ABSTRACT
Cerebral ischemia reperfusion (I/R) is a therapeutic strategy for ischemia; however, it usually causes injury by the aspect of inflammation and neuron apoptosis. This investigation aims to investigate the protective effects of phytic acid (IP6) for cerebral I/R injury in vitro. PC-12 cells under Oxygen and glucose deprivation/reperfusion (OGD/R) were performed to mimic cerebral I/R. IP6 was pretreated before PC-12 cells under OGD/R treatment. The data showed that IP6 activated the expression of sestrin2 in OGD/R injured PC-12 cells. IP6 inhibited OGD/R induced inflammation, oxidative stress, and apoptosis by activating sestrin2. Besides, p38 MAPK may mediate the effects of sestrin2 activated by IP6. Therefore, IP6 can be a potential drug to prevent neurological damage in cerebral I/R injury.
Graphical abstract
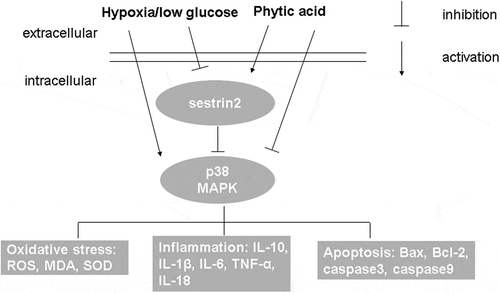
Phytic acid exerts protective effects in cerebral I/R injury by activating sestrin2.
Cerebral ischemia results in neurological disability to cause deaths year by year [Citation1]. Reperfusion is one of the ways to restore blood; however, refusion after cerebral ischemia can usually induce cerebral edema, brain hemorrhage, and neuronal death [Citation2]. Complex mechanisms of cerebral ischemia-reperfusion (I/R) injury include free radicals induced inflammation, disturbance of energy metabolism disorder in brain tissues, and cytotoxicity of NO [Citation3]. The most common injuries caused by stroke are loss of movement or sensation. To help patients recovering quickly and adapt to daily activities, rehabilitation therapies are involved including medication and early physical training [Citation4]. Constraint-induced Movement Therapy is usually performed to protect functional recovery after post-stroke [Citation5]. Previous studies indicate that neuroprotective strategies in rehabilitation of stroke are of great importance [Citation6].
Sestrins (SESN), consisting of three family members (SESN1, SESN2 and SESN3), are stress-inducible regulators, which enable the cells to accommodate the stress stimuli. Particularly, Sestrins exert cytoprotective effects by reducing the levels of reactive oxygen species (ROS) [Citation7]. SESN2 levels can be induced by hypoxia conditions, and other inducers such as DNA damage and oxidative stress can also trigger [Citation8]. Reoxygenation after hypoxia increases the oxidative stress and subsequently leads to cell death via DNA damage and lipid peroxidation [Citation9]. SESN2 knocked-down has been proved to exacerbate cerebral I/R injury by regulating mitochondrial dysfunction [Citation10]. Therefore, it can be a potential therapy target for cerebral ischemia/reperfusion injury.
Phytic acid, also known as inositol hexaphosphate or IP6, is abundant in various herbal plants seeds where it is the main storage form of phosphorus [Citation11]. IP6 has been investigated to treat diseases such as diabetes, kidney stone, cancer, and so on [Citation12–Citation14]. It has been reported the anti-oxidant effects to exert neuroprotective and lipid-lowering activity serving as a natural molecule to prevent disease [Citation15,Citation16]. IP6 as an iron chelator to regulate cell apoptosis in Parkinson’s disease [Citation17]. Our investigation found that IP6 enhances sestrin2 expression in OGD/R triggered cerebral I/R injury. We supposed that IP6 would suppress cerebral I/R injury by activating sestrin2.
Materials and methods
Cell culture and OGD/R models
Rat PC-12 (pheochromocytoma) cell line (Institute of Basic Medical Sciences at Chinese Academy of Medical Sciences, Beijing, China) was cultured at 37°C in RPMI 1640 medium (Gibco, USA) with 10% fetal bovine serum (FBS; Gibco, USA). The oxygen-glucose deprivation and reperfusion (OGD/R) model was established according to previous investigation [Citation18]. Phytic acid (IP6) was pretreated with PC-12 cells 1 h before OGD/R treatment. Cells were seeded in 96-well plates with 1.5 × 104 cells per well. After cells were attached to the bottom, it was treated with various concentration of IP6 (0.2, 0.4, 0.8, 1, 2 mM) for 2 h. Cell-counting kit-8 (CCK-8; Shanghai Yeasen Technology Inc., Shanghai, China) was used to analyze cell viability. Each well added 10 μL of reagent and incubated for 1 h at 37°C before detection. The measured wavelength is 490 nm.
Western blotting
PC-12 cells were harvested using RIPA buffer obtained from Beyotime, China. Protein concentration was detected using NanoDrop™ One/OneC Microvolume UV-Vis Spectrophotometer with Wi-Fi. The equal amount of protein samples was separated using 8% and 10% SDS-PAGE gel for 1 h and subsequently transferred onto polyvinylidene fluoride (PVDF) membrane (Millipore, USA) for 2 h. The PVDF membranes with targeting proteins were blocked with 5% bovine serum albumin (BSA; Roche, Switzerland) at room temperature for 1 h. After being blocked, membranes were incubated with anti-primary antibody, SESN2, Bax, Bcl-2, Cleaved-caspase3, caspase3, cleaved-casepase9, caspase9, phosphorylation (p)-p38 MAPK, p38MAPK, p-JNK, p-ERK, at 4°C overnight. The HRP-conjugated second antibody was used to incubate the membrane at room temperature for 2 h. Enhanced-chemiluminescence (ECL) detection kit for HRP (BD sera technology, Hangzhou, China) was performed to detect the protein band.
OGD/R induced inflammation
Cells (1 × 105 cells/well in 24 well plate) were pretreated with IP6 (0.2, 0.4, 0.6, 1 mM) for 2 h and subsequently established the OGD/R model. Production of IL-10 (ab100765), IL-1β (ab100768), IL-6 (ab100772), TNFα (ab100785), IL-18 (ab213909) in different treated cell supernatants was measured by ELISA from the supplier (Abcam, USA).
Detection of reactive oxygen species (ROS)
Intracellular ROS was evaluated by ROS Assay kit (Shanghai Yeasen Technology Inc., Shanghai, China) using 2ʹ,7ʹ-dichlorofluorescin diacetate (DCFH-DA). 10 μM DCFH-DA proper dye was incubated with cells at 37°C for 30 min. Cells were washed twice with PBS after incubation. 2′,7′-dichlorodihydrofluorescin (DCF) fluorescence was recorded with fluorescence microscope (Olympus, Japan). The fluorescence intensity (relative fluorescence units) was detected in a fluorescence spectrometer (Excitation 485 nm, Emission 530 nm).
Detection of malondialdehyde (MDA) levels and superoxide dismutase (SOD) activities
Following treatment of IP6 and OGD/R, cells were lysed by 0.2% Triton-100 for 25 min to detect the MDA and SOD. The samples were detected using MDA assay kit (Nanjing Jiancheng Bioengineering Institute, Nanjing, China) and SOD assay kit (Nanjing Jiancheng Bioengineering Institute, Nanjing, China) according to manufacturer’s instructions.
Determination of cytotoxicity by lactate dehydrogenase (LDH) leakage assay
Cells (1 × 106 cells/well in 6 well plate) were pretreated with IP6 (0.2, 0.4, 0.6, 1 mM) for 2 h and subsequently established the OGD/R model. The LDH release was determined by a kit (Nanjing Jiancheng Bioengineering Institute, Nanjing, China) according to manuals’ protocol. Cells and culture medium were collected, respectively, after OGD/R injury to assess the LDH levels. LDH leakage rate was performed as a percentage of total LDH activity (LDH in the medium and cells).
TUNEL analysis
PC-12 cells were seeded on 12-well slides (20 mm, Solarbio, Beijing, Nanjing) at a concentration of 1 × 104 cells/slides. After cells treated with IP6 and OGD/R, cells were fixed with 4% paraformaldehyde for 10 min. Subsequently, cells were permeabilized with 0.2% Triton X-100 for 5 min. TUNEL in situ detection kit assay kit (Key gene, Nanjing, China) was performed according to manufacturer’s protocol. Hematoxylin was performed to re-dye the nuclei. Samples were recorded by light microscope (Olympus, Japan).
Statistical analysis
Data were presented by mean value ± standard deviation. Statistical difference between groups was performed by student’s t test and multiple comparations between groups was performed by one-way ANOVA using GraphPad Prism 6.0 statistical software (GraphPad Software, Inc., USA).
Results
Phytic acid suppressed OGD/R-induced inflammation mediated by sestrin2
In the current investigation, we found that the expression of sestrin2 was decreased in PC-12 cells under OGD/R treatment ()), suggesting the potential connection between cerebral ischemia reperfusion injury and sestrin2. As the cytotoxicity of phytic acid was more than 25% in the concentration of 2 mM, we chose 0.2, 0.6, 1 mM to do the following experiments ()). In addition, expression levels of sestrin2 were significantly increased after treating with phytic acid (IP6) depended the concentration ()). To further investigate whether sestrin2 played roles in the effects of phytic acid, sestrin2 was silenced in PC-12 cells ()). We found the anti-proinflammatory mediator, IL-10, was downregulated in OGD/R treatment group, but was enhanced in phytic acid treated groups ((c)). Correspondingly, the inflammatory factors including IL-1β, IL-6, and TNFα were increased in OGD/R group and were reduced in phytic acid-treated groups ((c)). Silencing of sestrin2 hindered the anti-inflammatory effect of phytic acid in PC-12 cells under OGD/R injury.
Figure 1. Expression levels sestrin2 in PC-12 cells treated with OGD/R. (a) The protein levels of sestrin2 in PC-12 cells were determined by western blotting. (b) Cell viability after treated with IP6 was evaluated by CCK-8. IP6, Phytic acid.
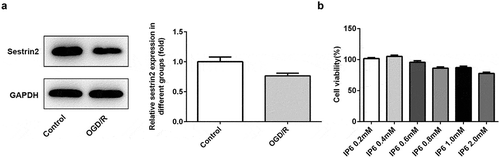
Figure 2. IP6 regulates sestrin2 expression in OGD/R injured PC-12 cells. (a) Expression of sestrin2 after treated with IP6 in different concentrations was estimated by western blot analysis. (b) Transfection efficacy of shRNA-sestrin2 in PC-12 cells. (c) Production of inflammatory factors in PC-12 cells with or without treatment. IP6, Phytic acid.
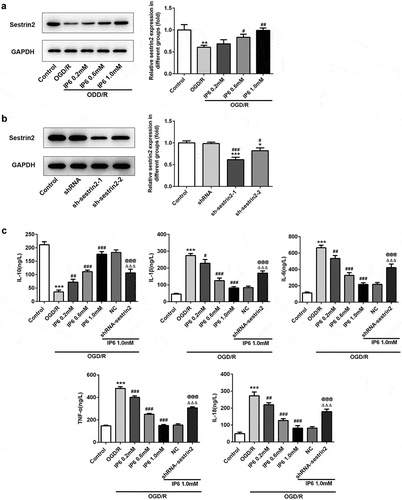
Phytic acid inhibited OGD/R induced oxidative stress via activation of sestrin2
Overload of ROS and superoxide in cells damage the cell function and mitochondria. Compared with control group, OGD/R enhanced the generation of ROS and MDA and destroyed the activity of SOD (c)), whereas phytic acid treatment reversed the OGD/R induced effects. Intercellular oxidative stress over enhanced may lead to cell death. The leakage of LDH was dramatically increased in OGD/R group compared with control group and was inhibited by phytic acid ()). The observations in also showed that downregulation of sestrin2 reduced the protective effects of phytic acid.
Figure 3. IP6 regulates oxidative stress in OGD/R injury mediated by sestrin2. (a). A representative confocal image of DCF-derived fluorescence (green) in PC-12 cells (scale bar: 100 μm). (b, c) The relative levels of MDA and activity of SOD in PC-12 cells with or without treatment. (d) Relative leakage of LDH in PC-12 cells with or without treatment. SESN2, sestrin2. IP6, Phytic acid.
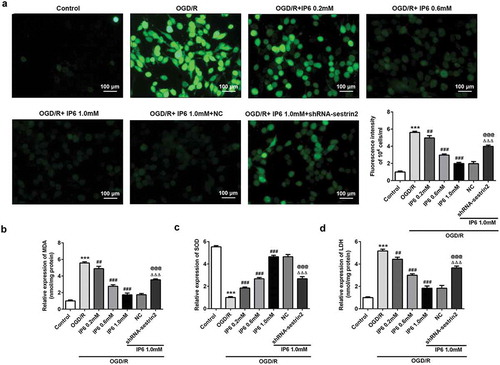
Phytic acid alleviated OGD/R induced apoptosis regulated by sestrin2
Tunel assay showed that phytic acid exerted the protective effects mainly by inhibiting cell apoptosis ()). Protein expression levels of Bcl-2 (anti-apoptotic protein) were promoted and Bax (pro-apoptotic protein) was inhibited by phytic acid in PC-12 cells under OGD/R treatment ()). Additionally, Caspase3 and Caspase9 activation was also found in OGD/R triggered PC-12 cells by the enhancement of cleaved-caspase3 and cleaved-caspase9 ()). Furthermore, we used a CCK-8 assay kit to evaluate the cell viability alteration ()). Data showed that cell viability was dramatically reduced under administration of OGD/R. IP6 with concentration of 0.2, 0.6, 1 mM dramatically enhanced the cell viability in OGD/R conditions. Downregulation of sestrin2 re-enhanced the apoptosis and apoptotic protein expressions in phytic acid-treated OGD/R injured PC12 cells (). These data indicating that phytic acid activates sestrin2 to inhibit cell apoptosis in OGD/R injured PC-12 cells.
Figure 4. Effects of IP6 in OGD/R induced apoptosis. (a) Apoptotic nuclei measurement in PC-12 cells with or without treatment (Scale bar: 50 μm). (b) Apoptotic protein levels of Bax, Bcl-2, Cleaved-caspse3, cleaved-caspase3, cleaved-caspase9, and caspase9 were detected by western blot analysis. SESN2, sestrin2. (c) Cell viability under OGD/R conditions with or without IP6 treatment was analyzed by CCK-8. IP6, Phytic acid.
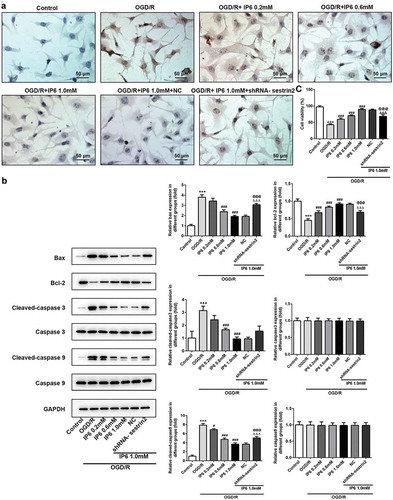
Phytic acid enhanced sestrin2 to exert the protective effects by inhibiting p-38 MAPK signaling
Based on the effects of phytic acid on inflammation, oxidative stress, and apoptosis in OGD/R models, the connections between the damage and MAPK were evaluated. As shown in , the levels of phosphorylated p38 (p-p38) were prominently increased in OGD/R group. Compared with that, pretreatment of phytic acid decreased the levels of p-p38 but did not affect the p38 expression. Also, sestrin2 inhibition reversed the effects of phytic acid. ERK1/2 and JNK1/2 were not dramatically regulated by phytic acid, indicating that phytic acid may regulate the p38 MAPK signaling to exert protective effects in cerebral ischemia-reperfusion injury.
Discussion
Cerebral ischemia induces severely mortality and morbidity. To discover novel therapy strategies for stroke is deadly needed. Previous investigation found that sestrin2 may regulate oxidative stress in neural cell to protect against the ischemic injury [Citation19]. This finding provided us a new target with neuroprotective effect. Phytic acid (IP6) has been proved to prevent inflammation and apoptosis in Parkinson’s disease models, which is a neuron disfunction disease [Citation20]. In the current study, we found that IP6 enhanced sestrin2 expression in PC-12 cells under OGD/R treatment, indicating the potential effects of phytic acid in cerebral ischemia reperfusion induced neural injury. A research shows that the concentration of phytic acid in rat organs ranged from 1.5 mmol/kg for bone to 48.4 mmol/kg for brain, and in human plasma is 0.0002 mmol/L [Citation21]. Given the exogenous IP6-sufficient diet increased levels of IP6 100-fold higher in brain tissues than in plasma [Citation22]. Due to IP6 is pre-treated before OGD/R injury in PC-12 cells and it showed beneficial results, the prophylactic treatment for cerebral ischemia/reperfusion is promised to further investigation.
Cerebral ischemia reperfusion significantly exacerbates pro-inflammatory (TNFα, IL-1β, and IL-18) production and alleviates anti-inflammatory cytokine (IL-10) expression [Citation23,Citation24]. OGD/R treated PC-12 cells expressed lower TNFα, IL-1β, IL-6, and IL-18 and higher IL-10 cytokine under treatment with IP6. Besides, inhibiting of sestrin2 reversed the anti-inflammatory effects of IP6. Severely investigation shows that silencing sestrin2 accelerate cerebral ischemia-reperfusion injury by regulating oxidative stress and apoptosis [Citation10]. In the present study, IP6 activated SOD to remove ROS in injured cells. Besides, low MDA production showed low lipid oxidation. IP6 prominently inhibited the MDA generation indicating the suppression of lipid oxidation of IP6. Excess oxidative stress usually leads to cell death, which is pervasively in reperfusion injury [Citation25]. Our data showed that IP6 reduced cell apoptosis dramatically. Cyt-c in cytoplasm binds to Apaf-1 (apoptotic protease activating factor-1), resulting in the recruitment and activation of caspase-9. Subsequently, the active caspase-9 cleaves procaspase-3/7, which supplies cell death by lysing hundreds of cell substrates [Citation26]. Caspase3 and Cyt-c are abundantly expressed in mitochondria [Citation27]. IP6 dramatically reduced the activation of caspase3, indicating that IP6 might inhibit the mitochondrial apoptosis in OGD/R injured PC-12 cells.
It has come to light that activation of MAPKs signaling shows the protective effects to cerebral ischemia reperfusion [Citation28,Citation29]. We measured p38 MAPK, ERK1/2, and JNK1/2 in cerebral ischemia-reperfusion injury. Data showed that OGD/R induced activation of p38, which can be activated by cellular stress including shock, inflammatory cytokines and lipopolysaccharide, was inhibited by IP6. p38 is phosphorylated under stimulation of extracellular stress and p-p38 turns into nucleus to activate apoptosis [Citation30]. Nevertheless, silencing of sestrin2 re-enhanced the expression of p-p38 which might mediate the effects of IP6 on cell inflammation, oxidative stress, and apoptosis.
According to current data, IP6 combined with the metal ions is not able to go into cells. However, the concentration of IP6 used in this study is much higher than the concentration of it required to chelate metal ions in medium. There must be the free IP6 that is possible to go into cells. Moreover, exogenous IP6 can be accumulated in rat brains [Citation22]. A research shows that IP6 can be taken into cells [Citation31]. Furthermore, it exerts anti-tumor effects or regulating neurological disorders by activating some molecular mechanisms like PI3 K/AKT signaling, and GSK-3β [Citation20,Citation31,Citation32]. Therefore, we believed that it enters cells for neuroprotective effects, not just as an anti-oxidant in cell culture medium. Our data only showed the protective effects of IP6 on cell apoptosis and oxidative stress. Whether exogenous IP6 can go into this PC-12 cells to exert biological effects is not confirmed directly. An HPLC analysis can be used to consider whether it goes into neuron cells in the further investigation.
This study mainly reveals the effects of phytic acid in an in vitro cerebral ischemia-reperfusion model. It showed the neuroprotective effects during the OGD/R injury by regulating inflammation, oxidative stress, and apoptosis. This might be a promising therapeutic strategy for the prevention of cerebral ischemia-reperfusion injury and in vivo investigation still requires to exploring.
Author contribution
Jing Liu and Ying Li made the plan for the project and designed the experiments. Chunli Mei, Xianbin Ning, and Jinfeng Pang carried out the experiments. Jing Liu also drafted the manuscript. Lei Gu, Liang Wu, and Jing Liu contributed to discussions and re-edited the manuscript.
Disclosure statement
No potential conflict of interest was reported by the authors.
Additional information
Funding
References
- Ovbiagele B, Nguyen-Huynh MN. Stroke epidemiology: advancing our understanding of disease mechanism and therapy. Neurotherapeutics. 2011 Jul;8(3):319–329.
- Schaller B, Graf R. Cerebral ischemia and reperfusion: the pathophysiologic concept as a basis for clinical therapy. J Cereb Blood Flow Metab. 2004 Apr;24(4):351–371.
- Yang Z, Weian C, Susu H, et al. Protective effects of mangiferin on cerebral ischemia-reperfusion injury and its mechanisms. Eur J Pharmacol. 2016 Jan;15(771):145–151.
- Brainin M, Zorowitz RD. Advances in stroke: recovery and rehabilitation. Stroke. 2013 Feb;44(2):311–313.
- Li C, Zhang B, Zhu Y, et al. Post-stroke constraint-induced movement therapy increases functional recovery, angiogenesis, and neurogenesis with enhanced expression of HIF-1alpha and VEGF. Curr Neurovasc Res. 2017;14(4):368–377.
- Szelenberger R, Kostka J, Saluk-Bijak J, et al. Pharmacological interventions and rehabilitation approach for enhancing brain self-repair and stroke Recovery. Curr Neuropharmacol. 2020;18(1):51–64.
- Cordani M, Sanchez-Alvarez M, Strippoli R, et al. Sestrins at the Interface of ROS control and autophagy regulation in health and disease. Oxid Med Cell Longev. 2019;2019:1283075.
- Pasha M, Eid AH, Eid AA, et al. Sestrin2 as a novel biomarker and therapeutic target for various diseases. Oxid Med Cell Longev. 2017;2017:3296294.
- Sun MS, Jin H, Sun X, et al. Free radical damage in ischemia-reperfusion injury: an obstacle in acute ischemic stroke after revascularization therapy. Oxid Med Cell Longev. 2018;2018:3804979.
- Li L, Xiao L, Hou Y, et al. Sestrin2 silencing exacerbates cerebral ischemia/reperfusion injury by decreasing mitochondrial biogenesis through the AMPK/PGC-1alpha pathway in rats. Sci Rep. 2016 Jul 25;6:30272.
- Hadi Alkarawi H, Zotz G. Phytic acid in green leaves. Plant Biol (Stuttg). 2014 Jul;16(4):697–701.
- Foster SR, Dilworth LL, Thompson RK, et al. Effects of combined inositol hexakisphosphate and inositol supplement on antioxidant activity and metabolic enzymes in the liver of streptozotocin-induced type 2 diabetic rats. Chem Biol Interact. 2017 Sep 25;275:108–115.
- Grases F, Garcia-Gonzalez R, Torres JJ, et al. Effects of phytic acid on renal stone formation in rats. Scand J Urol Nephrol. 1998 Jul;32(4):261–265.
- Liu C, Chen C, Yang F, et al. Phytic acid improves intestinal mucosal barrier damage and reduces serum levels of proinflammatory cytokines in a 1,2-dimethylhydrazine-induced rat colorectal cancer model. Br J Nutr. 2018 Jul;120(2):121–130.
- Bhowmik A, Ojha D, Goswami D, et al. Inositol hexa phosphoric acid (phytic acid), a nutraceuticals, attenuates iron-induced oxidative stress and alleviates liver injury in iron overloaded mice. Biomed Pharmacother. 2017 Mar;87:443–450.
- da Silva EO, Gerez JR, Hohmann MSN, et al. Phytic acid decreases oxidative stress and intestinal lesions induced by fumonisin B(1) and deoxynivalenol in intestinal explants of pigs. Toxins (Basel). 2019 Jan 4;11(1):18.
- Xu Q, Kanthasamy AG, Reddy MB. Neuroprotective effect of the natural iron chelator, phytic acid in a cell culture model of Parkinson’s disease. Toxicology. 2008 Mar 12;245(12):101–108.
- Wang G, Wang T, Zhang Y, et al. Schizandrin protects against OGD/R-induced neuronal injury by suppressing autophagy: involvement of the AMPK/mTOR pathway. Molecules. 2019 Oct 8;24:19.
- Chuang YC, Yang JL, Yang DI, et al. Roles of Sestrin2 and ribosomal protein S6 in transient global ischemia-induced hippocampal neuronal injury. Int J Mol Sci. 2015 Nov 4;16(11):26406–26416.
- Wang L, Zhang Z, Hou L, et al. Phytic acid attenuates upregulation of GSK-3beta and disturbance of synaptic vesicle recycling in MPTP-induced Parkinson’s disease models. Neurochem Int. 2019 Oct;129:104507.
- March JG, Simonet BM, Grases F. Determination of phytic acid by gas chromatography-mass spectroscopy: application to biological samples. J Chromatogr B Biomed Sci Appl. 2001 Jun 15;757(2):247–255.
- Grases F, Simonet BM, Vucenik I, et al. Effects of exogenous inositol hexakisphosphate (InsP(6)) on the levels of InsP(6) and of inositol trisphosphate (InsP(3)) in malignant cells, tissues and biological fluids. Life Sci. 2002 Aug 16;71(13):1535–1546.
- Storini C, Rossi E, Marrella V, et al. C1-inhibitor protects against brain ischemia-reperfusion injury via inhibition of cell recruitment and inflammation. Neurobiol Dis. 2005 Jun-Jul;19(12):10–17.
- Yang Y, Liu P, Chen L, et al. Therapeutic effect of Ginkgo biloba polysaccharide in rats with focal cerebral ischemia/reperfusion (I/R) injury. Carbohydr Polym. 2013 Nov 6;98(2):1383–1388.
- Granger DN, Kvietys PR. Reperfusion injury and reactive oxygen species: the evolution of a concept. Redox Biol. 2015 Dec;6:524–551.
- Taylor RC, Cullen SP, Martin SJ. Apoptosis: controlled demolition at the cellular level. Nat Rev Mol Cell Biol. 2008 Mar;9(3):231–241.
- Cai J, Yang J, Jones DP. Mitochondrial control of apoptosis: the role of cytochrome c. Biochim Biophys Acta. 1998 Aug 10;1366(12):139–149.
- Armstead WM, Ganguly K, Riley J, et al. RBC-coupled tPA prevents whereas tPA aggravates JNK MAPK-mediated impairment of ATP- and Ca-sensitive K channel-mediated cerebrovasodilation after cerebral photothrombosis. Transl Stroke Res. 2012 3; Mar(1): 114–121.
- Maddahi A, Edvinsson L. Cerebral ischemia induces microvascular pro-inflammatory cytokine expression via the MEK/ERK pathway. J Neuroinflammation. 2010 Feb;26(7):14.
- Wang T, Wang F, Yu L, et al. Nobiletin alleviates cerebral ischemic-reperfusion injury via MAPK signaling pathway. Am J Transl Res. 2019;11(9):5967–5977.
- Cui F, Sun J, Yang X, et al. Ultrasensitive fluorometric determination of iron(iii) and inositol hexaphosphate in cancerous and bacterial cells by using carbon dots with bright yellow fluorescence. Analyst. 2019 Aug 5;144(16):5010–5021.
- Masunaga T, Murao N, Tateishi H, et al. Anti-cancer activity of the cell membrane-permeable phytic acid prodrug. Bioorg Chem. 2019 Nov;92:103240.