ABSTRACT
Atopic dermatitis (AD) is a chronic inflammatory skin disease characterized by pruritic and eczematous skin lesions. The skin of AD patients is generally in a dried condition. Therefore, it is important for AD patients to manage skin moisturization. In this study, we examined the effects of orally administered fermented barley extract P (FBEP), which is prepared from a supernatant of barley shochu distillery by-product, on stratum corneum (SC) hydration and transepidermal water loss (TEWL) in AD-like lesions induced in hairless mice using 2,4,6-trinitrochlorobenzene. Oral administration of FBEP increased SC hydration and decreased TEWL in the dorsal skin of this mouse model. Further fractionation of FBEP showed that a pyroglutamyl pentapeptide, pEQPFP comprising all -L-form amino acids, is responsible for these activities. These results suggested that this pyroglutamyl pentapeptide may serve as a modality for the treatment of AD.
GRAPHICAL ABSTRACT
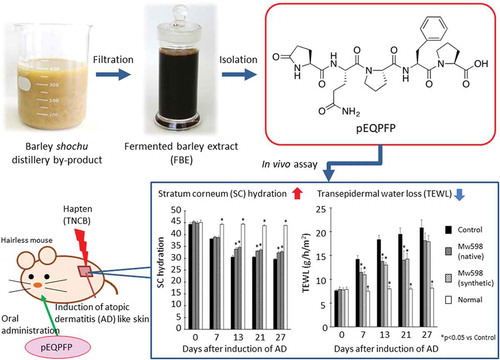
A novel pyroglutamyl pentapeptide was isolated from FBE. Oral administration of the peptide improved epidermal barrier of AD-like hairless mice.
Atopic dermatitis (AD) is a chronic, pruritic, relapsing inflammatory skin disease that affects 10–20% of children and 1–3% of adults worldwide [Citation1,Citation2]. Pruritus results in a vicious cycle of itching and scratching, further compromising the already-damaged skin barrier. Barrier disruption and the continuous exposure to allergens are responsible for the development of AD. The cutaneous barrier dysfunction of AD includes a decrease in the water-retention capability of the stratum corneum (SC). Therefore, the skin of AD patients is generally in a dried condition. Several authors have reported significantly decreased skin hydration in the lesional skin of AD patients compared to healthy skin [Citation3–Citation7]. Darlenski et al. reported that an increase in skin hydration improves AD, whereas a disruption of dermal hydration balance and increased dryness of skin aggravates AD [Citation8]. The treatment strategy usually involves the curing of exanthemas followed by long-term management using exanthema-prevention strategies. While topical steroids remain the mainstay of treatment of exanthemas, it is important for AD patients to manage daily skin moisturization in the long term.
In recent years, considerable attention has been focused on diets that improve AD. Foods fermented with koji–mold or yeast have been reported to have effects on skin. Kim et al. reported that oral administration of an ethanol extract of Canavalia gladiata (sword beans) fermented with yellow koji–mold (Aspergillus oryzae) increased epidermal hydration and improved AD [Citation9]. Yeh et al. found that a legume product fermented by Saccharomyces cerevisiae attenuated cutaneous AD-like inflammation [Citation10]. These results suggest that products fermented with koji-mold and yeast represent potential candidates for the treatment of AD.
Fermented barley extract (FBE) is a supernatant of barley shochu distillery by-products and contains high levels of amino acids, peptides, organic acids, inorganic salts, and vitamins. Barley shochu is made from barley and brewed with yeast (S. cerevisiae) and white koji-mold (Aspergillus kawachii). Therefore, various components in FBE are derived from decomposition products of barley and metabolites of these two microorganisms. We have vigorously investigated various physiological effects of FBE, including activities that counter hepatic disorders [Citation11,Citation12] and inflammation [Citation13] and that accelerate urinary excretion of uric acid, in both animal and human studies [Citation14,Citation15]. Furthermore, Hokazono et al. reported that oral administration of fermented barley extract P (FBEP), a fraction of FBE that adsorbs to a hydrophobic resin, to normal mice significantly enhanced erythrocyte glutathione peroxidase (GPx) activity and increased hepatic glutathione levels compared to those of a control group [Citation16]. Given a report that increasing antioxidant activity such as GPx in NC/Nga mice suppresses Th2 cytokine and IgE levels [Citation17], we inferred that oral administration of FBEP may be useful for treatment of AD. However, FBEP’s effect on AD-model mice remains unknown.
In the present study, we first examined the effect of FBEP on the epidermal barrier by evaluating SC hydration and transepidermal water loss (TEWL) of AD-like skin lesions generated in hairless mice by continuous topical application of the hapten 2,4,6-trinitrochlorobenzene (TNCB). The TNCB-treated mice overproduce IgE and are suitable for studying the pathology of AD skin lesions and the immunological characteristics of these lesions [Citation18]. Given that oral administration of FBEP showed beneficial effects on this animal model of AD, we further fractionated FBEP to identify the compound(s) responsible for this activity.
Materials and methods
Materials
Fresh barley shochu distillery by-product was provided by Sanwa Shurui Co., Ltd. (Oita, Japan). FBE was produced by filtering this by-product as described previously [Citation15]. FBEP was prepared from the FBE using a synthetic adsorbent and ion-exchange resin as reported in the same work [Citation15]. The synthetic all-L-form pyroglutamyl peptides were custom synthesized by Genscript (Piscataway, NJ, USA). All other chemicals were commercially available and obtained as analytical-grade reagents.
Purification of Mw486 and Mw598 by preparative HPLC
A 600-L volume of FBE was passed over a 60-L Diaion® HP-20 column pre-equilibrated with tap water. After washing with 300 L of tap water, the adsorbed material (FBEP) was eluted by sequential addition of 120 L of 20% (fractions P0–20), 120 L of 40% (P20–40), and 120 L of 60% (P40–60) ethanol:water (v/v). All eluates were evaporated under reduced pressure to remove the ethanol and then freeze-dried.
The lyophilized material from fractions P20–40 (20 g) was extracted using 100 mL of chloroform:methanol = 80:20 (v/v). After filtering with No. 2 filter paper (Advantec Toyo, Tokyo, Japan), the filtrate was vacuum-dried to remove the solvent. The resulting solids were dissolved in 3.5 mL water to yield a 0.1 g/mL solution, which then was subjected to high-performance liquid chromatography (HPLC) using a Prominence System with an LC-20 pump and a SPD-20A ultraviolet detector (Shimadzu Corporation, Kyoto, Japan) equipped with a Synergi® 4 µm Hydro-RP 80 Å column (250 × 21.2 mm; Phenomenex, Inc., Torrance, CA, USA). The mobile phase consisted of isocratic water:acetonitrile = 85:15 containing 0.05% trifluoroacetic acid (TFA); the flow rate was 10 mL/min. The eluate was monitored at 210 nm.
The fractions containing a compound with a molecular weight of 486 (Mw486) were collected. Following lyophilization, this sample (2.0 g) was washed with 20 mL of water and centrifuged at 1,519 × g for 5 min. The resultant insoluble material contained Mw486 at high purity, as confirmed by liquid chromatography-mass spectrometry (LC-MS) analysis.
Similarly, the fractions containing a compound with a molecular weight of 598 (Mw598) were collected. Following lyophilization, this sample (0.16 g) was dissolved in 1.6 mL of water and subjected to HPLC purification using a Prominence System equipped with a Synergi® 4 µm Hydro-RP 80 Å column (250 × 21.2 mm) and eluted with 0.05% aqueous TFA (A) and acetonitrile containing 0.05% TFA (B) at a flow rate of 10 mL/min. Separation was achieved using the following gradient program: a linear gradient from 20% to 40% solvent B (0–15 min). The eluate was monitored at 210 nm. The HPLC peak containing Mw598 was collected and lyophilized. The purified Mw486 and Mw598 samples were used for animal experiments and structural analyses.
Animals
Male hairless mice (Hos:HR1), 6 weeks old, were purchased from Sankyo Labo Service Corporation (Tokyo, Japan) and maintained under controlled conditions (24 ± 1°C; relative humidity, 55 ± 5%; light conditions, 12-h/12-h light/dark cycle). The mice were provided with ad libitum access to commercial pelleted food (CRF-1, Oriental Yeast Co., Ltd., Tokyo, Japan) and distilled water. Following a one-week period of quarantine and acclimatization, the animals were randomized and assigned to groups designed to have similar mean and standard deviation body weights before the initiation of the in-life experiments. To the CRF-1 diet, 5% (w/w) FBEP was added. The purified and synthetic samples were administered orally using a stomach sonde at a per-day dose of 6 mg for Mw486 and 3 mg for Mw598. The animal experiments were approved by the Animal Research Committee of Material Research Center Co., Ltd. (Approval Numbers 201712-002 and 201904-008). These experiments were performed in accordance with “Standards Relating to the Care and Management of Laboratory Animals and Relief of Pain” (Ministry of the Environment Notification No. 88, April 28 2006; most-recent revision: Ministry of the Environment Notification No. 84, August 30 2013).
Sensitization of mice
On day −7, mice were sensitized by topical application to the dorsal skin of 100 µL of TNCB dissolved to 1% in acetone. On day 0, mice were challenged again, by topical application to the dorsal skin of 100 µL of 1% TNCB solution every second day until day 21 or day 27.
Skin analysis
SC hydration and TEWL of dorsal skin were measured using a Corneometer® CM825MP and a Tewameter® TM300MP (Courage and Khazaka Electronics, Cologne, Germany), respectively, 5 h after that day’s challenge. SC hydration was expressed as relative capacitance.
Mass spectrometric analysis
LC-MS measurements were carried out in a positive ion mode on an LCMS-IT-TOF mass spectrometer (Shimadzu Corporation) equipped with an electrospray ionization source. HPLC separation was performed on a C18 microbore column (1 × 250 mm, Grace Vydac®, Hesperia, CA, USA). The column was eluted with 0.1% formic acid in water (C) and 0.1% formic acid in acetonitrile (D), at a flow rate of 0.05 mL/min, using isocratic elution with 5% D for 5 min followed by a linear gradient of 5 to 50% D over 45 min. For MS/MS analysis, precursor ions were selected automatically, and product ion spectra were obtained using argon as the collision gas. The mass scale was calibrated externally using sodium trifluoroacetate cluster ions. HRMS data were obtained on a Bruker timsTOF® spectrometer equipped with an atmospheric pressure chemical ionization source.
NMR spectroscopy
NMR spectra were recorded on a Bruker AVANCEIII 600 spectrometer (600 MHz for 1H; 151 MHz for 13C) in CD3SOCD3. Chemical shifts are reported in parts per million relative to tetramethylsilane as an internal standard.
Statistical analysis
Where relevant, the data are expressed as mean ± SEM. Comparison of two groups was performed using a two-tailed non-paired Student’s t-test, Welch’s t-test, or Mann-Whitney U-test to detect significant differences. Comparisons of data among more than two groups were assessed using a Dunnett’s test or Steel’s test for comparison with the control group. The software MEPHAS (http://www.gen-info.osaka-u.ac.jp/testdocs/tomocom/) was used for statistical analysis. p values of <0.05 were considered significant.
Results
Improvement of epidermal barrier by FBEP
We examined the influence of FBEP on SC hydration and TEWL. After 21 days of oral administration, there were significant differences in SC hydration and TEWL between the FBEP group and the control group (). The results suggested that compounds responsible for these activities were contained in FBEP. Therefore, in the following experiments, we fractionated FBEP to identify the active compounds.
Figure 1. Effects of FBEP on dorsal skin in mice after 21 days of oral administration. SC hydration (a) and TEWL (b) of dorsal skin in mice were analyzed. The Mann-Whitney U-test was used for statistical analysis. Data are presented as mean ± SEM (n = 7). Significant differences are indicated by asterisks (**p < 0.01).
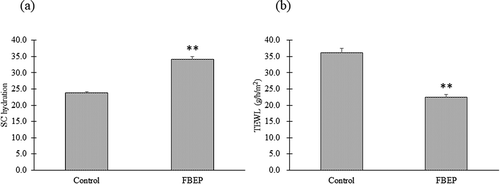
Purification of Mw486 and Mw598
In a preliminary experiment, FBEP was subjected to HP-20 column chromatography (Supplemental material, “Fractionation of FBEP”). The fractions thus obtained were tested for their influence on SC hydration and TEWL of dorsal skin of mice when administered orally (Supplemental material, “Animals”). We found that the active compounds were contained in the fractions eluted with 99% ethanol after elution with 20% ethanol (Supplemental Figure 1, P20–99). Further fractionation of P20–99 by silica-gel column chromatography yielded active fractions (Supplemental Figure 2, Fr. 2 and Fr. 3), and LC/MS analysis (Supplemental material, “LC-MS analysis of Fr. 2 and Fr. 3”) revealed that these fractions contained a compound with a molecular weight of 486 (Mw486) and a compound with a molecular weight of 598 (Mw598) (Supplemental Figures 3–6).
Assuming that one or both of these compounds affect SC hydration and TEWL, we next purified the compounds by large-scale synthetic adsorbent column chromatography and preparative HPLC. In this experiment, we fractionated the adsorbates by more gradual stepwise increase of ethanol concentration than in the preliminary experiment. A total of 1,200 L of FBE was subjected to HP–20 column chromatography. After washing with tap water, the adsorbate (FBEP) was fractionated by stepwise elution with 20%, 40%, and 60% ethanol to obtain fractions P0–20, P20–40, and P40–60, respectively, which after lyophilization yielded 9,651 g, 4,224 g, and 495 g of residues, respectively. Since LC-MS analysis showed that both Mw486 and Mw598 were present in P20–40, we did not collect the fractions eluted with ethanol at a concentration higher than 60%. To isolate these compounds from P20–40, the sample was extracted with chloroform:methanol = 80:20 (v/v). After drying the supernatant, the residue was dissolved in water and subjected to preparative HPLC. Mw486 and Mw598 eluted at retention times of 16.2–18.9 min and 42.1–49.6 min, respectively. Given that Mw486 was insoluble in water, this compound was purified by collecting the insoluble precipitate after suspending the fraction containing Mw486 in water. On the other hand, Mw598 was purified by successive rounds of preparative HPLC. Pure Mw598 was obtained by collecting the peak eluted at 12.1–13.4 min in the second HPLC separation. In the end, more than 1.5 g of each sample were obtained. These purified samples were used for structural analyses and animal experiments.
Structural analysis of Mw486 and Mw598
The molecular formula of Mw486 was determined as C24H30N4O7 on the basis of HRMS analysis (m/z [M+H]+: calculated, 487.2187; found, 487.2188), and the degree of unsaturation was calculated to be 12. Assignment of 1H and 13C NMR signals, summarized in , was based on 1H-1H correlation spectroscopy (COSY), heteronuclear single quantum coherence (HSQC), and heteronuclear multiple bond correlation (HMBC). Brief inspection of 13C and 1H NMR spectra of Mw486 indicated the existence of five carbonyls (169.36, 170.60, 170.87, 173.13, and 177.30 ppm), four methine α-carbons (51.99, 53.62, 58.53, and 59.32 ppm), and four α-protons (4.21, 4.30, 4.34, and 4.56 ppm) of α-amino acid, suggesting that Mw486 is a peptidic compound. Indeed, amino acid analysis of Mw486 revealed the presence of one Glu, one Tyr, and two Pro (Supplemental Figure 7). The configuration of all of these amino acids was determined to be L based on the advanced Marfey’s method [Citation19] (Supplemental Figure 8). The sum of the degree of unsaturation of a peptide consisting of these amino acids (11) is smaller than the above-mentioned value (12). This observation suggested the presence of a cyclic structure in Mw486, which could be attributed to conversion of the N-terminal Glu to pyroglutamate (pGlu). The presence of the pGlu was confirmed by the HMBC correlations between the amide proton (7.74 ppm) and the γ-carbonyl (177.30 ppm) as well as the γ-carbon (28.89 ppm), as shown in ). The pGlu residue is presumed to have been hydrolyzed to Glu under the harsh acidic conditions employed during amino acid analysis. The order of the connection of amino acids of Mw486 was determined by the HMBC correlations between the α-proton of the terminal Pro (4.21 ppm) and the Tyr carbonyl (169.36 ppm), the amide proton of Tyr (7.84 ppm) and the carbonyl of the internal Pro (170.87 ppm), and the α-proton of the internal Pro (4.30 ppm) and the α-carbonyl of pGlu (170.60 ppm), as shown in ). Thus, the structure of Mw486 was determined as L-pGlu-L-Pro-L-Tyr-L-Pro (pEPYP, )). The MS/MS analysis of Mw486 supported the determined structure ()). The predominant fragment ion with m/z 279.1 is inferred to be derived from cleavage of an amide bond on the C-terminal side of the Pro residue. The structure was unambiguously confirmed by comparison of HPLC retention times and MS/MS fragmentation profiles between native and synthetic Mw486 (Supplemental Figures 9–11). The NMR spectra of native Mw486 are included in the Supplemental material (Supplemental Figures 17–21).
Table 1. 1H and 13C NMR of Mw486 and Mw589 in DMSO-d6.a–c.
Figure 2. The 1H-1H COSY and HMBC correlations of Mw486 (a) and Mw598 (b). For clarity, the configurations of the amino acids are omitted.
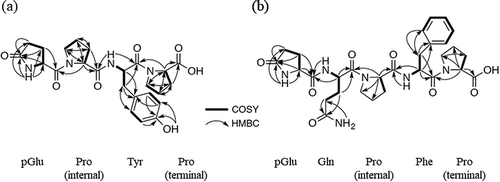
With respect to Mw598, this compound’s molecular formula was determined as C29H38N6O8 based on HRMS analysis (m/z [M+H]+: calculated, 599.2825; found, 599.2824), and the degree of unsaturation was calculated to be 14. As for Mw486, 13C and 1H NMR data of Mw598 () represented seven carbonyls (169.23, 169.76, 171.20, 172.21, 173.11, 173.82, and 177.32 ppm), five methine α-carbons (49.66, 51.80, 55.13, 58.55, and 59.17 ppm), and five α-protons (4.04, 4.23, 4.30, 4.48, and 4.66 ppm) of α-amino acid, suggesting the peptidic nature of Mw598. Amino acid analysis of Mw598 revealed the presence of two Glu, one Phe, and two Pro (Supplemental Figure 12); the advanced Marfey’s method [Citation19] revealed the configuration of all these amino acids to be L (Supplemental Figure 13). As observed in the structure of Mw486, Mw598 also contained an N-terminal pGlu residue, an observation that was confirmed by the HMBC correlations between the amide proton (7.78 ppm) and the γ-carbonyl (177.32 ppm) as well as the γ-carbon (29.07 ppm) of the pGlu residue ()). One of two Glu detected by amino acid analysis was inferred to be derived from this pGlu residue. Additionally, the 1H-1H COSY correlations between the two amide protons (6.85 and 7.27 ppm) at side chains, and the HMBC correlations between these protons and the γ-carbon (30.84 ppm) in the same residue ()) indicated the existence of a Gln residue, which corresponds to the other Glu detected by amino acid analysis. Finally, the amino acid sequence of Mw598 was determined as L-pGlu-L-Gln-L-Pro-L-Phe-L-Pro (pEQPFP, )), based on the HMBC correlations between the α-proton of the terminal Pro (4.23 ppm) and the Phe carbonyl (169.23 ppm), the α-proton of Phe (4.66 ppm) and the carbonyl of the internal Pro (171.20 ppm), the α-proton of the internal Pro (4.30 ppm) and the α-carbonyl of Gln (169.76 ppm), and the Gln α-proton (4.48 ppm) and the α-carbonyl of pGlu (172.21 pm) ()). The MS/MS analysis of Mw598 supported the determined structure ()). The structure was unambiguously confirmed by comparison of HPLC retention times, MS/MS fragmentation profiles, and 1H and 13C NMR data between native and synthetic Mw598. (Supplemental Figures 14–16, Supplemental Tables 1 and 2). The NMR spectra of native Mw598 (Supplemental Figures 22–26) and synthetic Mw598 (Supplemental Figures 27–31) are included in the Supplemental material.
Effect of oral administration of Mw486 and Mw598 on epidermal barrier function
We examined the effects of the isolated Mw486 and Mw598 on hairless mice treated with TNCB after 21 days of oral administration of the peptides. There were no significant differences between the control group and the Mw486-treated group in terms of SC hydration and TEWL ()), whereas the Mw598-treated group showed a significant increase in SC hydration and decrease in TEWL compared with the control group on day 21 ()). Therefore, Mw598 was found to be the active compound in FBE.
Figure 5. Effects of native Mw486 and Mw598 on dorsal skin in mice after 21 days of oral administration. SC hydration (a) and TEWL (b) of dorsal skin in the Mw486 group, and SC hydration (c) and TEWL (d) of dorsal skin in the Mw598 group were analyzed. Differences between the control group and the test group were analyzed using Welch’s t-test for (a), and using a two-tailed non-paired Student’s t-test for (b), (c), and (d). Data are presented as mean ± SEM (n = 6). Significant differences are indicated by asterisks (*p < 0.05, **p < 0.01).
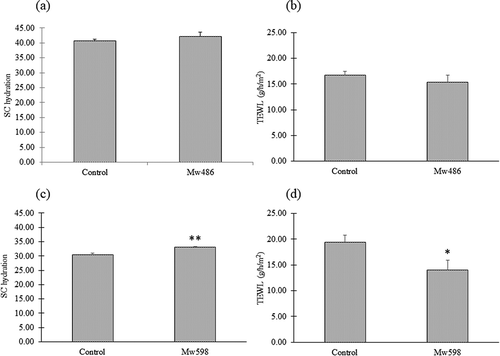
Since Mw598 was identified as the pyroglutamyl pentapeptide indicated in ), we next evaluated the effects of the pyroglutamyl pentapeptide purified from FBE as well as a synthetic version on SC hydration and TEWL of dorsal skin of mice when orally administered. As shown in ), SC hydration was significantly increased in Mw598-administered mice compared with the control group from day 13 to day 27. As shown in ), TEWL was significantly decreased in Mw598-administered mice compared with the control group from day 7 to day 21. These results confirmed that oral administration of the pyroglutamyl pentapeptide affected SC hydration and TEWL.
Figure 6. Effects of oral administration of native or synthetic Mw598 on SC hydration (a) and TEWL (b) of dorsal skin. Data are presented as mean ± SEM (n = 6). Differences between the control group and the test group were analyzed using Steel’s test for (a) and Dunnett’s test for (b). Significant differences are indicated by asterisks (*p < 0.05).
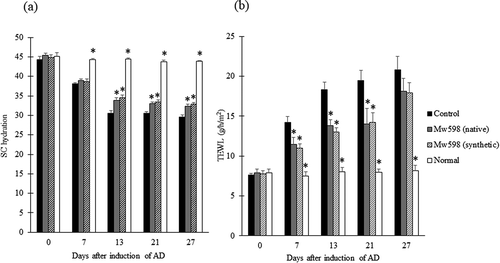
Discussion
In this study, we found that oral administration of FBEP affected SC hydration and TEWL of dorsal skin in AD-like mice (). In order to identify the active compounds, we performed HP-20 and silica-gel column chromatography and preparative HPLC. As a result, Mw486 and Mw598 were found in active fractions (Supplemental Figure 1–6) and identified as pEPYP and pEQPFP, respectively (–). Oral administration of pEQPFP yielded increased SC hydration and decreased TEWL compared to control, whereas pEPYP showed no effect on dorsal skin (). As shown in , there were significant differences in the effect on both SC hydration and TEWL between the control mice and the pEQPFP-administered mice from day 7 to day 21, whereas no significant differences were found on day 27 in the case of TEWL. This result suggests that oral administration of pEQPFP may be more effective on SC hydration than on TEWL in countering long-term barrier disruption. However, it is possible that the relatively low effect of pEQPFP on TEWL may be caused by decreased intercellular lipids due to continuous application of the organic solvent [Citation20].
The present results indicated that pEQPFP is the compound in FBEP responsible for the effect on SC hydration and TEWL. Other pyroglutamyl peptides also have been shown to exert various biological activities. For example, pEHP-amide is known as thyrotropin-releasing hormone [Citation21], and pEL attenuates D-galactosamine-induced acute hepatitis [Citation22] and dextran sulfate sodium (DSS)-induced colitis in mice [Citation23]. However, there has been, to our knowledge, no report that oral administration of pyroglutamyl peptide influences skin hydration and barrier function in AD-like mice. Thus, this work is the first report of an orally administered pyroglutamyl peptide that increases SC hydration and decreases TEWL.
Since FBE is the supernatant of a barley shochu distillery by-product, this extract contains various metabolites and degradation products derived from barley, white koji-mold, and yeast. pEQPFP appears to be derived from hordein, which is decomposed by white koji-mold during the brewing of shochu. Hordein is the major endosperm storage protein of barley, comprising 30–50% of the total grain protein in barley [Citation24–Citation26] and is composed of four sub-fractions (B hordein, C hordein, γ-hordein, and D hordein) that are distinguished by their electrophoretic mobility and amino acid composition [Citation26]. It has been reported that pyroglutamyl peptides are produced from peptides with a glutaminyl residue at their amino termini [Citation27]. Thus, we infer that pEQPFP is derived from QQPFP. We found that B hordein (Accession Number ABB82614), C hordein (Accession Number AAA92333), and γ-hordein (Accession Number AFM77738) are rich in the QQPFP sequence. Therefore, the pyroglutamyl pentapeptide presumably is produced from these proteins by the action of a protease secreted by white koji-mold. Further studies are underway to elucidate the detailed mechanism of this process.
Although the mechanism by which the oral administration of pEQPFP affects skin function is unknown, multiple studies have suggested an intimate connection between the gut and skin conditions. Gut microbiota have been shown to control psoriasis-like skin inflammation by altering the T cell response [Citation28]. Kaikiri et al. also reported that oral administration of 10-hydroxy-cis-12-octadecenoic acid alleviates AD through alteration of the intestinal microbiota [Citation29]. On the other hand, oral administration of pyroglutamyl peptides reportedly affects gut microbiota composition. Wada et al. showed that oral administration of a pyroglutamyl dipeptide (pEL) attenuates DSS-induced colitis through normalizing the population of Bacteroides and Firmicutes in the colon [Citation23]. Therefore, oral administration of pEQPFP may affect the epidermal barrier by altering the gut microbiota, although further studies are needed to elucidate the detailed mechanism underlying the AD-improving effect of pEQPFP.
In conclusion, the present study revealed that a pyroglutamyl pentapeptide, pEQPFP, is present in FBE, and that oral administration of this pentapeptide increases SC hydration and decreases TEWL in a mouse model of AD. This pentapeptide is expected to be useful as a skin-moisturizing food stuff for the improvement and prevention of AD. Future studies should focus on the mechanism by which oral administration of pEQPFP improves AD.
Author contribution
Naruyuki Maruoka and Hideki Hokazono designed the research; Naruyuki Maruoka, Daigo Ando, and Bunta Watanabe performed the experiments; Naruyuki Maruoka, Bunta Watanabe and Masahiro Miyashita analyzed the data; and Naruyuki Maruoka, Bunta Watanabe, Masahiro Miyashita, and Tatsuo Kurihara wrote the manuscript. All authors read and approved the final manuscript.
Supplemental_Material-Revision2_Maruoka_.pdf
Download PDF (2.5 MB)Acknowledgments
We are grateful to Dr. Hitoshi Ishida for his technical assistance. Parts of the experimental measurements were carried out using the Bruker NMR and MS spectrometers in the Joint Usage/Research Center (JURC) at the Institute for Chemical Research, Kyoto University. The authors are greatly indebted to Ms. Kyoko Ohmine and Ms. Akiko Fujihashi for performing instrumental analyses.
Disclosure statement
No potential conflict of interest was reported by the authors.
Supplementary material
Supplemental data for this article can be accessed here.
Additional information
Funding
References
- Leung DY, Boguniewicz M, Howell MD, et al. New insights into atopic dermatitis. J Clin Investig. 2004;113(5):651–657.
- Asher MI, Montefort S, Björkstén B, et al. Worldwide time trends in the prevalence of symptoms of asthma, allergic rhinoconjunctivitis, and eczema in childhood: ISAAC phases one and three repeat multicountry cross-sectional surveys. Lancet. 2006;368(9537):733–743.
- Kim DW, Park JY, Na GY, et al. Correlation of clinical features and skin barrier function in adolescent and adult patients with atopic dermatitis. Int J Dermatol. 2006;45(6):698–701.
- Seidenari ST, Giusti G. Objective assessment of the skin of children affected by atopic dermatitis: a study of pH, capacitance and TEWL in eczematous and clinically uninvolved skin. Acta Derm Venereol. 1995;75(6):429–433.
- Hon KL, Wong KY, Leung TF, et al. Comparison of skin hydration evaluation sites and correlations among skin hydration, transepidermal water loss, SCORAD index, Nottingham Eczema Severity Score, and quality of life in patients with atopic dermatitis. Am J Clin Dermatol. 2008;9(1):45–50.
- Choi SJ, Song MG, Sung WT, et al. Comparison of transepidermal water loss, capacitance and pH values in the skin between intrinsic and extrinsic atopic dermatitis patients. J Korean Med Sci. 2003;18(1):93–96.
- Pellacani G, Seidenari S. Water sorption-desorption test and moisture accumulation test for functional assessment of atopic skin in children. Acta Derm Venereol. 2001;81(2):100–103.
- Darlenski R, Kazandjieva J, Tsankov N, et al. Acute irritant threshold correlates with barrier function, skin hydration and contact hypersensitivity in atopic dermatitis and rosacea. Exp Dermatol. 2013;22(11):752–753.
- Kim OK, Chang JY, Nam DE, et al. Effect of Canavalia gladiata extract fermented with Aspergillus oryzae on the development of atopic dermatitis in NC/Nga mice. Int Arch Allergy Immunol. 2015;168(2):79–89.
- Yeh CY, Jung CJ, Huang CN, et al. A legume product fermented by Saccharomyces cerevisiae modulates cutaneous atopic dermatitis-like inflammation in mice. BMC Complement Altern Med. 2014;14(1):194.
- Mochizuki S, Miyamoto A, Hagiwara M, et al. Effects of barley shochu lees on the prevention of fatty livers in rats fed an orotic acid-containing diet. J Brew Soc Jpn. 2001;96(8):559–563. Japanese.
- Mochizuki S, Miyamoto A, Oga S, et al. Effects of barley shochu lees on D-galactosamine-induced hepatic injury in rats. J Brew Soc Jpn. 2005;100(2):135–140. Japanese.
- Giriwono PE, Hashimoto T, Ohsaki Y, et al. Extract of fermented barley attenuates chronic alcohol induced liver damage by increasing antioxidative activities. Food Res Intl. 2010;43(1):118–124.
- Hokazono H, Omori T, Ono K. Anti-hyperuricemic effect of fermented barley extract is associated with increased urinary uric acid excretion. Food Sci Technol Res. 2010;16(4):295–304.
- Hokazono H, Omori T, Yamamoto T, et al. Effects of a fermented barley extract on subjects with slightly high serum uric acid or mild hyperuricemia. Biosci Biotechnol Biochem. 2010;74(4):828–834.
- Hokazono H, Omori T, Suzuki H, et al. Effects of fermented barley extract on antioxidant status in mice. Food Sci Technol Res. 2009;15(6):599–604.
- Yoon YS, Sajo ME, Ignacio RM, et al. Positive effects of hydrogen water on 2, 4-dinitrochlorobenzene-induced atopic dermatitis in NC/Nga mice. Biol Pharm Bull. 2014;37(9):1480–1485.
- Matsumoto K, Mizukoshi K, Oyobikawa M, et al. Establishment of an atopic dermatitis-like skin model in a hairless mouse by repeated elicitation of contact hypersensitivity that enables to conduct functional analyses of the stratum corneum with various non-invasive biophysical instruments. Skin Res Technol. 2004;10(2):122–129.
- Fujii K, Ikai Y, Oka H, et al. A nonempirical method using LC/MS for determination of the absolute configuration of constituent amino acids in a peptide: combination of Marfey’s method with mass spectrometry and its practical application. Anal Chem. 1997;69(24):5146–5151.
- Rissmann R, Oudshoorn MH, Hennink WE, et al. Skin barrier disruption by acetone: observations in a hairless mouse skin model. Arch Dermatol Res. 2009;301(8):609–613.
- Bøler J, Enzmann F, Folkers K, et al. The identity of chemical and hormonal properties of the thyrotropin releasing hormone and pyroglutamyl-histidyl-proline amide. Biochem Biophys Res Commun. 1969;37(4):705–710.
- Sato K, Egashira Y, Ono S, et al. Identification of a hepatoprotective peptide in wheat gluten hydrolysate against D-galactosamine-induced acute hepatitis in rats. J Agric Food Chem. 2013;61(26):6304–6310.
- Wada S, Sato K, Ohta R, et al. Ingestion of low dose pyroglutamyl leucine improves dextran sulfate sodium-induced colitis and intestinal microbiota in mice. J Agric Food Chem. 2013;61(37):8807–8813.
- Jonnasen IB, Ingversen J, Brandt A. Synthesis of SPII albumin, β-amylase and chymotrypsin inhibitor CI-1 on polysomes from the endoplasmic reticulum of barley endosperm. Carlsberg Res Commun. 1981;46(3):175–181.
- Kirkman MA, Shewry PR, Miflin BJ. The effect of nitrogen nutrition on the lysine content and protein composition of barley seeds. J Sci Food Agric. 1982;33(2):115–127.
- Shewry PR, Franklin J, Parmar S, et al. The effects of sulphur starvation on the amino acid and protein compositions of barley grain. J Cereal Sci. 1983;1(1):21–31.
- Sato K, Nisimura R, Suzuki Y, et al. Occurrence of indigestible pyroglutamyl peptides in an enzymatic hydrolysate of wheat gluten prepared on an industrial scale. J Agric Food Chem. 1998;46(9):3403–3405.
- Zakostelska Z, Malkova J, Klimešová K, et al. Intestinal microbiota promotes psoriasis-like skin inflammation by enhancing Th17 response. PLoS One. 2016;11(7):e0159539.
- Kaikiri H, Miyamoto J, Kawakami T, et al. Supplemental feeding of a gut microbial metabolite of linoleic acid, 10-hydroxy-cis-12-octadecenoic acid, alleviates spontaneous atopic dermatitis and modulates intestinal microbiota in NC/nga mice. Int J Food Sci Nutr. 2017;68(8):941–951.