.
- Kishida T, Mizushige T, Ohtsu Y, et al. Dietary soy isoflavone–aglycone lowers food intake in female rats with and without ovariectomy. Obesity (Silver Spring). 2008;16(2):290–297.
- Messina MJ. Legumes and soybeans: overview of their nutritional profiles and health effects. Am J Clin Nutr. 1999;70:439S-450S.
- Bhattarai K, Adhikari S, Fujitani M, et al. Dietary daidzein, but not genistein, has a hypocholesterolemic effect in non-ovariectomized and ovariectomized female Sprague-Dawley rats on a cholesterol-free diet. Biosci Biotechnol Biochem. 2017;81(9):1805–1813.
- Fujitani M, Mizushige T, Bhattarai K, et al. Dynamics of appetite-mediated gene expression in daidzein-fed female rats in the meal-feeding method. Biosci Biotechnol Biochem. 2015;79(8):1342–1349.
- Setchell KD, Clerici C, Lephart ED, et al. S-equol, a potent ligand for estrogen receptor beta, is the exclusive enantiomeric form of the soy isoflavone metabolite produced by human intestinal bacterial flora. Am J Clin Nutr. 2005;81(5):1072–1079.
- Rachoń D, Vortherms T, Seidlová-Wuttke D, et al. Effects of dietary equol on body weight gain, intra-abdominal fat accumulation, plasma lipids, and glucose tolerance in ovariectomized Sprague-Dawley rats. Menopause. 2007;14(5):925–932.
- Fujitani M, Mizushige T, Bhattarai K, et al. Dietary daidzein induces accumulation of S-equol in enterohepatic circulation to far higher levels than that of daidzein in female rats with and without ovariectomy. Biomed Res. 2019;40(3):97–105.
- Chen J. Mechanisms of action of the implantable gastric stimulator for obesity. Obes Surg. 2004;14(Suppl 1):S28–32.
- Oesch S, Rüegg C, Fischer B, et al. Effect of gastric distension prior to eating on food intake and feelings of satiety in humans. Physiol Behav. 2006;87(5):903–910.
- Phillips RJ, Powley TL. Gastric volume rather than nutrient content inhibits food intake. Am J Physiol. 1996;271(3 Pt 2):R766–R769.
- Rolls BJ, Castellanos VH, Halford JC, et al. Volume of food consumed affects satiety in men. Am J Clin Nutr. 1998;67(6):1170–1177.
- Braghetto I, Davanzo C, Korn O, et al. Scintigraphic evaluation of gastric emptying in obese patients submitted to sleeve gastrectomy compared to normal subjects. Obes Surg. 2009;19(11):1515–1521.
- Fujitani M, Kishida T, Shimizu E, et al. Difructose anhydride III decreases body fat as a low-energy substitute or by decreasing energy intake in non-ovariectomized and ovariectomized female rats. Biosci Biotechnol Biochem. 2017;81(7):1425–1432.
- Park SB, Lee YJ, Chung CK. Bone mineral density changes after ovariectomy in rats as an osteopenic model: stepwise description of double dorso-lateral approach. J Korean Neurosurg Soc. 2010;48(4):309–312.
- Wang L, Martínez V, Rivier JE, et al. Peripheral urocortin inhibits gastric emptying and food intake in mice: differential role of CRF receptor 2. Am J Physiol Regul Integr Comp Physiol. 2001;281(5):R1401–R1410.
- Nakade Y, Tsukamoto K, Pappas TN, et al. Central glucagon like peptide-1 delays solid gastric emptying via central CRF and peripheral sympathetic pathway in rats. Brain Res. 2006;1111(1):117–121.
- Miyada T, Nakajima A. Surgery of the digestive system in the rat 3 ileostomy and gastrectomy. J Jpn Assoc Dietary Fiber Res. 17(2):87–93.
- Janning P, Schuhmacher US, Upmeier A, et al. Toxicokinetics of the phytoestrogen daidzein in female DA/Han rats. Arch Toxicol. 2000;74(8):421–430.
- Martinez V, Barquist E, Rivier J, et al. Central CRF inhibits gastric emptying of a nutrient solid meal in rats: the role of CRF2 receptors. Am J Physiol. 1998;274(5):G965–G970.
- Moran TH, McHugh PR. Cholecystokinin suppresses food intake by inhibiting gastric emptying. Am J Physiol. 1982;242(5):R491–R497.
- Fujitani M, Mizushige T, Bhattarai K, et al. The daidzein- and estradiol- induced anorectic action in CCK or leptin receptor deficiency rats. Biosci Biotechnol Biochem. 2015;79(7):1164–1171.
- Song X, Pan ZZ. Estrogen receptor-beta agonist diarylpropionitrile counteracts the estrogenic activity of estrogen receptor-alpha agonist propylpyrazole-triol in the mammary gland of ovariectomized Sprague Dawley rats. J Steroid Biochem Mol Biol. 2012;130(1–2):26–35.
- Al-Shboul OA, Nazzal MS, Mustafa AG, et al. Estrogen relaxes gastric muscle cells via a nitric oxide- and cyclic guanosine monophosphate-dependent mechanism: a sex-associated differential effect. Exp Ther Med. 2018;16(3):1685–1692.