ABSTRACT
Awa-bancha is a post-fermented tea produced in Naka and Kamikatsu, Tokushima, Japan. We investigated the lactic acid bacteria in each stage of production of Awa-bancha and evaluated the relationships with the components. Lactic acid bacteria were isolated from tea leaves cultured with de Man, Rogosa, and Sharpe (MRS) agar plates, and the species were identified by homology of the 16 S rRNA gene and multiplex polymerase chain reaction (PCR) of the recA gene to distinguish the Lactobacillus plantarum group. As a result, a variety of species were isolated from the raw tea leaves, and Lactobacillus pentosus was isolated most frequently after anaerobic fermentation. Regarding the tea leaf components, organic acids, such as lactic acid, increased, free amino acids decreased, and catechins changed owing to anaerobic fermentation. Our results suggest that the microbial flora mainly composed of L. pentosus is important in the anaerobic fermentation process for flavor formation of Awa-bancha.
Graphical abstract
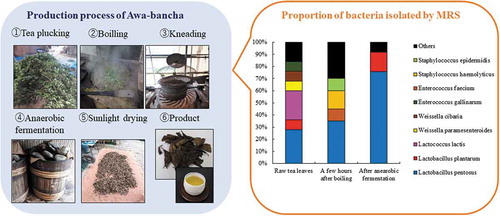
The dominant species of bacteria involved in tea fermentation differ depending on the production process.
Tea is classified into non-fermented tea, semi-fermented tea, fermented tea, and post-fermented tea according to the production method [Citation1]. Non-fermented tea is green tea that is steamed and dried. Semi-fermented tea includes oolong tea and fermented tea includes black tea, which are produced by changing the components using tea leaf oxidase. Post-fermented teas in East Asia include Pu’er tea in China, Miang in Thailand, and Lahpet in Myanmar, which are produced by fermentation with microorganisms [Citation2,Citation3]. There are four types of post-fermented teas in Japan, including Awa-bancha in Tokushima, Ishizuchi-kurocha in Ehime, Goishi-cha in Kochi, and Batabata-cha in Toyama [Citation4–Citation7]. Each post-fermented tea has a different production process. Awa-bancha, Miang, and Lahpet are produced by anaerobic fermentation mainly by lactic acid bacteria, and Batabata-cha and Pu’er tea are produced by aerobic fermentation mainly by fungi. Ishizuchi-kurocha and Goishi-cha are produced by a two-stage process of aerobic fermentation followed by anaerobic fermentation. The anaerobic fermentation process of post-fermented tea involves lactic acid bacteria, and this creates a unique flavor with sourness. Of these, Awa-bancha is produced in Kamikatsu and Naka in Tokushima. There are various theories as to whether the production method of Awa-bancha was naturally occurring or transmitted from the continent, but the origin is unknown because of insufficient literature [Citation8]. For production of Awa-bancha, Yamacha and Yabukita tea leaves are plucked from July to August and boiled 5 to 30 min in a kettle. After boiling, the leaves are kneaded with a kneading machine, leading to scratched leaves. The tea leaves are then packed in barrels to prevent air from entering. After placing stones over the leaves, add boiled soup of the tea leaves and the leaves are fermented anaerobically for 2 to 4 weeks. The tea leaves are then removed and sun-dried to complete the process (). The production process of Awa-bancha varies depending on the producer, such as how the tea leaves are boiled, the weight of the stones, and the period of anaerobic fermentation. In addition, Awa-bancha is produced with lactic acid bacteria present in the environment. Therefore, the lactic acid bacteria involved in anaerobic fermentation may vary depending on the production area. The production process and location affect the lactic acid bacteria and microflora involved in fermentation, and Awa-bancha tends to have different flavors among producers. The anaerobic fermentation process of Awa-bancha has been reported to involve lactic acid bacteria such as Lactobacillus plantarum and Lactobacillus pentosus [Citation9,Citation10]. However, these reports are investigations of strains isolated by culture methods, and the microflora and the dominant bacteria during fermentation are unknown. As for the components of Awa-bancha, it has been reported that lactic acid increases owing to anaerobic fermentation [Citation11]. However, the relationships between the components that characterize Awa-bancha and the microorganisms mainly composed of lactic acid bacteria involved in fermentation have not been investigated. It is necessary to understand these relationships in order to produce Awa-bancha with stable quality. In this study, we analyzed the relationships between the lactic acid bacteria and microbial genes involved in the fermentation of Awa-bancha and the components that affect flavor formation.
Figure 1. The production area and production process of Awa-bancha.
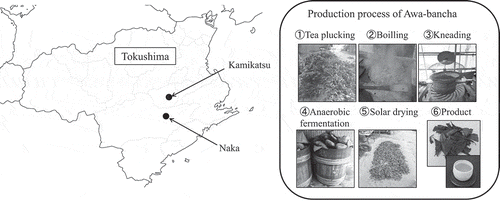
Materials and methods
Tea leaves
In 2018, we collected tea leaves from each production process (raw tea leaves, during boiling, a few hours after boiling, and after anaerobic fermentation) of Awa-bancha in Kamikatsu and Naka (). These were collected from seven producers (A, B, C, D, E, F, and G) and three times from different lots from producer A (A1, A2, and A3). The samples were used to determine viable counts and isolate lactic acid bacteria within 24 h. Samples used for 16 S rRNA-based metagenomics analysis were stored at 4°C until use, and those used for component analysis were stored at −20°C and lyophilized before use.
Table 1. Samples collected from different producer.
Viable bacteria count
Sterilized phosphate buffered saline (PBS; FUJIFILM Wako Pure Chemical Corporation, Osaka, Japan) and wet tea leaves from each production process (raw tea leaves, during boiling, a few hours after boiling, and after anaerobic fermentation) were mixed to a concentration of 0.1 mg/mL and stirred well with a vortex mixer. The suspension was serially diluted with sterile PBS and smeared on standard methods agar “DAIGO” (Nihon Pharmaceutical Co., Ltd., Tokyo, Japan) or de Man, Rogosa, and Sharpe (MRS) (Merck KGaA, Darmstadt, Land Hessen, Germany) medium or yeast malt (YM) medium containing 2% Bacto agar (Becton, Dickinson and Company, Franklin Lakes, New Jersey, USA). The YM medium was prepared with a composition of 0.3% Bacto Yeast Extract (Becton, Dickinson and Company), 0.5% HIPOLYPEPTON (Nihon Pharmaceutical), 0.3% Bacto Malt Extract (Becton, Dickinson and Company) and 1% glucose (KANTO CHEMICAL CO., INC., Tokyo, Japan). Standard agar plates were aerobically cultured at 37°C for 2 days. MRS agar plates were cultivated at 37°C for 2 days in an AnaeroPack-Anaero anaerobic gas chamber (Mitsubishi Gas Chemical Co., Inc., Tokyo, Japan). YM agar plates was cultured at 30°C for 5 days. Bacterial estimates were determined by colony formation per gram of tea leaves.
16 S rRNA-based metagenomics analysis
The DNA was extracted from raw tea leaves and tea leaves after anaerobic fermentation using an Extrap Soil DNA Kit Plus ver. 2 (NIPPON STEEL & SUMIKIN Eco-Tech Corporation, Tokyo, Japan) according to the instruction manual. Using this DNA as a template, 16 S rRNA V3-V4 region was amplified using Life ECO ver2. (Nippon Genetics Co., Ltd. Tokyo, Japan). Polymerase chain reaction (PCR) amplification was performed using KAPA HiFi HotStart ReadyMix (Nippon Genetics), forward primer 341 F (5ʹ-CCTACGGGNGGCWGCAG-3ʹ), reverse primer 805 R (5ʹ-GACTACHVGGGTATCTAATCC-3ʹ) [Citation12]. The reaction system was prepared with a total volume of 25 μL containing 2.5 μL of template DNA, 1 µM of primers, 12.5 μL of 2 × KAPA HiFi HotStart ReadyMix. All the primers were synthesized by Fasmac Co., Ltd. (Kanagawa, Japan).The thermal cycler reaction conditions were as follows: denaturation at 95°C for 3 min; 25 cycles of 95°C for 30 s, 55°C for 30 s, and 72°C for 50 s; and 72°C for 5 min. The sequence was performed using the miseq system (Illumina, Inc., San Diego, California, USA). After the sequencing, sample data were sorted for each target sequence using Claident (https://www.claident.org/), a software package for DNA barcoding. The primer sequence and target sequence were removed, and the sample was sorted based on this information. The quality trimming tool Sickle (https://github.com/najoshi/sickle) was used to remove low-quality sequences with a Q value of 25 or less. The pair end reads were connected by FLASH (https://ccb.jhu.edu/software/FLASH/), a software tool that connects the pair end reads while considering the overlap region. Finally, BLAST+ 2.7.1 (ftp://ftp.ncbi.nlm.nih.gov/blast/executables/blast+/2.7.1/) was used to perform homology searches by conducting local blast.
Isolation and identification of bacteria from Awa-bancha using MRS agar plates
The wet tea leaves in each production process (raw tea leaves, a few hours after boiling, and after anaerobic fermentation) were added to sterilized PBS to a concentration of 0.1 mg/mL. The suspension was serially diluted with sterile PBS, spread on MRS agar plates, and anaerobically cultured at 37°C for 2 days. The isolated colonies were picked and cultured at 37°C for 2 days in 10 mL of MRS broth dispensed into screw-top test tubes. The stock culture was stored at −80°C in MRS broth containing 30% glycerol. The bacterial species were identified by the homology of the base sequence of the 16 S ribosomal RNA gene. Bacterial DNA was extracted using a DNeasy Blood & Tissue Kit (Qiagen, Hilden, Germany). The culture solution was collected from MRS broth by centrifugation at 10,000 rpm for 10 min. This pellet was suspended in the lysis solution attached to the kit and sonicated for 5 min using Bioraptor (BM Equipment Co., Ltd., Tokyo, Japan). DNA was then extracted according to the kit protocol. PCR amplification was performed using a TaKaRa PCR Thermal Cycler (Takara Bio Inc., Shiga, Japan) with the bacterial universal primers 27 F (5ʹ-AGAGTTTGATCCTGGCTCAG-3ʹ), 1492 R (5ʹ-GGTTACCTTGTTACGACTT-3ʹ) [Citation13], and TaKaRa Ex Taq DNA polymerase (Takara Bio). The PCR amplifications were performed with a total volume of 50 μL containing 1 μL of template DNA, 1 μM of primers, 5 μL of PCR buffer, 0.2 mM of each deoxynucleotide triphosphate, and 1.25 U Ex Taq polymerase. All the primers were synthesized by Eurofins Genomics K.K. (Tokyo, Japan). The amplification cycles were performed as follows: denaturation at 95°C for 3 min; 40 cycles of 95°C for 30 s, 55°C for 55 s, and 72°C for 1 min; and 72°C for 10 min. The amplified PCR product was sequenced with the sequencing primer LAB-SeqF (5ʹ-TCCTGGCTCAGGACGAACGCT-3ʹ). The sequence was determined by Macrogen Japan Corp. (Kyoto, Japan). The bacterial species were identified by the Standard Nucleotide BLAST of the National Center for Biotechnology Information (https://blast.ncbi.nlm.nih.gov/Blast.cgi). In addition, multiplex PCR was performed on the recA gene to distinguish L. plantarum-related species [Citation14]. The following primers were used: paraF (5ʹ-GTCACAGGCATTACGAAAAC-3ʹ), pentF (5ʹ-CAGTGGCGCGGTTGATATC-3ʹ), planF (5ʹ-CCGTTTATGCGGAACACCTA-3ʹ), and pREV (5ʹ-TCGGGATTACCAAACATCAC-3ʹ). The PCR amplifications were performed with a total volume of 50 μL containing 1 μL of template DNA, 1.5 mM MgCl2, 0.25 μM of primers paraF, pentF, and pREV, 0.12 µM planF, 0.2 mM of each deoxynucleotide triphosphate, and 1.25 U of Ex Taq polymerase. The amplification cycles were performed as follows: denaturation at 94°C for 3 min; 30 cycles of 94°C for 30 s, 56°C for 10 s, and 72°C for 30 s; and 72°C for 5 min.
Lactic acid bacteria growth test
L. pentosus NBRC 106467 T and L. pentosus NBRC 12011 and L.plantarum subsp. plantarum NBRC15891 T were purchased from the Biological Resource Center of the National Institute of Technology and Evaluation (Tokyo, Japan). The green tea extract containing 1% glucose was prepared by adding 1.5 mg/mL green tea leaves and 10 mg/mL glucose to hot water and stirring for 30 min. The supernatant was then sterilized through a 0.22 μm filter (Toyo Roshi Kaisha, Ltd., Tokyo, Japan) and transferred to a screw-top test tube. The lactic acid bacteria suspension cultured in MRS broth was added to a screw-top test tube to 1% (v/v) and cultured at 37°C for 2 days, and then the optical density (OD600) was measured by a UV-visible spectrophotometer (UV-1200, SHIMADZU CORPORATION, Kyoto, Japan).
Component analysis of tea leaves
The raw and post-anaerobic fermentation tea leaves of Awa-bancha were powdered using a Milcer IFM-700 G (Iwatani Corporation, Tokyo, Japan). Tea leaves were added to water to a concentration of 50 mg/mL, and after stirring for 30 min, the pH was measured with a LAQUA pH meter (HORIBA Ltd., Kyoto, Japan). Organic acids were analyzed by an organic acid analysis system (LC-800 series, JASCO Corporation, Tokyo, Japan). Water was added to the tea leaves to a concentration of 100 mg/mL, and the mixture was shaken for 1 h and filtered through a 0.45 μm filter (Toyo Roshi Kaisha) and then used for analysis. A Shodex Ionpak C-811 (8 mm × 500 mm; Showa Denko K.K., Tokyo, Japan) was used as the column at 60°C. The flow rate ratio was 1.0 mM/min with 3.0 mM HClO4 (FUJIFILM Wako) as the eluent and 1.5 mM/min with 0.2 mM bromothymol blue (KANTO CHEMICAL) in 15.0 mM Na2HPO4 (KANTO CHEMICAL) as the reagent. Organic acids were detected at a wavelength of 445 nm. Free amino acids were analyzed using a fully automatic amino acid analyzer (JLC-500 V/2, JEOL Ltd., Tokyo, Japan) [Citation15]. Tea leaves and 10% sulfosalicylic acid (KANTO CHEMICAL) were mixed to a concentration of 100 mg/mL and shaken at 25°C for approximately 2 h, and then the supernatant was diluted with lithium citrate buffer (pH of 2.98) and filtered through a 0.45 μm filter. Catechins and caffeine were analyzed by a high-performance liquid chromatograph (Waters Corporation, Milford, Connecticut, USA) [Citation16]. A mixture of equal amounts of acetonitrile (FUJIFILM Wako) and water was mixed with tea leaves to a concentration of 5 mg/mL. The mixture was shaken for 40 min, and then the supernatant was filtered through a 0.45 μm filter and used for analysis. The column was a CAPCELL PAK C18 UG120 (S3) (4.6 mm × 100 mm; Osaka Soda Co., Ltd., Osaka, Japan). The column temperature was 40°C. The mobile phase was 0.5% (v/v) phosphoric acid/methanol (FUJIFILM Wako) = 82/18 and the flow rate was 0.8 mL/min. The detection wavelength was 280 nm. Epigallocatechin (EGC), catechin (C), epigallocatechin gallate (EGCg), epicatechin (EC), epicatechin gallate (ECg) (Mitsui Norin Co., Ltd., Tokyo, Japan), and caffeine (FUJIFILM Wako) were used as standard reagents.
Evaluation of degranulation inhibition by cultured cells
The evaluation of degranulation inhibition was performed according to the method of Watanabe et al. using RBL-2H3 cells [Citation17]. Sterile water was added to powdered tea leaves (raw tea leaves and leaves after anaerobic fermentation) to a concentration of 50 mg/mL, and the mixture was incubated at 4°C for 24 h and used for the experiment. RBL-2H3 cells were obtained from the JCRB Cell Bank (National Institutes of Biomedical Innovation, Health and Nutrition., Osaka, Japan), and 10% heat-inactivated fetal bovine serum (FBS; HyClone Laboratories, GE Healthcare, Chicago, Illinois, USA) and 250 ng/mL of amphotericin B (antibiotics mixture; Nacalai Tesque Inc., Kyoto, Japan) were added to the culture in minimum essential medium (MEM; Gibco, Thermo Fisher Scientific Inc.). Cells were planted in a 24 well plate at a concentration of 2.5 × 105 cells/mL and cultured for 1 day at 37°C in a 5% CO2 atmosphere. Thereafter, the culture medium was replaced with 10% FBS MEM containing 50 ng/mL of monoclonal mouse IgE anti-dinitrophenyl (YAMASA CORPORATION, Choshi, Japan) and incubated for 2 h. The cells were washed with modified Tyrode’s buffer (MT buffer: 137.0 mM NaCl, 2.7 mM KCl, 1.8 mM CaCl2, 1.0 mM MgCl2, 5.6 mM glucose, 20.0 mM HEPES, and 0.1% (w/v) bovine serum albumin (BSA); pH of 7.4), and then MT buffer containing tea leaf extract to a final dilution 50 times was added and incubated at 37°C for 10 min. Next, 2, 4-dinitrophenylated BSA (Cosmo Bio Co., Ltd., Tokyo, Japan) was added to a final concentration of 50 ng/mL and further incubated for 30 min. The supernatant was collected and the cells were further lysed with MT buffer containing 0.1% Triton X-100 (MP Biomedicals, LLC, Santa Ana, California, USA). A 96 -wellplate with 50 μL of supernatant or cell lysate and 100 μL of 0.1 M citrate buffer (pH of 4.5) containing 3.3 mM p-nitrophenyl-2-acetamido-2-deoxy-β-D-glucopyranoside (FUJIFILM Wako) was incubated at 37°C for 25 min. The reaction was stopped by adding 100 μL of glycine buffer (pH of 10.4), and the absorbance at 405 nm was measured using a microplate reader. The release rate was calculated as the absorbance of supernatant/(absorbance of supernatant + absorbance of cell lysate) × 100.
Evaluation of sugar utilization of isolated L.pentosus
Utilization of the sugars by isolated lactic acid bacteria was evaluated by API50CH (BioMérieux Inc., Marcy-L’Etoile, France). Lactic acid bacteria were precultured in MRS broth and collected by centrifugation at 5,400 g for 10 min. The pellet was washed once with PBS and then suspended in API50CHL medium (BioMérieux) to the same turbidity as McFarland No. 2. The suspension was placed in a test tube of API50CH, covered with mineral oil, and cultured at 37°C for 2 days. Sugar utilization was determined by a change in the color of the medium.
Results
Viable bacteria count
Viable bacteria in each tea production process were counted using standard agar plates, YM agar plates, and MRS agar plates (). The viable bacteria count of the raw tea leaves per gram of wet weight was approximately 5.4 × 105 for the standard agar plates, 3.7 × 106 for the YM agar plates, and 2.8 × 104 for the MRS agar plates. No viable bacteria were detected from the tea leaves in the boiling process. However, the viable bacteria counts of tea leaves left for a few hours after boiling were 2.1 × 104 for the standard agar plates, 6.1 × 104 for the YM agar plates, and 1.8 × 102 for the MRS agar plates. The number of viable bacteria in the tea leaves after anaerobic fermentation increased significantly, and were 1.2 × 108 for the standard agar plates, 1.6 × 108 for the YM agar plates, and 1.4 × 108 for the MRS agar plates.
Figure 2. Number of bacteria in each production process.
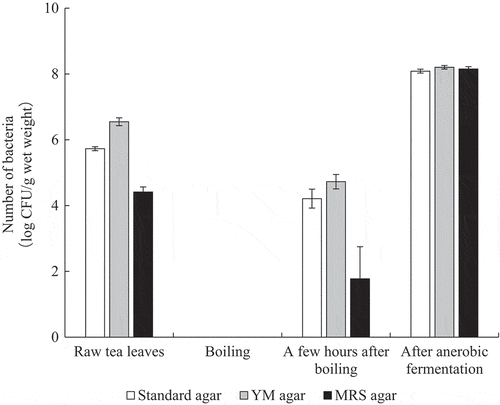
16 S rRNA-based metagenomics analysis
Metagenomics analysis based on 16 S rRNA genes was performed on raw tea leaves and tea leaves after anaerobic fermentation. As for the raw tea leaves, Aerosakkonema funiforme accounted for 49% to 74% for all producers, followed by Rhodoligotrophos jinshengii accounting for 9% to 14% ()). In contrast, the genus Lactobacillus, which is a lactic acid bacterium, accounted for only 1% to 3%. In the tea leaves after anaerobic fermentation, the genus Lactobacillus was dominant and accounted for 42–78% for all producers ()), followed by Klebsiella, which accounted for 7–17%. At the species level, L. plantarum was the most dominant after anaerobic fermentation, and accounted for 31–70%, followed by Lactobacillus vaccinostercus (0–21%), Lactobacillus paracollinoides (0–12%), and Klebsiella variicola (2–9%). It was shown that the bacterial flora of tea leaves was changed significantly by anaerobic fermentation.
Isolation and identification of bacteria from Awa-bancha using MRS agar plates
A total of 119 strains of bacteria were isolated with MRS agar plates, namely 25 strains from the raw tea leaves, 20 strains from the tea leaves left for a few hours after boiling, and 74 strains from the tea leaves after anaerobic fermentation. Lactic acid bacteria isolated from Awa-bancha were identified by the homology of the base sequence of 16 S rRNA. In addition, because L. plantarum is difficult to distinguish by the homology of 16 S rRNA, the exact bacterial species was identified by multiplex PCR for the recA gene. In multiplex PCR for the recA gene, the PCR amplification product was expected to be 318 bp for L. plantarum, 218 bp for L. pentosus, and 107 bp for Lactobacillus paraplantarum. The results are shown in . From the raw tea leaves, L. pentosus, Lactococcus lactis, Weissella oryzae, Weissella cibaria, and Enterococcus gallinarum were isolated, and the bacterial species were diverse. From the tea leaves left for a few hours after boiling, L. pentosus, Enterococcus faecium, L. lactis, Lactobacillus coryniformis, Staphylococcus haemolyticus, Staphylococcus hominis, and Staphylococcus epidermidis were isolated. For most producers, L. pentosus was most frequently isolated from tea leaves after anaerobic fermentation, followed by L. plantarum, L. coryniformis, Lactobacillus pantheris, and Lactobacillus brevis. The ratios of bacteria separated by MRS agar plates from each production process by genus and species are presented in . At the genus level, Lactobacillus accounted for 60% in raw tea leaves, followed by Weissella at 20%. In the tea leaves left for a few hours after boiling, Lactobacillus accounted for 50%, followed by Staphylococcus, which accounted for 30%. On the other hand, Lactobacillus accounted for 100% in tea leaves after anaerobic fermentation. At the species level, L. pentosus accounted for 28% in the raw tea leaves, followed by L. lactis at 24%. L. pentosus accounted for 35% in the tea leaves left for a few hours after boiling, followed by S. haemolyticus at 15%. In tea leaves after anaerobic fermentation, L. pentosus accounted for 76% and L. plantarum accounted for 16%.
Table 2. Number of bacteria isolated by MRS agar plates in each product process.
Lactic acid bacteria growth test
The isolated lactic acid bacteria were cultured in a green tea extract containing 1% glucose at 37°C for 1 day, and then OD600 was measured. L. pentosus and L. plantarum grew well, but the other species did not ().
Table 3. Growth of lactic acid bacteria isolated on green tea extract medium containing 1% glucose.
Evaluation of sugar utilization of L.pentosus isolated
The sugar utilization of isolated lactic acid bacteria was examined using API50CH (). The isolated lactic acid bacteria had some differences in sugar utilization compared with that of L. pentosus NBRC 106467 T and L. pentosus NBRC 12011. In particular, the isolated lactic acid bacteria used methyl-αD-glucopyranoside, but L. pentosus NBRC 106467 T and L. pentosus NBRC 12011 did not.
Table 4. Sugar utilization of Lactobacillus pentosus isolated.
Component analysis of tea leaves
Components of the raw tea leaves and tea leaves after anaerobic fermentation were analyzed for each producer. For almost all producers, the pH of the tea leaves decreased because of anaerobic fermentation. The organic acid analysis results showed that oxalic acid and malic acid decreased owing to anaerobic fermentation (). However, lactic acid and acetic acid increased considerably, and succinic acid also increased. Free amino acids tended to decrease overall after anaerobic fermentation (). In addition, gamma-aminobutyric acid (GABA) increased in the tea leaves of all producers owing to anaerobic fermentation. In the analysis of catechins, inspite of variation, the overall trend was that EGC increased and EGCg decreased after anaerobic fermentation (). The caffeine contained in tea leaves also tended to decrease after anaerobic fermentation.
Table 5. Contents of organic acids and pH in Awa-bancha.
Table 6. Contents of free amino acids in Awa-bancha.
Table 7. Contents of caffeine and catechins in Awa-bancha.
Evaluation of degranulation inhibition by cultured cells
As a physiological activity of Awa-bancha, RBL-2H3 cells were used to evaluate the degranulation inhibitory effect. Although there were variations among producers, degranulation tended to be suppressed in tea leaves after anaerobic fermentation compared with that in raw tea leaves ().
Figure 5. Evaluation of degranulation by the tea extract for RBL-2H3 cells.
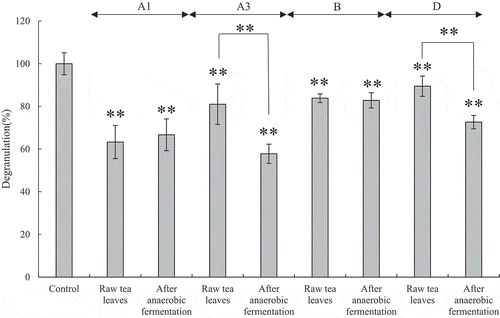
Discussion
In this study, we analyzed the genes of lactic acid bacteria for each production process of Awa-bancha and investigated the relationship with changes in the components of tea leaves. For production of Awa-bancha, the tea leaves ware harvested and then boiled in a kettle (). On a standard agar plates, a viable count of approximately 100,000 cfu/g was detected in the raw tea leaves. No colonies were detected in the tea leaves in the boiling process, but approximately 10,000 cfu/g was detected in the tea leaves left for several hours in the workplace. The number of viable bacteria in the tea leaves after anaerobic fermentation increased rapidly to 100 million cfu/g. Moreover, when cultured on YM agar plates, the colonies in the raw tea leaves reached approximately 1 million cfu/g, but increased to 100 million cfu/g after anaerobic fermentation. In addition, when cultured on MRS agar plates, approximately 10,000 cfu/g was detected in the raw tea leaves, which was approximately 10% of the number of viable bacteria. In addition, the viable count increased significantly to 100 million cfu/g after anaerobic fermentation. It was suggested that lactic acid bacteria and yeast coexist in the anaerobic fermentation process of Awa-bancha. In addition, viable bacteria were detected in the tea leaves a few hours after boiling. This might have been due to the possibility of bacteria from the air, tools, or workers infecting the tea leaves, or the possibility that a small number of bacteria that survived the boiling process grew. As per 16 S rRNA-based metagenomics analysis, A. funiforme was dominant for all producers of tea leaves. A. funiforme is a filamentous cyanobacteria that is abundant in the environment, but because its DNA is similar to chloroplast DNA, the chloroplast DNA of tea leaves may be determined as A. funiforme [Citation18]. The next most dominant bacteria was R. jinshengii. R. jinshengii has been reported to be isolated from activated sludge and grow at a pH of 5–10 [Citation19]. In the tea leaves after anaerobic fermentation, the bacterial flora changed greatly, and L. plantarum became the most dominant. The next most dominant bacteria were L. vaccinostercus, L. paracollinoides, and K. variicola, which differed by producer. L. vaccinostercus is isolated from cow dung and conducts heterofermentation [Citation20]. L. paracollinoides is isolated from breweries and also conducts heterofermentation [Citation21]. K. variicola is a gram-negative bacterium that is isolated from banana trees [Citation22]. For the colonies isolated on MRS agar plates, the bacterial species was determined by the homology of 16 S rRNA. In addition, L. plantarum, L. pentosus, and L. paraplantarum, which are L. plantarum groups, cannot be distinguished by the homology of 16 S rRNA, so multiplex PCR was performed on the recA gene. As a result, various bacterial species were isolated from the raw tea leaves and tea leaves boiled for a few hours. However, L. pentosus was isolated most frequently from tea leaves after anaerobic fermentation, and L. plantarum was the second most isolated. Thus, it was suggested that L. pentosus plays the most important role in the anaerobic fermentation process of Awa-bancha. To investigate the cause of the selection of specific bacterial species in the anaerobic fermentation process, a growth test of lactic acid bacteria was conducted using a green tea extract containing 1% glucose. As a result, L. plantarum and L. pentosus grew well, while the other bacterial species did not. Tea leaves contain substances that exhibit antibacterial activity, such as tannins and catechins, and bacterial species are subject to growth inhibition. However, it has been reported that the growth of bacteria with diaminopimelic-type peptidoglycan on their cell walls, such as L. plantarum, is not inhibited [Citation23]. In addition, L. pentosus and L. plantarum have been reported to have tannase activity to hydrolyze tannins [Citation24], which might be why they can grow even in tea leaves rich in tannin. In the green tea extract containing 1% glucose, L. plantarum grew better than L. pentosus. However, L. pentosus was frequently isolated from the tea leaves after anaerobic fermentation. L. pentosus was isolated from the tea leaves more frequently than L. plantarum. L. pentosus may be dominant even after anaerobic fermentation. On the other hand, L. plantarum has been reported to be dominant in other post-fermented teas with anaerobic fermentation processes, such as Ishizuchi-kurocha and Miang [Citation3,Citation10]. Therefore, it was suggested that the flora of lactic acid bacteria that inhabit the region varies. L. pentosus and L. plantarum have been reported to produce bacteriocin and may be involved in the formation of microflora in the anaerobic fermentation process [Citation25,Citation26]. In the anaerobic fermentation process of Awa-bancha, the anaerobic conditions and the components of tea leaves became selective pressures, and a microflora mainly composed of L. pentosus was formed. In the tea leaf component analysis, organic acids, catechins, free amino acids, and organic acids were analyzed. In the organic acid analysis, it was found that lactic acid, acetic acid, and succinic acid in the tea leaves increased after anaerobic fermentation. Lactic acid was produced by lactic acid bacteria such as L. pentosus and L. plantarum. In addition, it was considered that acetic acid was produced by lactic acid bacteria with heterogeneous fermentation, and succinic acid was produced by lactic acid bacteria or yeast [Citation27,Citation28]. In the tea leaves after anaerobic fermentation, most producers detected more lactic acid, but producer B detected more acetic acid than lactic acid. The 16 S rRNA-based metagenomics analysis showed that in the tea leaves of producer B after anaerobic fermentation, the genus Lactobacillus only accounted for approximately 42% of the bacteria, which was lower than that of the other producers. It was suggested that when the proportion of the genus Lactobacillus in the bacterial flora in the tea leaves after anaerobic fermentation was low, acetic acid increased more than lactic acid. The free amino acid analysis showed that the amount of free amino acids in the tea leaves tended to decrease owing to anaerobic fermentation. These were presumed to have been used by microorganisms involved in anaerobic fermentation. In contrast, GABA tended to increase. L. brevis, L. plantarum, and L. pentosus have been reported to produce GABA [Citation29–Citation31], and Awa-bancha is likely to be involved in the production of GABA. In the analysis results of catechins, the difference between producers was large. The growth and storage conditions of the raw tea leaves might have had an effect. As for the overall trend of catechins, EGCg decreased and EGC increased. These results were considered to be caused by the decomposition of EGCg into EGC and gallic acid by tannase, such as from L. pentosus and L. plantarum. EGC has a less repellent taste than EGCg [Citation32] and has good absorption efficiency in the intestinal tract [Citation33]. The degradation of catechins by lactic acid bacteria may affect the taste and physiological activity of Awa-bancha. In addition, it was suggested that anti-allergic properties were also improved by changes in the tea leaf components due to anaerobic fermentation. Awa-bancha has more catechins and fewer amino acids than post-fermented tea, such as Shikoku, Ishizuchi-kurocha, and Goishi-cha [Citation4–Citation6,Citation11]. Because Ishizuchi-kurocha and Goishi-cha have an aerobic fermentation process with fungi, catechins are considered to be degraded by esterase enzymes from fungi and amino acids are increased by peptidases. Therefore, Awa-bancha has different components from other post-fermented teas that create a unique flavor. In this study, it was suggested that the flavor of Awa-bancha is formed by the metabolization of the components by the microbial flora centering on L. pentosus in the anaerobic fermentation process. It is thought that the management of the microflora during anaerobic fermentation is important for the production of Awa-bancha with stable quality.
Author contributions
H.N. and M.H. conceived and designed the experiments. H.N. and T.M performed the experiments. H.I. supervised the experiments. H.N. wrote the manuscript. M.H. and H.I. discussed the results and improved the manuscript. All authors read and approved the final manuscript.
Acknowledgments
We thank the Awa-bancha producers for providing the Awa-bancha tea leaves.
Disclosure statement
No potential conflict of interest was reported by the authors.
Correction Statement
This article has been republished with minor changes. These changes do not impact the academic content of the article.
References
- Omori M. Classify various teas. Tea science. Tokyo(JP): Kodansha;2017 25–28. Japanese.
- Jianqing T, Zixiang Z, Bing W, et al. Bacterial and fungal communities in Pu’er tea samples of different ages. J Food Sci. 2018;78(8):1249–1256.
- Chaikaew S, Baipong S, Sone T, et al. Diversity of lactic acid bacteria from Miang, a traditional fermented tea leaf in northern Thailand and their tannin-tolerant ability in tea extract. J Microbiol. 2017;55(9):720–729.
- Horie M, Tada A, Kanamoto N, et al. Evaluation of lactic acid and component change during fermentation of Ishizuchi-kurocha. J Food Process Preserv. 2019;43(11):e14186.
- Kato M, Tamura A, Saitou H, et al. Changes of flavor during production process of Japanese fermented tea (Ishizuchi-kurocha) and its characteristic. J Home Econ Jpn. 1995;46(6):525–530. Japanese.
- Kato M, Tamura A, Omori M, et al. Changes of flavor during production process of japanese fermented tea (Goishi-cha) and its characteristic. J Home Econ Jpn. 1994;45(6):527–532. Japanese.
- Horie M, Nishioka H, Tada A, et al. Microorganisms involved in fermentation of Batabata-cha. J Jpn Soc Taste Technol. 2019;18(2):62–70. Japanese.
- Yamauchi K. Origin of Awa-bancha. Awa tea. Tokushima: Aioi town office;1980 142–154. Japanese.
- Okada S, Takahashi N, Ohara N, et al. Microorganisms involving in fermentation of Awa-bancha, japanese fermented tea leaves. J Jpn Soc Food Sci Technol. 1996;43(1):12–20. Japanese.
- Horie M, Sato H, Tada A, et al. Regional characteristics of Lactobacillus plantarum group strains isolated from two kinds of Japanese post-fermented teas, Ishizuchi-kurocha and Awa-bancha. BMFH. 2019;38(1):11–22.
- Kato M, Tamura A, Mizooti Y, et al. Changes of flavor during production process of japanese fermented tea (Awa-bancha) and its characteristic. J Home Econ Jpn. 1993;44(7):561–565. Japanese.
- Klindworth A, Pruesse E, Schweer T, et al. Evaluation of general 16S ribosomal RNA gene PCR primers for classical and next-generation sequencing-based diversity studies. Nucleic Acids Res. 2013;41(1):e1.
- Lane D. 16S/23S rRNA sequencing. Nucleic acid techniques in bacterial systematics. Hoboken(NJ): Wiley; 1991. p. 115–175.
- Torriani S, Felis GE, Dellaglio F. Differentiation of Lactobacillus plantarum, L. pentosus, and L. paraplantarum by recA gene sequence analysis and multiplex PCR assay with recA gene-derived primers. Appl Environ Microbiol. 2001;67(8):3450–3454.
- Suzuki T. Sample solution preparation method for free amino acid determination. New food analysis method. Tokyo(JP): Korin;1996 499–504. Japanese.
- Goto T. Quantitative determination of functional components (catechins and caffeine) in green tea. Food function research method. Tokyo(JP): Korin;2000 328–333. Japanese.
- Watanabe J, Shinmoto H, Tsushida T. Coumarin and flavone derivatives from estragon and thyme as inhibitors of chemical mediator release from RBL-2H3 Cells. Biosci Biotechnol Biochem. 2005;69(1):1–6.
- Tomitani A, Tanaka A. Origin and evolution of chloroplasts. Protein Nucleic Acid Enzyme. 2000;45(8): 318–328. Japanese.
- Shi-kai D, Guo-Quiang C, Qing C, et al. Rhodoligotrophos jinshengii sp. nov., isolated from activated sludge. Int J Syst Evol Microbiol. 2014;64(9):3325–3330.
- Okada S, Suzuki Y, Kozai M, et al. Lactobacillus species with meso-diaminopimelic acid in peptidoglycan, Lactobacilhts vaccinostercus Kozaki and Okada sp. nov.. J Gen Appl Microbiol. 1979;25(4):215–221.
- Suzuki K, Funahashi W, Koyanagi M, et al. Lactobacillus paracollinoides sp. nov., isolated from brewery environments. Int J Syst Evol Microbiol. 2004;54(1):115–117.
- Rosenbnlueth M, Matínez L, Silva J, et al. Klebsiella variicola, a novel species with clinical and plant-associated isolates. Syst Appl Microbiol. 2004;27(1):27–35.
- Nishiyama R, Kozaki M. Studies on phenol related compounds inhibiting the growth of microorganisms. Bull Teikyo Junior Coll. 1991;8:49–71.
- Ueda S, Nomoto R, Yoshida K, et al. Comparison of three tannases cloned from closely related lactobacillus species: L. plantarum, L. paraplantarum, and L. Pentosus. BMC Microbiol. 2014;14:87–95.
- Guerreiro J, Monteiro V, Ramos C, et al. Lactobacillus pentosus B231 isolated from a portuguese PDO cheese: production and partial characterization of its bacteriocin. Probiotics Antimicrob Proteins. 2014;6(2):95–104.
- Todorov S, Perin L, Carneiro B, et al. Safety of Lactobacillus plantarum ST8Sh and its bacteriocin. Probiotics Antimicrob Proteins. 2017;9(3):334–344.
- Tsuji A, Okada S, Hols P, et al. Metabolic engineering of Lactobacillus plantarum for succinic acid production through activation of the reductive branch of the tricarboxylic acid cycle. Enzyme Microb Technol. 2013;53(2):97–103.
- Conway E, Downey M. An outer metabolic region of the yeast cell. Jcloned from closely related Biochem. 1950;47(3):347–355.
- Huang J, Mei L, Wu H, et al. Biosynthesis of γ-aminobutyric acid (GABA) using immobilized whole cells of Lactobacillus brevis. World J Microbiol Biotechnol. 2007;23:865–871.
- Tajabadi N, Baradaran A, Ebrahimpour A, et al. Overexpression and optimization of glutamate decarboxylase in Lactobacillus plantarum Taj-Apis362 for high gamma-aminobutyric acid production. Microb Biotechnol. 2015;8(4):623–632.
- Zhong Y, Wu S, Chen F, et al. Isolation of high γ‑aminobutyric acid‑producing lactic acid bacteria and fermentation in mulberry leaf powders. Exp Ther Med. 2019;18(1):147–153.
- Narukawa M, Kimata H, Noga C, et al. Taste characterisation of green tea catechins. Int J Food Sci Tech. 2010;45(8):1579–1585.
- Hayashi T, Ueda S, Nomoto R, et al. Anti-obesity effect of joint oral administration of a tannase producing Lactobacillus plantarum and epigallocatechingallate in mice. J Microbiol. 2013;27(3):151–158.