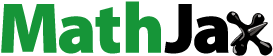
ABSTRACT
The aims of this study were to compare the effectiveness of different drying methods and to investigate the effects of adding a series of individual protectant such as skim milk, sucrose, maltodextrin, and corn starch for preserving Lactobacillus acidophilus FTDC 3081 cells during spray and freeze-drying and storage at different temperatures. Results showed a remarkable high survival rate of 70–80% immediately after spray- and freeze-drying in which the cell viability retained at the range of 109 to 1010 CFU/mL. After a month of storage, maltodextrin showed higher protective ability on both spray- and freeze-dried cells as compared to other protective agents at 4°C, 25°C, and 40°C. A complete loss in viability of spray-dried L. acidophilus FTDC 3081 was observed after a month at 40°C in the absence of protective agent.
GRAPHICAL ABSTRACT
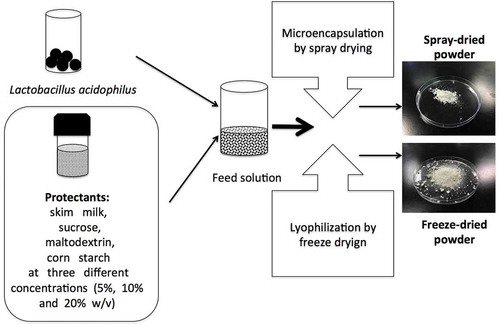
A schematic overview of freeze-drying and spray-drying of L. acidophilus FTDC 3801.
The productions of functional foods containing probiotic bacteria such as lactobacilli are gaining high significance throughout the world. These bacteria enhance the microbial safety and offer organoleptic, technological, nutritional, and health benefits to the consumers. Among lactobacilli, L. acidophilus is a probiotic which widely used in health foods and fermented milk. As a major component of dairy starters, L. acidophilus has a generally recognized as safe status and a qualified presumption of safety status.
However, due to their short shelf life, researchers have come out with some effective solutions to extend the shelf life of probiotic. Most common solution is chilled at low temperature of ≤4 ± 1°C. This method allows probiotics to survive for several months even though the number of colony-forming units (CFU) is declining slowly. According to a review by Govender et al. [Citation1], the cell viability of probiotic should be in the range of 108–109 when it reaches gut to achieve the excellent health benefits. To overcome this weakness and extend a longer life span, drying method which converts living probiotics into powder form by dehydration process could be carried out [Citation2]. The cell viability of L. acidophilus decreased after undergoing the drying process. However, extreme temperature is the major cause of cell damage during the spray and freeze-drying. The temperature supply during the inlet of spray drying is high, ranging between 130°C and 170°C while the temperature in freeze drying is at −20°C to −80°C [Citation3]. Therefore, the protective factors for higher survival rates of cells such as lactic acid bacteria (LAB) through dehydration in extreme temperature should be considered.
Encapsulating method provides the basic matrix and protection to preserve the cells. Many studies have shown that encapsulating LAB in protective agents such as skim milk, sorbitol, and maltodextrin can reduce the number of deaths during the drying process. Protectant provides stability and protection against other inactivating mechanisms such as denaturation of sensitive proteins and decrease viability of many cell types. There are many types of protective agents, but sugar-based protective agents are widely used since they composed of polysaccharides and disaccharides with capability to protect cells by encapsulating them and replacing water molecules without disturbing cell membrane during drying process. The sugar-based protectant has given a high percentage of viability by 39% compared to skim milk, mannitol, and de Man Rogosa and Sharpe (MRS) broth. Mixture of other type protective agents with sugar are proven to give high viability by 62% [Citation4]. The aims of this study are to compare the effectiveness of different drying methods and to investigate the effects of adding a series of individual protectant for preserving L. acidophilus FTDC 3081 cells during spray and freeze-drying and storage at different temperatures. The high cell viability of L. acidophilus FTDC 3081 is the main target to develop a probiotic formulation for commercial application in human or animal diets.
Materials and methods
Materials
Lactobacillus acidophilus FTDC 3081 was obtained from the Bioprocess Technology Division, School of Industrial Technology, Universiti Sains Malaysia, Malaysia. MRS agar and MRS broth were purchased from HiMedia (India). The sugarcane molasses (food grade) was obtained from MSM Malaysia Holdings Berhad, Perai, Malaysia. Sulfuric acid (H2SO4) and potassium hydroxide (KOH) were supplied by QRëC, Malaysia. Glycerol, sucrose and yeast extract was purchased from Merck, Darmstadt, Germany. Phosphate buffer saline (PBS) was supplied by Sigma-Aldrich, United State. Skim milk powder, corn starch, and maltodextrin were purchased from local food shop, Malaysia.
Fermentation of Lactobacillus acidophilus FTDC 3081 in 10L bioreactor for cell biomass
The L. acidophilus FTDC 3081 strain was maintained in 30% (v/v) glycerol at −20°C. To activate the strain, 1 mL of glycerol stock of L. acidophilus FTDC 3081 was inoculated into sterile 100 mL MRS broth and incubated at 30°C for 24 h and used as inoculum for production stage.
L. acidophilus FTDC 3081 was produced in a 10 L bioreactor (Techfors, INFORS HT, Bottmingen-Basel, Switzerland) containing 5 L initial working volume of culture medium. The culture medium was composed of 10% (v/v) of pre-treated molasses which was added with 10 g/L of yeast extract and the pH was adjusted to 7 using 4.0 M KOH. Molasses was pre-treated with H2SO4 as described by Vidra, Tóth [Citation5]. About 2 mL of 20% (v/v) 6 M H2SO4 was added to 100 mL molasses and the mixture was heated in boiling water for 45 min and cooled at room temperature prior to use. The system was equipped with pH, temperature, and pO2 probes and four peristaltic pumps for the addition of alkali, acid, nutrients, salts, or antifoam solutions. The boiler was set up for steam sterilization at 121°C for 15 min. A 10% inoculum was added into the medium to start the fermentation with agitation at 150 rpm, 30°C. The culture was harvested when the growth reached a stationary phase after 24 h and centrifuged at 8,000 × g for 20 min at 4°C to obtain the cell pellets prior to adding protective media for drying purpose.
Preparation of protective media
The cell pellets collected after centrifugation were washed three times using the same volume ratio of sterile phosphate buffer saline (PBS) with pH 7.2 to remove the remaining molasses sticking to the cells. The cells were suspended with different protective agents at equal volume of initial culture and kept at 4°C prior to drying. Four different types of protective agents, namely skim milk, sucrose, maltodextrin, and corn starch at three different concentrations (5%, 10% and 20% w/v) were prepared in distilled water and autoclaved at 121°C for 15 min before mixing with the L. acidophilus FTDC 3081 [Citation4,Citation6,Citation7]. A control was prepared by resuspending the cell pellet with sterile-distilled water. A total of 78 sets of experiment were performed for both spray and freeze drying.
Microencapsulation process of L. acidophilus FTDC 3081 by spray drying
Spray drying were performed with a Buchi Mini spray dryer (B-290, New Castle, USA) at a fixed inlet air temperature of 130°C and flow dry air of 35 m3/h as described by Behboudi-Jobbehdar, Soukoulis [Citation8] with some modifications. The outlet temperature (70°C), pump flow rate (7 mL/min), and compressor air pressure (0.5 MPa) were kept constant throughout the drying process. Dried L. acidophilus FTDC 3081 formulations were collected from the cyclone separator vessel and placed in sealed falcon tubes.
Lyophilization process of L. acidophilus FTDC 3081 by freeze drying
Freeze-drying technique was carried out using a freeze dryer (Labconco, Missouri, USA) at the temperature of −51°C under vacuum condition for 24 h. After the freeze-drying cycle was completed, the falcon tubes were sealed.
Influence of storage temperature on the stability of formulated L. acidophilus FTDC 3081
After the completion of process, the spray and freeze-dried samples of L. acidophilus FTDC 3081 were stored at the three storage conditions of 4°C (fridge), room temperature (27°C) (shelf) and 40°C (extreme) for 1 month. The viability of the spray and freeze-dried samples for 1 month was determined through plate count technique and expressed as CFU/mL.
Enumeration and determination of cell viability after drying processes
After drying processes, the cultures were reconstituted to their original pre-dried volumes by adding sterile-distilled water and incubated at 37°C for 30 min prior to be spread on the agar. The mixture was diluted up to 10−10, and each dilution was spread on the MRS agar plate and incubated at 37°C, for 48 h. The number of colonies was determined using a colony counter and compared with the control for determination of cell viability (CFU/mL) and survivability (%). Enumeration of the bacteria was performed in triplicate. The survivability of the bacteria after the spray and freeze-drying process was calculated according to EquationEquation (1)(1)
(1) :
where N0 and N represent the number of viable bacteria (log CFU/mL) prior and after the spray-drying process.
Results
Effects of types and concentrations of protective agents on viability of L. acidophilus FTDC 3081 during drying
The effect of different concentration of protective agents on cell viability of L. acidophilus FTDC 3081 after spray drying and freeze-drying process is presented in . The cell viability during spray drying and freeze-drying processes was not affected by the types and concentration of the protective agents that added into the live cells. The cell viability for rehydrated L. acidophilus FTDC 3081 in all protective agents of maltodextrin, corn starch, skim milk, and sucrose were at range from 70% to 80% immediately after spray-drying and freeze-drying, in which the cell viability retained at the range of 109 to 1010 CFU/mL. However, the viable cell count for freeze-drying was ranging from 1.1 × 1010 to 2.9 × 1010 CFU/mL, 10 times higher than spray-drying (1.0 × 109 to 1.1 × 1010 CFU/mL. For non-protective additive, the cell viability substantially decreased from 1.03 × 1013 CFU/mL to 2.0 × 103 CFU/mL after spray-drying while the cell viability was slightly decreased to 7.8 × 108 CFU/mL after freeze-drying. The cell viability before spray and freeze-drying was 1.03 × 1013 CFU/mL.
Table 1. The viability of L. acidophilus FTDC 3081 formulated with 5%, 10% and 20% (w/v) of protective agents immediately after spray drying and freeze-drying processes.
Effect of spray and freeze-drying processes on bacterial survivability rate and moisture content
In spray-drying, maltodextrin at concentration of 10% (w/v) demonstrated the highest viability (1.1 × 1010 CFU/mL) and survivability (77.2%). The cell viability retained at the range of 1.0 to 8.0 × 109 CFU/mL in other protective agents. Sucrose as a protective agent only managed to protect the cells from the harsh spray-drying conditions with viability of approximately 69% ().
Figure 1. The survivability of L. acidophilus FTDC 3081 cells formulated with 5%, 10% and 20% (w/v) of protective agents immediately after spray-drying process. The survivability of L. acidophilus FTDC 3081 cells without addition of protective agent was 25.37%.
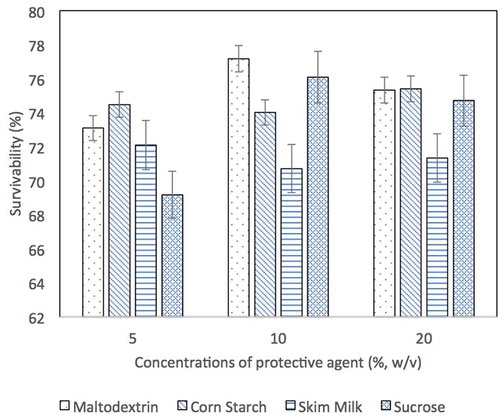
For freeze-dried cells, high cell viability (1.1 to 2.9 × 1010 CFU/mL) was obtained for all protective agents except 20% of corn starch which has lowest cell viability of 9.3 × 109 CFU/mL. As depicted in , the survivability of freeze-dried culture had >76% in all protective agents at different concentrations. Furthermore, freeze-dried culture without protective agent was maintained at 68% of survival rate which was 2.72 times higher than spray-dried culture without protective agent (25% of survival rate). This gives a good sign of freeze drying as one of effective drying method as compared to spray drying.
Figure 2. The survivability of L. acidophilus FTDC 3081 cells formulated with 5%, 10% and 20% (w/v) of protective agents immediately after freeze-drying process. The survivability of L. acidophilus FTDC 3081 cells without addition of protective agent was 68.33%.
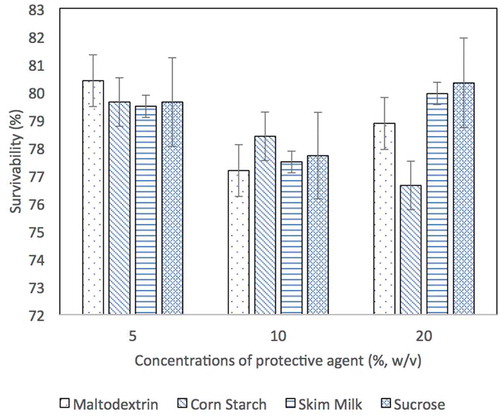
The concentration of protective agents affected the moisture content in the powder. In maltodextrin, dried products with a 20% maltodextrin presented a higher moisture content than those with a lower concentrations, which could have been due to the fact that increasing the material concentration, a faster wall formation is eased, restricting water steam diffusion from the inside of the liquid product to the surface during the process. Similar results were observed in other protective agents.
Effects of storage temperature on viability of spray and freeze-dried L. acidophilus FTDC 3081
shows effects of different storage temperatures on the cell viability of rehydrated spray-dried L. acidophilus in various protective agents for 1 month. In spray-drying study, storage temperature at 4°C appeared to be the most preferable for the stability of L. acidophilus followed by 25°C. Meanwhile, storage at 40°C showed the lowest stability of L. acidophilus FTDC 3081. Furthermore, the cells of L. acidophilus FTDC 3081 with the addition of maltodextrin or skim milk had higher cell viability compared to other protectants in all storage temperatures. The spray-dried sucrose showed lowest cell viability at 40°C where only 54% of cells survived after 1 month.
Table 2. The cell viability of spray-dried L. acidophilus FTDC 3081 during storage at 4°, 25°C, and 40°C for 30 days.
presents the viability of L. acidophilus FTDC 3081 with different protective agents after freeze-drying. After 1 month at 4°C and 25°C, the cell viability showed a slight decrease when the concentration of maltodextrin increased from 5% (w/v) to 10% (w/v). On the other hand, the cell viability of freeze-dried culture was sharply decreased in 20% (w/v) of maltodextrin. At 40°C, only maltodextrin provided the highest stability of L. acidophilus FTDC 3081 (108 CFU/mL) after 1 month. Cell viability decreased to 107 CFU/mL for corn starch and skim milk which was about 25% and 23% cell death, respectively, after 1 month at 40°C. Sucrose shows the lowest cell resistance at only 106 CFU/mL after 1 month at 40°C.
Table 3. The cell viability of freeze-dried Lactobacillus acidophilus FTDC 3081 during storage at 4°C, 25°C, and 40°C for 30 days.
Overall, maltodextrin shows as a good protective agent as it can preserve cells at different temperatures with high cell viability even after 1 month in both drying methods. The physical appearance of spray- and freeze-dried L. acidophilus FTDC 3081 powders using maltodextrin as protective agents were presented in . Although the cell viability of L. acidophilus FTDC 3081 was higher in freeze-dried sample, the spray-dried powder was finer than the freeze-dried powder. Therefore, maltodextrin was selected as the most suitable protectant in formulating spray-dried and freeze-dried L. acidophilus. FTDC 3081
Discussion
Spray and freeze-drying techniques are used with the purpose of removing moisture and prolonging the shelf-life of microorganisms. Probiotic cultures for food applications are frequently supplied in frozen or dried form, as either freeze-dried or spray-dried powders [Citation9]. The successful spray drying of Lactobacillus sp. and Bifidobacterium sp. has previously been reported [Citation10,Citation11]. However, most of the probiotic bacteria do not survive well during the spray and freeze-drying process due to temperature and osmotic extremes. When these drying methods are used for the preservation of potential probiotic cultures, much of their activity is typically lost after few weeks of storage at room temperature. This is associated with stress that is caused by temperature changes, phase changes, and drying, a combination of which tends to damage cell membranes and associated proteins. The cryo-injury is a sequential event occurring prior to dehydration inactivation of cells from the sublimation step of freeze drying, while thermal injury inactivation simultaneously occurs with dehydration inactivation during the falling drying rate period of spray drying. Although dehydration inactivation is obligatory for each drying process, the removal of water by the evaporation in spray drying and by the sublimation of water ice in freeze drying may exert quantitatively different dehydration stresses to cells. This difference may be a reason for the relatively high viability of freeze-dried cells despite formerly being subjected to the freezing stress. Furthermore, a high viability of the dried starter cultures cannot be assured solely by decreasing those inactivation stresses that occurred during drying. A low inactivation rate during storage is equally important or even more important if a very long storage period is required. Therefore, optimal storage conditions should also be taken into account [Citation12].
A wide range of protective agents have been developed to preserve the probiotics bacterial strains during the drying process such as spray-drying and freeze-drying which type of protective agents used in the drying will greatly affect on cell viability. Among these, carbohydrates are commonly used as protectants either individually or in combination with other additives. For example, the incorporation of protectants, such as trehalose [Citation13], nonfat milk solids and/or adonitol, growth-promoting factors including various probiotic/prebiotic combinations [Citation14] and granular starch [Citation15] have been shown to improve culture viability during the drying process and storage. In this study, higher cell viability of L. acidophilus FTDC 3081 was achieved using maltodextrin as protective agent in the powder formulation. This could be due to the characteristic of maltodextrins which has the ability to maintain water, prevent stickiness, lower viscosity, and better oxidative stability that makes maltodextrin as a good protective agent [Citation16,Citation17]. The cells’ stability is improved in the presence of carbohydrates such as maltodextrin during the drying process by glass formation and stabilization of the phospholipid cell membrane by hydrogen bond formation that generates a depression of the membrane phase transition temperature [Citation18]. It was also evident that the use of maltodextrin reduced the absorption of moisture by 50% unit (hygroscopicity) which prevents stickiness of powder formulation during storage. Physical and chemical characteristics of maltodextrin might have contributed to the increase of survivability of L. acidophilus FTDC 3081 during drying processes and storage more than the cell wall materials. The results demonstrated that the use of maltodextrin did not show a significant positive effect on the survivability of L. acidophilus FTDC 3081 during drying process in which the cell count decreased from 13 log CFU/mL to 10 log CFU/mL. However, after 30 days the presence of maltodextrin act as a better protective agent for the preservation of L. acidophilus FTDC 3081 compared to other protectants. Sucrose also showed higher cell viability of L. acidophilus FTDC 3081 after spray drying and freeze-drying processes. This is agreed to the study of Oslan, Halim [Citation19] who claimed that trehalose and sucrose showed significantly high survival rate (93–95%) of mutant Pasteurellamultocida B:2 immediately after freeze-drying. However, the results obtained from accelerated stability of L. acidophilus FTDC 3081 at 40°C for 30 days decreased by 42% and 51% of cell viability compared to other protective agents. Moreover, L. acidophilus FTDC 3081 in powder form using sucrose as protective agent turned into highly viscous liquid when it is stored at 40°C. Sucrose has a strong tendency to absorb the moisture from atmosphere which activates the cell metabolism during storage. The activation of cell metabolism resulted in the L. acidophilus FTDC 3081 entered to the death phase in a shorter time. This is one of the main reasons for the short shelf life of the bacteria even the formulation is in the form of powder. The use of disaccharides as cryoprotectants for Pantoea agglomerans strain CPA-2 resulted more than 60% of cell viability compared to monosaccharides (30–60% cells viability) after freeze-drying process.
In order to reduce the cost of probiotic production, molasses has been used as a carbon source however, the usage of molasses makes the drying process becomes difficult due to the presence of residual molasses. Naturally, molasses is sticky in nature, which attach to the cell surface and not possible to remove its residue by washing. Molasses contains the high hygroscopicity, thermoplasticity, and low glass transition temperature of low-molecular-weight substances that contribute to the stickiness problem. This resulted in higher interaction with water (increase of hygroscopicity), higher particle-particle stickiness (cohesion), and particle-wall surface stickiness (adhesion). The stickiness of molasses caused the probiotic powder stick on the inner surface of spray-drying chamber and nozzles, which leads to operating problems and low product yield. Similar issues were observed during the freeze-drying process as the residues of molasses become a viscous liquid and forming bubbles. Therefore, the freeze-dried L. acidophilus FTDC 3081 powder could not be stored at ambient temperature because it tends to absorb moisture from atmosphere which becomes sticky and rubbery. The increased moisture content of the dried powder could result in reduced stability during storage, with higher moisture values correlated with poor survivability [Citation20]. To minimize the stickiness problem, addition of high molecular weight drying aids to the feed solution thereby increasing the glass transition temperature and reducing the powders moisture gain (hygroscopicity) [Citation21,Citation22]. The most commonly used additives/drying aids used in spray drying includes maltodextrins, gum arabic, and starch due to their high solubility and less viscosity [Citation23].
The effect of storage temperature after drying is the main factor in determining the ideal condition to prolong the storage of the probiotic bacteria with higher cell viability. One of the observations highlighted in this study was the shelf life of spray and freeze-dried products which highly depended on the storage temperature. The storage stability of freeze-dried cultures are higher at low temperature (e.g. 4°C) [Citation24]. In the study of Costa, Usall [Citation25], the viability of Pantoea agglomerans decreased by 0.5 log after 90 days of storage at 4°C compared to 25°C, which decreased by 3 logs after 28 days. Other studies that evaluated L. acidophilus viability using different encapsulating agents during refrigerated storage showed that the encapsulation of probiotic bacteria by spray drying and storage at refrigerated temperature improved the storage stability/cells viability [Citation8,Citation26–Citation29]. In general, a minimum concentration of viable L. acidophilus above 6 log CFU/g or mL of product is needed at the time of consumption to have health benefits in the human body [Citation30,Citation31]. In this study, the cell counts from all the L. acidophilus FTDC 3081 powder formulation stored at different temperatures meet the guideline for the usage of probiotic products (at least 6 log CFU/g), except the formulation with sucrose during spray-drying process.
Formulation of L. acidophilus FTDC 3081 with protective agents produced good preservation which can enhance cell viability after spray and freeze-drying processes. Addition of filler materials is required when the molasses is used as fermentation substrate to reduce the stickiness during the drying process. Maltodextrin acts as a better protective agent to L. acidophilus FTDC 3081, resulting in higher cell viability/storage stability, particularly in comparison with the other protectants. The stability of dried L. acidophilus FTDC 3081 is higher at low-temperature storage (4°C). In future study, moisture content determination and optimization of the inlet and outlet drying temperature during spray drying could be performed to improve the survivability of dried L. acidophilus FTDC 3081.
Authors’ contributions
Hock Wei Tang carried out the experiment and wrote the manuscript with support from Joo Shun Tan, Hui Suan Ng, and Yew Joon Tam. Sahar Abbasiliasi and Paramasivam Murugan conceived of the presented idea and supervised the project.
Disclosure statement
No potential conflict of interest was reported by the authors.
Additional information
Funding
References
- Govender M, Choonara YE, Kumar P, et al. A review of the advancements in probiotic delivery: conventional vs. non-conventional formulations for intestinal flora supplementation. AAPS PharmSciTech. 2014;15(1):29–43.
- Nireesha GR, Divya L, Sowmya C, et al. Lyophilization/freeze drying - an review. Int J Novel Trends Pharm Sci. 2013;3:2277-2782.
- Madhu AN, Awasthi SP, Reddy KBPK, et al. Impact of freeze and spray drying on the retention of probiotic properties of lactobacillus fermentum: an in vitro evaluation model. Int J Microbiol Res. 2011;2(3):243–251.
- Berner D, Viernstein H. Effect of protective agents on the viability of Lactococcus lactis subjected to freeze-thawing and freeze-drying. Sci Pharm. 2006;74(3):137–149.
- Vidra A, Tóth AJ, Németh Á. Lactic acid production from cane molasses. Waste Treat Recovery. 2017;2(1):13–16. .
- Champagne CP, Fustier P. Microencapsulation for delivery of probiotics and other ingredients in functional dairy products. Functional Dairy Products. 2007;404–426.
- Zhan Y, Xu Q, Yang MM, et al. Screening of freeze-dried protective agents for the formulation of biocontrol strains, Bacillus cereus AR156, Burkholderia vietnamiensis B418 and Pantoea agglomerans 2Re40. Lett Appl Microbiol. 2012 Jan;54(1):10–17. .
- Behboudi-Jobbehdar S, Soukoulis C, Yonekura L, et al. Optimization of spray-drying process conditions for the production of maximally viable microencapsulated L. acidophilus NCIMB 701748. Drying Technol. 2013;31(11):1274–1283.
- Holzapfel WH, Haberer P, Geisen R, et al. Taxonomy and important features of probiotic microorganisms in food and nutrition. Am J Clin Nutr. 2001;73(2):365s–373s.
- De Castro-Cislaghi FP, Carina Dos Reis ES, Fritzen-Freire CB, et al. Bifidobacterium Bb-12 microencapsulated by spray drying with whey: survival under simulated gastrointestinal conditions, tolerance to NaCl, and viability during storage. J Food Eng. 2012;113(2):186–193.
- Shokri Z, Fazeli MR, Ardjmand M, et al. Factors affecting viability of Bifidobacterium bifidum during spray drying. DARU J Pharma Sci. 2015;23(1):7.
- Santivarangkna C, Kulozik U, Foerst P. Inactivation mechanisms of lactic acid starter cultures preserved by drying processes. J Appl Microbiol. 2008;105(1):1–13.
- Conrad PB, Miller DP, Cielenski PR, et al. Stabilization and preservation of Lactobacillus acidophilus in saccharide matrices. Cryobiology. 2000;41(1):17–24.
- Desmond C, Ross R, O’callaghan E, et al. Improved survival of Lactobacillus paracasei NFBC 338 in spray‐dried powders containing gum acacia. J Appl Microbiol. 2002;93(6):1003–1011.
- Crittenden R, Laitila A, Forssell P, et al. Adhesion of bifidobacteria to granular starch and its implications in probiotic technologies. Appl Environ Microbiol. 2001;67(8):3469–3475.
- Parikh A, Agarwal S, Raut K. A review on applications of maltodextrin in pharmaceutical industry. Int J Pharm Biol Sci. 2014;4(4):67–74.
- Savedboworn W, Kerdwan N, Sakorn A, et al. Role of protective agents on the viability of probiotic Lactobacillus plantarum during freeze drying and subsequent storage. Int Food Res J. 2017;24(2):787–792.
- Schutyser MA, Perdana J, Boom RM. Single droplet drying for optimal spray drying of enzymes and probiotics. Trends Food SciTechnol. 2012;27(2):73–82.
- Oslan SNH, Halim M, Ramle NA, et al. Improved stability of live attenuated vaccine gdhA derivative Pasteurella multocida B: 2 by freeze drying method for use as animal vaccine. Cryobiology. 2017;79:1–8.
- Mis-Solval KE, Jiang N, Yuan M, et al. The effect of the ultra-high-pressure homogenization of protein encapsulants on the survivability of probiotic cultures after spray drying. Foods. 2019;8(12):689.
- Bhandari B, Howes T. Implication of glass transition for the drying and stability of dried foods. J Food Eng. 1999;40(1–2):71–79.
- Hernández-Carranza P, López-Malo A, Jiménez-Munguía M-T. Microencapsulation quality and efficiency of Lactobacillus casei by spray drying using maltodextrin and vegetable extracts. J Food Res. 2014;3(1):61.
- Muzaffar K, Nayik GA, Kumar P. Stickiness problem associated with spray drying of sugar and acid rich foods: a mini review. J Nutr Food Sci. 2015;S12:1.
- Santivarangkna C, Kulozik U, Foerst P. Alternative drying processes for the industrial preservation of lactic acid starter cultures. Biotechnol Prog. 2007;23(2):302–315.
- Costa E, Usall J, Teixido N, et al. Water activity, temperature, and pH effects on growth of the biocontrol agent Pantoea agglomerans CPA-2. Can J Microbiol. 2002;48(12):1082–1088.
- Yonekura L, Sun H, Soukoulis C, et al. Microencapsulation of Lactobacillus acidophilus NCIMB 701748 by spray drying: impact of soluble fibres on storage stability and survival after in vitro digestion. J Funct Foods. 2013;6(100):205–214.
- Maciel G, Chaves K, Grosso C, et al. Microencapsulation of Lactobacillus acidophilus La-5 by spray-drying using sweet whey and skim milk as encapsulating materials. J Dairy Sci. 2014;97(4):1991–1998.
- Pispan S, Hewitt C, Stapley A. Comparison of cell survival rates of E. coli K12 and L. acidophilus undergoing spray drying. Food Bioprod Process. 2013;91(4):362–369.
- Riveros B, Ferrer J, Borquez R. Spray drying of a vaginal probiotic strain of Lactobacillus acidophilus. Drying Technol. 2009;27(1):123–132.
- Zhao R, Sun J, Torley P, et al. Measurement of particle diameter of Lactobacillus acidophilus microcapsule by spray drying and analysis on its microstructure. World J Microbiol Biotechnol. 2008;24(8):1349–1354.
- Machado TADG, de Oliveira MEG, Campos MIF, et al. Impact of honey on quality characteristics of goat yogurt containing probiotic Lactobacillus acidophilus. LWT-Food Science andTechnology. 2017;80:221–229.