ABSTRACT
Antibacterial activities against Staphylococcus aureus and Bacillus subtilis were found in an ethanol fraction of tempe, an Indonesian fermented soybean produced using Rhizopus oligosporus. The ethanol fraction contained free fatty acids, monoglycerides, and fatty acid ethyl esters. Among these substances, linoleic acid and α-linolenic acid exhibited antibacterial activities against S. aureus and B. subtilis, whereas 1-monolinolenin and 2-monolinolenin exhibited antibacterial activity against B. subtilis. The other free fatty acids, 1-monoolein, monolinoleins, ethyl linoleate, and ethyl linolenate did not exhibit bactericidal activities. These results revealed that R. oligosporus produced the long-chain polyunsaturated fatty acids and monolinolenins as antibacterial substances against the Gram-positive bacteria during the fungal growth and fermentation of heat-processed soybean.
GRAPHICAL ABSTRACT
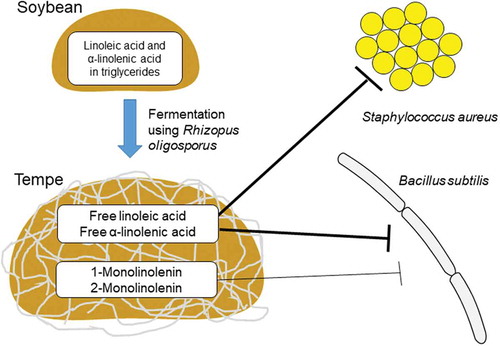
Tempe contains linoleic and α-linolenic acids, which are bactericidal against S. aureus and B. subtilis, and monolinolenins, which are bactericidal against B. subtilis.
Tempe is a heat-processed fermented soybean food, which includes many beneficial substances for human health [Citation1]. The fungal fermentation process causes many changes in nutrition, texture, and taste of soybean. Many enzymes were secreted during the fermentation process to break down macromolecules into smaller molecules that have higher bioavailability. Preceded researches have reported the nutrition, anti-hypertension, anti-diabetic, antioxidant, antidiarrheal, and antibacterial contents found in tempe [Citation2–Citation5]. Additionally, the tempe-making process can remove antinutrients such as antitrypsin, phytic acid, and oligosaccharides from soybean [Citation5]. The tempe extracts protect human and animal intestinal cells from enterotoxigenic Escherichia coli infection [Citation6,Citation7].
From a food preservability viewpoint, antibacterial activities of tempe extract have been investigated. The water extract from tempe produced using Rhizopus microsporus exhibits antibacterial activity against Bacillus cereus [Citation8]. Nullification of the bactericidal effect in the extract after heat or protease treatment indicates the proteinaceous nature of antibacterial substances included in the extract. In addition, the fractions extracted with ethanol or ethyl acetate from tempe produced using Rhizopus oligosporus inhibit the growth of Bacillus subtilis and Staphylococcus aureus [Citation9]. The tempe starter R. oligosporus can produce the 5.5-kDa peptide in submerged cultivation broth, which inhibits the growth of Gram-positive bacteria including B. subtilis natto, S. aureus, and Streptococcus cremoris [Citation10]. The ethanol fraction of tempe produced using R. oligosporus inhibits the growth of S. aureus and the antibacterial activity is dependent on the fungal growth in heat-processed soybean [Citation11]. Therefore, it should be hypothesized that antibacterial substances included in the ethanol fraction of tempe have the proteinaceous nature as reported in the water extract from tempe produced using R. microsporus and R. oligosporus [Citation8,Citation10].
In this study, purification and characterization of antibacterial substances included in the ethanol fraction of tempe produced using R. oligosporus were performed in order to clarify their chemical structures and bactericidal activities. A mechanism of their production during fermentation and availability as food preservatives in tempe were discussed.
Materials and methods
Microorganisms and reagents used
The spore suspension of R. oligosporus (a starter of ragi tempe, Raprima, Bandung, Indonesia) and tempe were prepared as described previously [Citation11]. S. aureus NCTC50581 (National Collection of Type Cultures, London, UK) was cultured in Bacto tryptic soy broth (Becton, Dickinson and Company, Sparks, MD). B. subtilis ATCC6633 (American Type Culture Collection, Manassas, VA, USA) was cultured at 37ºC in Luria-Bertani medium (10 g/L tryptone, 5 g/L yeast extract, 10 g/L NaCl, pH 7.2). Oleic acid, linoleic acid, and α-linolenic acid were purchased from Sigma‐Aldrich (Tokyo, Japan). Palmitic acid, stearic acid, and 1-monoolein were purchased from Tokyo Chemical Industry (Tokyo, Japan). 1-Monolinolein was purchased from AccuStandard (New Haven, CT, USA).
Preparation of the ethanol fractions from heat-processed soybean and tempe
Soybean grains treated with steam pressure before or after fermentation were lyophilized and finely ground by a pestle and mortar. The ground powder was suspended in 2.5 mL ethanol/g dry wt. The suspension was vigorously agitated at 4°C for 8 h and centrifuged at 13,000 × g for 10 min. The supernatant was used as the ethanol fraction from soybean or tempe and stored at −20°C.
Characterization of an antibacterial substance in the ethanol fraction of tempe
The ethanol fractions were treated with 50, 100, 250, and 500 µg/mL trypsin, and incubated for 4 h and overnight. Conical tubes containing the ethanol fraction were placed in boiling water for 5, 30, 45, and 60 min. The ethanol fractions after trypsin or boiling treatment were added to S. aureus and B. subtilis cultures after measuring 1.0% (vol/vol) of the cultures. Bacterial growths were monitored at absorbance 600 nm.
Analyses by liquid chromatography (LC)
Test samples were filtrated using HLC disk 3, 0.45 μm pore size (Kanto Chemical, Tokyo, Japan). LC-ultraviolet (UV) absorption was performed using an LC system, which was composed of 1525 binary pump, 717 plus autosampler, and 2996 photodiode array detector (Nihon Waters, Tokyo, Japan), equipped with InertSustain C18 column (5 μm, 4.6 × 250 mm, GL Sciences, Tokyo, Japan). Substances in the test samples were eluted at a flow rate of 1.0 mL/min with a gradient of water containing acetic acid (pH 3.5) and methanol from 50% to 100% (vol/vol) methanol for 40 min and 100% methanol from 40 to 60 min. LC-mass spectrometry (MS) was performed using an LC system, which was composed of LC-20AD XR pumps, SIL-20A XR autosampler, CTO-20AC column oven, CBM-20A communication bus module, and LCMS-2020 mass spectrometer (Shimadzu, Kyoto, Japan), as performed in LC-UV. Negative ions formed through atmospheric-pressure chemical ionization were detected.
Analysis by gas chromatography (GC)
The acid-catalyzed methyl esterification was performed as described previously [Citation12]. One milliliter esterification solution (methanol-chloroform-sulfuric acid, 10:10:0.3 (vol/vol/vol)) was added to fatty acid or an evaporated LC-UV fraction in a screw-capped test tube with poly(1,1,2,2-tetrafluoroethylene) (PTFE) liner. The test tube was heated in a hole of aluminum block placed on a hot stirrer at 94°C, 200 rpm for 30 min. The reaction mixture was cooled down to room temperature, and 1 mL of water containing 0.9% (wt/vol) NaCl was added. The test tube was vigorously agitated with a vortex mixer and centrifuged at 1,700 × g for 10 min. The organic phase was collected using a Pasteur pipette, and 1 µL of the organic phase was injected to GC-MS (Focus GC-DSQII, Thermo Fisher Scientific, Yokohama, Japan), which equipped a capillary column TC-70 (0.25 mm I.D. × 60 m, GL Science, Tokyo, Japan). Helium (>99.99995%) was used as a carrier gas at a flow rate of 2.0 mL/min, and thermal conditions of column oven were 75°C for 2 min, 25°C/min to 180°C, 6°C/min to 240°C, and 240°C for 2 min.
Synthesis of monoglycerides and ethyl esters
Monoglycerides were synthesized using p-toluenesulfonic acid [Citation13]. Fatty acid and glycerol were mixed at an equal molar ratio in a screwcap test tube with PTFE liner, and p-toluenesulfonic acid was added after measuring 5 wt % of the mixed glycerol. The test tube was heated in a hole of aluminum block placed on a hot stirrer at 112°C, 200 rpm for 5 h. Monoglycerides were dissolved in an adequate volume of chloroform. Ethyl esters were synthesized as described in the method of acid-catalyzed methyl esterification with an exception of the use of ethanol instead of methanol. Monoglycerides or ethyl esters dissolved in chloroform were injected multiple times to LC-UV for purification. After collecting fractions, the eluting solvent was evaporated, and the dried residues were dissolved in adequate volumes of ethanol after weighing so that concentrations of monoglycerides and ethyl esters were adjusted to 100 mg/mL.
Determination of minimum bactericidal concentrations
Overnight cultures of S. aureus and B. subtilis were inoculated at one-hundredth volume of liquid medium. The commercially available fatty acids and 1-monoolein and the synthesized and purified monolinoleins, monolinolenins, 2-monolein, and ethyl esters were used as test compounds. The test compounds dissolved in ethanol were added within one-hundredth of culture volume or less at the concentrations indicated, and the bacterial cells were exposed to the test compounds with 150 rpm shaking at 37°C for 18 h. After the exposure to test compounds, 50 µL of cell suspension was spread on plate medium in order to evaluate bacterial viability.
Results and discussion
Biochemical features of antibacterial substance in the ethanol fraction
The ethanol fraction from tempe repressed growths of B. subtilis and S. aureus, whereas their growths were not affected by an equal amount of ethanol. The growth-repressing activities still remained in the ethanol fraction after the overnight trypsin treatment or boiling in water for 60 min (Supplemental Figure. 1). A flow-through fraction in ultrafiltration inhibited the bacterial growths (Supplemental Figure 2), indicating that a molecular weight of antibacterial substance might be lower than its molecular weight cutoff (5 kDa). The peptide with molecular weight of 5.5 kDa [Citation10] was not observed in sodium dodecyl sulfate-polyacrylamide gel electrophoresis of the ethanol fraction (Supplemental Figure 3). These results suggest that an antibacterial substance contained in the ethanol fraction is not the peptide with 5.5 kDa molecular size reported in R. oligosporus.
Analysis of substances in the ethanol fractions
The ethanol fractions collected from heat-treated soybeans before and after fermentation were analyzed by the reverse phase LC. Major peaks numbered from 1 to 8 were observed in a chromatogram of the fraction collected from soybean after fermentation, whereas only small peaks in the retention time from peak 1 to peak 8 were observed in a chromatogram of the fraction collected from soybean before fermentation (). It has been shown that the antibacterial activity against S. aureus increased significantly after fermentation for 48 and 72 h [Citation11]. Therefore, it is possible that the antibacterial activities against B. subtilis and S. aureus originate from the substances eluted at the retention times of these peaks.
Identification of substances in the ethanol fraction after fermentation
Substances in the LC-UV peak fractions were methyl-esterified and analyzed by GC-MS. Two major peaks emerged around 12.7 min in the chromatograms for peak 1, 2, 5, and 7 fractions and 12.1 min in the chromatograms for peak 3, 4, 6, and 8 fractions, respectively (). Mass spectra of the peaks emerged at 12.7 and 12.1 min corresponded to those of methyl linolenate and methyl linoleate, whose molecular ions were 292 and 294 m/z, respectively. A mass spectrum of the peak at 12.7 min contained ions of m/z = 236 and m/z = 108 as observed in the mass spectrum of methyl α-linolenate [Citation14], while it did not contain ions of m/z = 194 and m/z = 150, which were specific ions for the mass spectrum of methyl γ-linolenate [Citation15]. The mass spectrum of the peak at 12.7 min was identified as that of methyl α-linolenate. Therefore, it is suggested that α-linolenic acyl group and linoleic acyl group are included in the structures of substances in peak 1, 2, 5, and 7 fractions and peak 3, 4, 6, and 8 fractions, respectively. The ethanol fraction of tempe was analyzed by GC-MS without the esterification process. Peaks for ethyl esters of palmitic acid, stearic acid, oleic acid, linoleic acid, and α-linolenic acid, together with a small peak for methyl linoleate, emerged in a chromatograph of the fraction collected from soybean after fermentation (). As the fatty acid composition in soybean oil [Citation16] corresponds to the fatty acyl composition of the ethyl esters, these esters in ethanol fraction might originate from fatty acids bound in triglycerides of soybean oil. Then, LC-UV peak fractions were analyzed with GC-MS without the esterification process. Chromatograms of peak 7 and peak 8 fractions included major peaks at 12.9 and 12.3 min, respectively, whose mass spectra corresponded to those of ethyl linolenate and ethyl linoleate (). The result indicates that peak 7 and peak 8 fractions contain ethyl linolenate and ethyl linoleate, respectively.
Figure 2. GC-MS chromatograms of LC-UV peak fractions after methyl esterification.
The peak numbers of the fraction are shown at the right side. Arrows indicate the positions where main methyl esters were eluted.
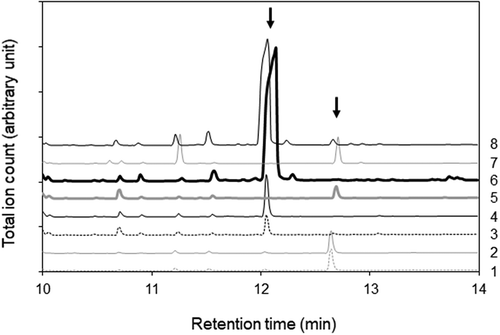
Figure 3. GC-MS chromatograms of the ethanol fractions and LC-UV peak fractions without methyl esterification.
The peak numbers of the fraction are shown at the right side.
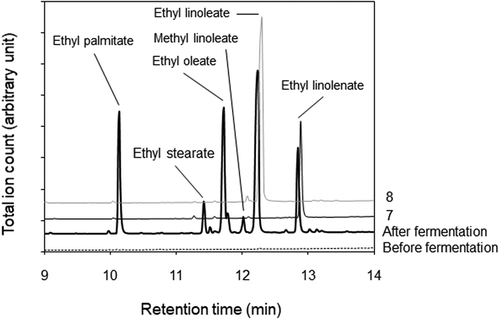
An LC-MS chromatogram included peaks corresponding to the LC-UV peaks 1 to 6 (). However, the peaks 7 and 8 were not observed probably because hydrophobicity of the ester bond might make ethyl linolenate and ethyl linoleate difficult to form their molecule-related ions. In addition, peaks a to e were observed in the LC-MS chromatogram. As peaks 3–4 and peaks a-b exhibited the same mass spectra as those of commercially available 1-monolinolein and 1-monoolein, respectively (). Molecule-related ions were detected as acetic acid adducts of deprotonated monolinolein and monoolein. Then, monolinoleins were synthesized from linoleic acid and glycerol. In a chromatogram of the synthesized monolinoleins, a peak of 1-monolinolein was higher than that of 2-monolinolein, because esterification in two hydroxyl groups of glycerol results in the synthesis of 1-monolinolein while esterification in one hydroxyl group of glycerol results in the synthesis of 2-monolinolein. Monooleins and monolinolenins were also synthesized from oleic acid or α-linolenic acid and glycerol, respectively. The synthesized monooleins, monolinoleins, and monolinolenins were purified by LC-UV while defining peaks with the larger area as peaks of 1-monoglycerides and peaks with the smaller area as peaks of 2-monoglycerides. The LC-MS peaks 1–2 exhibited the same mass spectra as those of the synthesized monolinolenins (). A molecule-related ion was also detected as an acetic acid adduct of deprotonated monolinolenin. From the retention times of synthesized and commercially available compounds, main substances included in the LC-UV and LC-MS peaks were identified as follows: peak 1, 2-monolinolenin; peak 2, 1-monolinolenin; peak 3, 2-monolinolein; peak 4, 1-monolinolein; peak 5, α-linolenic acid; peak a, 2-monoolein; peak b, 1-monoolein; peak 6, linoleic acid; peak c, palmitic acid; peak d, oleic acid; peak e, stearic acid. It was difficult in LC-UV to detect the substances included in peaks a to e because of low extinction coefficients at 210 nm in an acyl group with non- or single-double bond within their structures.
Minimum bactericidal concentrations of antibacterial substances
Minimum bactericidal concentrations of fatty acids, monoglycerides, and ethyl esters detected in the ethanol fraction were evaluated. Linoleic acid (peak 5) and α-linolenic acid (peak 6) exhibited bactericidal activities against S. aureus and B. subtilis while palmitic acid (peak c), oleic acid (peak d), and stearic acid (peak e) did not exhibit the activities at 1,000 µg/mL (). Monolinolenins (peaks 1–2) exhibited an antibiotic activity against B. subtilis, while no activities were found in monolinoleins (peaks 3–4), monooleins (peaks a–b), ethyl linolenate (peak 7), and ethyl linoleate (peak 8). These results indicate that formation of an ester bond in linoleic acid and α-linolenic acid weakens their bactericidal activities. The difference in the antibiotic activities between monoglycerides and ethyl esters may be related to the difference in hydrophilicity around the carbonyl group. The mechanisms of antibacterial fatty acids in bactericidal activities are well documented [Citation17]. It might correlate to the amphipathic property of fatty acids and the prime target on bacteria is the cell membrane. At low concentrations, antibacterial fatty acids cause pores in the cell membrane whereas at high concentrations, they solubilize cell membrane so that cell metabolites leak out and cells lyse. Medium- and long-chain unsaturated free fatty acids tend to be more active against Gram-positive bacteria than Gram-negatives [Citation17]. The previous studies have demonstrated the biological effects of linoleic acid and α-linolenic acid on inhibiting the growth of various Gram-positive bacteria [Citation18,Citation19].
Table 1. Evaluation of bactericidal activities of fatty acids, monoglycerides, and ethyl esters and concentrations of linoleic acid, α-linolenic acid, and monolinolenins in tempe.
Contribution of linoleic acid and α-linolenic acid to the antibacterial activities in tempe
During tempe fermentation, R. oligosporus produces variety of enzymes such as carbohydrase, lipase, protease, and phytase [Citation20]. Lipase is a kind of lipolytic enzymes that cleave fatty acid from lipid head groups by hydrolysis [Citation17]. In this study, the composition of fatty acids and acyl groups in ethyl esters corresponded to the fatty acid composition in soybean oil [Citation16]. Therefore, it is likely that linoleic acid, α-linolenic acid, and monolinolenins might be converted from triglycerides in soybean oil by R. oligosporus lipase during fermentation. Bacillus spp. are involved in deteriorating of large varieties of food products such as milk, dairy products, meat products, bakery products, and fermented soybeans [Citation21]. For the food industries, the spoilage of products with spore-forming bacilli is the main concern in extending their shelf-life. The concentrations of linoleic acid and α-linolenic acid were higher than those of monolinolenins, and three orders of magnitude higher than their minimum bactericidal concentrations against B. subtilis (). Furthermore, monolinolenins were included in tempe at the concentration around their minimum bactericidal concentrations. The result suggests that linoleic acid and α-linolenic acid might mainly contribute to the intensive antibacterial activity especially to B. subtilis in tempe. This study has first demonstrated that the antibacterial-free fatty acids and monoglycerides, whose concentrations are especially effective against B. subtilis, are contained in tempe produced using R. oligosporus.
Author Contribution
DK and IM were responsible for the organization of research and design of experiments. DK, MW, MM, XX, and IM were responsible for the identification of compounds. DK, MW, MM, KY, and IM were responsible for the syntheses of compounds and their antibiotic activity measurements. All authors contributed to the writing of the final manuscript.
Supplemental_data_ver.2.pdf
Download PDF (84.3 KB)Disclosure statement
No potential conflict of interest was reported by the authors.
Supplementary material
Supplemental data for this article can be accessed here.
References
- Astuti M, Meliala A, Dalais FS, et al. Tempe, a nutritious and healthy food from Indonesia. Asia Pac J Clin Nutr. 2000 Dec;9(4):322–325. PubMed PMID: 24394511; eng.
- Esaki H, Onozaki H, Kawakishi S, et al. New antioxidant isolated from tempeh. J Agric Food Chem. 1996 Jan 01;44(3):696–700.
- Gyoergy P, Murata K, Ikehata H. Antioxidants isolated from fermented soybeans (tempeh). Nature. 1964 Aug 22;203:870–872. PubMed PMID: 14204076; eng.
- Karyadi D, Lukito W. Beneficial effects of tempeh in disease prevention and treatment. Nutr Rev. 1996;54(11):S94–S98.
- Nout MJR, Kiers JL. Tempe fermentation, innovation and functionality: update into the third millenium. J Appl Microbiol. 2005;98(4):789–805.
- Roubos-van den Hil PJ, Nout MJ, Beumer RR, et al. Fermented soya bean (tempe) extracts reduce adhesion of enterotoxigenic Escherichia coli to intestinal epithelial cells. J Appl Microbiol. 2009 Mar;106(3):1013–1021. PubMed PMID: 19191956; eng.
- Roubos-van den Hil PJ, Schols HA, Nout MJ, et al. First characterization of bioactive components in soybean tempe that protect human and animal intestinal cells against enterotoxigenic Escherichia coli (ETEC) infection. J Agric Food Chem. 2010 Jul 14;58(13):7649–7656. PubMed PMID: 20550210; eng.
- Roubos‐van PJ, Dalmas E, Nout MR, et al. Soya bean tempe extracts show antibacterial activity against Bacillus cereus cells and spores. J Appl Microbiol. 2010;109(1):137–145.
- Mambang D, Suryanto D. Antibacterial activity of tempe extracts on Bacillus subtilis and Staphylococcus aureus. Jurnal Teknologi dan Industri Pangan. 2014;25(1):115–118.
- Kobayasi S, Okazaki N, Koseki T. Purification and characterization of an antibiotic substance produced from Rhizopus oligosporus IFO 8631. Biosci Biotechnol Biochem. 1992 Jan;56(1):94–98. PubMed PMID: 1368137; eng.
- Kusumah D, Kabuyama Y, Maeda I. Promotion of fungal growth, antibacterial and antioxidative activities in tempe produced with soybeans thermally treated using steam pressure. Food Sci Technol Res. 2018;24(3):395–402.
- Seto Y, Kang J, Ming L, et al. Genetic replacement of tesB with PTE1 affects chain-length proportions of 3-hydroxyalkanoic acids produced through beta-oxidation of oleic acid in Escherichia coli. J Biosci Bioeng. 2010 Oct;110(4):392–396. PubMed PMID: 20547355; eng.
- Bossaert WD, De Vos DE, Van Rhijn WM, et al. Mesoporous sulfonic acids as selective heterogeneous catalysts for the synthesis of monoglycerides. J Catal. 1999;182(1):156–164.
- Hallgren B, Ryhage R, Stenhagen E. The mass spectra of methyl oleate, methyl linoleate, and methyl linolenate. Acta Chem Scand. 1959;13(4):845–847.
- Holman RT, Rahm JJ. Analysis and characterization of polyunsaturated fatty acids. Prog Chem Fats Other Lipids. 1971;9:13–90.
- Graef G, Fehr W, Miller L, et al. Inheritance of fatty acid composition in a soybean mutant with low linolenic acid. Crop Sci. 1988;28(1):55–58.
- Desbois AP, Smith VJ. Antibacterial free fatty acids: activities, mechanisms of action and biotechnological potential. Appl Microbiol Biotechnol. 2010 Feb;85(6):1629–1642. PubMed PMID: 19956944; eng.
- Feldlaufer M,F, Knox D,A, Lusby W,R, et al. Antimicrobial activity of fatty acids against Bacillus larvae, the causative agent of American foulbrood disease. Apidologie. 1993;24(2):95–99.
- Kabara JJ, Swieczkowski DM, Conley AJ, et al. Fatty acids and derivatives as antimicrobial agents. Antimicrob Agents Chemother. 1972 Jul;2(1):23–28. PubMed PMID: 4670656; PubMed Central PMCID: PMCPMC444260. eng.
- de Reu JC, Ramdaras D, Rombouts FM, et al. Changes in soya bean lipids during tempe fermentation. Food Chem. 1994 01;50(2):171–175. Jan.
- Abdou AM, Higashiguchi S, Aboueleinin AM, et al. Antimicrobial peptides derived from hen egg lysozyme with inhibitory effect against Bacillus species. Food Control. 2007;18(2):173–178.