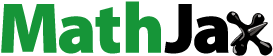
ABSTRACT
In this study, we report the development of a hybrid polymer material comprising poly(butyl methacrylate) and titania, which exhibits the three key elements of visible-light transparency, UV shielding, and self-healing. Nanodispersion of titania in the polymers was achieved using the sol – gel method using titanium propoxide as the raw material. Furthermore, by adding tetrabutylphosphonium chloride, an ionic liquid, we suppressed visible light absorption due to charge transfer between poly(butyl methacrylate) and titania, resulting in enhanced visible light transmittance. An approach involving crosslinking points comprising Diels – Alder functional groups was used to heal scratches in the hybrid material at 75°C for 30 min. Dynamic viscoelastic measurements and microscopic observations were conducted to investigate the self-healing mechanism. These findings suggested that this phenomenon occurred through the fluidization of the polymer matrix and the recombination reaction of the crosslinking points, which comprise Diels – Alder and titania. The development of intelligent materials with combined transparency, UV protection, and self-healing capabilities provides potential avenues for future research to enhance and broaden their versatile characteristics for widespread applications.
1. Introduction
With recent developments in intelligent materials, multifunctional polymeric materials have becomeFootnote1 increasingly important [Citation1]. A typical multifunctional polymer material can be designed by incorporating inorganic components, which impart electrical conductivity [Citation2], optical properties [Citation3], and thermal conductivity [Citation4] to the polymer material. Recently, self-healing has been cited as a critical element in multifunctional materials. Self-healing materials have been intensively studied over the past two decades, with the potential to significantly enhance the safety, longevity, energy efficiency, and environmental impact of engineered materials [Citation5]. In particular, the annual corrosion losses of surface coatings account for almost 2% of the global GDP, and the development of self-healing coatings is urgently required [Citation6]. Hybrid materials comprising inorganic components and optically transparent polymers with properties such as UV protection, heat ray protection, and a high refractive index are in great demand as surface coating materials. It is essential to disperse inorganic components at the nanoscale in an optically transparent polymer matrix to create optically superior hybrid materials [Citation7]. Therefore, developing methods that integrate the self-healing functions and nanodispersion of inorganic components in the polymer matrix is critical to create hybrid materials with optical properties and self-healing functions.
There are two types of self-healing: Extrinsic and intrinsic. Extrinsic self-healing materials trap the monomers in microcapsules or nanocapsules, which are released upon mechanical damage, and then polymerized using a catalyst to reconnect cracks and interfaces [Citation5]. However, this approach provides temporary self-healing properties, posing challenges. Furthermore, when applied to optical materials, adjust the size and refractive index of the capsules in the matrix is crucial, which poses a challenge regarding the versatility [Citation8,Citation9]. Conversely, intrinsic self-healing materials can control molecular cleavage caused by cracks by incorporating networks composed of reversibly reactive functional groups into polymers [Citation10],[Citation11]. Cross-linking structural strategies with reversible Diels – Alder-reactive functional groups have been widely studied and applied to various thermoset materials owing to their temperature-controlled self-healing [Citation12,Citation13]. In contrast, Adachi et al. reported the use of a sol – gel method to prepare styrene hybrid network polymers using tetraethyl orthosilicate and furan-maleimide-modified alkoxysilane complexes as crosslinking points [Citation14]. The advantage of preparing hybrid polymers using the sol – gel process is that it uses alkoxysilane in the side chain to generate inorganic nanoparticles and simultaneously form a crosslinked structure, resulting in the suppression of phase separation between the inorganic nanoparticles and the polymer. Although this hybrid polymer exhibited good silica dispersibility, its optical and self-healing properties have not been investigated thus far.
The sol – gel method is useful for preparing highly dispersible hybrid polymer materials with high inorganic components, but, optical transparency remains a challenge. In our previous study, we prepared a titania/polymethyl methacrylate hybrid material by the sol – gel method and demonstrated that the hydrogen bond interaction between titania and the carbonyl group of polymethyl methacrylate expands the light absorption region of titania [Citation15]. We also showed that tetrabutylphosphonium chloride (TBPC) is an effective ionic additive for inhibiting these hydrogen bonds, thus improving the transparency and mechanical properties of titania/poly(methyl methacrylate) (PMMA) hybrid materials. Moreover, by incorporating TBPC into a titania/PMMA hybrid material with thermoreversible crosslinking points comprising titania and carboxyl groups, we developed a recyclable, transparent, and hybrid network polymer material exhibiting UV shielding ability [Citation16]. However, these materials do not possess self-healing properties because of the high glass transition temperature of PMMA.
In this study, we synthesized a copolymer of butyl methacrylate (BMA) and a monomer comprising a retro Diels – Alder functional group and a silane group (). We selected BMA because of its lower glass transition temperature than that of PMMA. Additionally, the ratio of BMA to the monomer with Diels – Alder functionality (monomer DA) was determined to be 95:5 through various studies on titania hybrid materials, including TBPC (details of monomer and polymer synthesis are provided in the Supporting Information) [Citation15–18]. The polymerization of monomer DA was carried out under tert-butoxycarbonyl (BOC) protection because both monomer DA and the monomer with a silyl group exhibited gel behavior during precipitation in methanol. Poly(BMA-co-triethylsilane-DA) was successfully synthesized via the deprotection of the BOC of poly(BMA-co-Boc-DA) and its reaction with triethyl isocyanate. A hybrid network polymer material with high transparency, UV shielding ability, and self-healing properties is fabricated using the sol – gel method in the presence of this polymer, titanium isopropoxide, and TBPC (). The Diels – Alder reaction plays a crucial role in repairing scratched polymers and occurs through an equilibrium reaction between 75 and 120°C. Moreover, this reaction involved an exchange reaction of crosslinking points via titania.
2. Experimental section
The detailed synthesis method for the raw materials, with Diels – Alder parts, and copolymers are described in the Supporting Information.
2.1. Hybrid film preparation by the sol – gel method
Poly(BMA-co-triethylsilane-DA) (2.5 g) was dissolved in THF (80 mL). Titanium tetraisopropoxide (TiOPr) (15 wt% based on the copolymer) was added under nitrogen atmosphere, followed by the addition of TBPC (10 wt% based on the copolymer). Subsequently, 1 M hydrochloric acid (25 μL) was added dropwise, and the mixture was heated at 20°C for 15 min and stirred for 1 min. This mixture was spread on a Petri dish and allowed to stand at room temperature for 2 d to form a film. Subsequently, it was heat-treated at 100°C for 90 min in an argon atmosphere to obtain a hybrid film denoted as Ti15TB10DA. The hybrid films comprising 15 wt% poly(BMA-co-MSi) and TiOPr without TBPC and 15 wt% TiOPr with 10 wt% TBPC: 10 wt % were denoted as Ti15TB0 and Ti15TB10, respectively.
2.2. Characterization
UV – Vis measurements were performed using a JASCO V-670 instrument at a scan speed of 400 nm/min, a measurement wavelength range of 200–800 nm, and room temperature. In addition, the transmittance is calculated using EquationEquation (3)(3)
(3) below with the thickness correction value of 0.02 cm to correct the sample thickness.
where T1 is the transmittance after thickness correction (%), T2 is the measured transmittance (%); t1 is the thickness correction value (0.02 cm), t2 is the sample thickness (cm), and μ is the linear absorption coefficient. DMA was performed using a DMS6100 model manufactured by Hitachi High-Tech Science Co., Ltd. The measurements were conducted in the tensile mode, with a measurement temperature between −10 and 300°C, a frequency of 1 Hz, and a N2 gas flow rate of 200 mL/min. The specimen used was 8 mm × 30 mm, and the distance between the chucks was set at 10 mm. An OLYMPUS system polarizing microscope BX53-33P-0C-1 was used for the polarizing microscope, and the camera magnification was set to 100 × .
3. Results and discussion
3.1. Investigation on the amount of TBPC added to the PBMA matrix
The amount of titanium isopropoxide added (15 wt% relative to the copolymer) was determined according to previous studies. The TBPC content in the PBMA matrix was limited to 10 wt% because leakage was confirmed from the film surface when 15 wt% TBPC was added. According to our previous report, the side chain carbonyls of TBPC interacted with PMMA, as confirmed by infrared measurements, revealing that PMMA matrixes can hold up to 80 wt% TBPC [Citation16,Citation18]. This difference was attributed to the strong hydrophobic interaction between the alkyl chains of butyl methacrylate, which has a longer side-chain alkyl chain than methyl methacrylate, resulting in variations in the interaction between the carbonyl groups of both polymers and TBPC. Moreover, we concluded that the polarity acting on the carbonyl group weakened with the lengthening of the side chain, resulting in a relatively stronger van der Waals interaction that led to the leakage of TBPC from PBMA [Citation19]. In contrast, it is essential to investigate the effect of TBPC addition on the mechanical properties of the PBMA matrix because variations in the alkyl chains also affect the composition of hydrogen bonds between titania and PBMA [Citation15–18]. To confirm the effect of TBPC on mechanical properties of the PBMA matrix, tensile tests were performed on 15 wt% titania precursor with TBPC contents of 0 and 10 wt% without Diels – Alder functional groups (Ti15TB0 and Ti15TB10) (). Consequently, the tensile strength improved by A times, and the toughness improved by B times (), which is similar to the improvement observed when 40 wt% TBPC is added to the PMMA/titania hybrid system. This result shows that the reduced polarity owing to the long alkyl chain weakens the hydrogen bond with titania, resulting in improved mechanical strength even with the addition of a small amount of TBPC.
3.2. Optical properties of hybrid films
shows Ti15TB0, Ti15TB10 and a hybrid film containing 15 wt% titania precursor and 10 wt% TBPC with Diels – Alder functional groups (Ti15TB10DA). In addition, the hybrid film exhibited good uniformity and no phase separation of the components was observed. shows the UV – Vis spectra of each hybrid film. All hybrid films blocked light at wavelengths below 350 nm owing to absorption by titania. A discernible difference was observed in the visible light transmittance between Ti15TB0 and Ti15TB10. This phenomenon was not observed in the PMMA matrixes. This difference in the electron-withdrawing properties of butyl methacrylate induces charge transfer in titania and redshifts its absorption region. Therefore, Ti15TB0 resulted in a yellow sample with lower visible light transmission than that of the PMMA sample. In contrast, Ti15TB10DA exhibited high transparency, although its visible light transmittance was lower than that of Ti15TB10. Considering the disparity in transparency between Ti15TB0 and Ti15TB15, it is reasonable to infer that the absorption of Ti15TB10DA from 350 to 450 nm originates from the absorption of the Diels – Alder functional group. These results indicate that TBPC improves the visible light transmittance of the hybrid film by inhibiting the interaction between titania and the carbonyl group of butyl methacrylate, which is consistent with the mechanism of improving mechanical properties.
3.3. Dynamic-mechanical properties of hybrid films
The DMA method is ideal for analyzing polymeric materials with thermoreversible networks. Moreover, utilizing the DMA method, the elastic modulus can be obtained for these materials as a function of temperature and time [Citation20,Citation21]. shows the DMA characteristics of each hybrid film. A plateau region was observed in the storage modulus (E’) of Ti15TB0 and Ti15TB10 between 100 and 250°C. These results indicate that a covalent network was formed in both the films. Additionally, the peak temperature of tan δ, which indicates the glass transition temperature (Tg) of the hybrid film, was significantly different for Ti15TB0 (69 °C) and Ti15TB10 (65 °C). This result indicates that TBPC did not affect the glass transition temperature of the PBMA matrix. Conversely, the glass transition temperature of Ti15TB10DA was 51°C, which was significantly lower than the other two films. This result could be attributed to the inhibition of the interaction between the polymer chains owing to the bulkiness of the side chains of the Diels – Alder part. In addition, the slope of E’ of Ti15TB10DA, which was in the range of 50–120°C, decreased gradually. The DA adduct, comprising maleimide and furan, is a reversible reaction that forms a strong bond at room temperature. However, at higher temperatures, the equilibrium shifts toward dissociation [Citation22], and these reactions are equilibrium reactions. A gentle E’ slope was also observed in the previous hybrid material resulting from an equilibrium reaction between the carboxy groups and titania [Citation16]. Moreover, the slope of E’ decreases sharply above 120°C. Therefore, the results obtained from DMA indicate that the network consists of equilibrium reactions between 70 and 120°C, and that the network of Ti15TB10DA disconnects at 120°C. These data indicate that the titania-mediated Diels – Alder crosslinking point functioned as designed. However, compared to Ti15TB0 (1.4 MPa), E’ at 30°C for Ti15TB10 (0.9 MPa) and Ti15TB10DA (0.4 MPa) were lower. This decrease could be attributed to the plasticizing effect of TBPC addition.
3.4. Self-healing performance of hybrid film
Scratch tests were conducted to evaluate the self-healing properties of the hybrid films. The appropriate conditions for self-healing were determined to be above the glass transition temperature and below 120°C, which corresponded to the dissociation temperature of the Diels – Alder complex [Citation23]. Therefore, a healing test was conducted at 75°C based on the DMA results. shows the scratch test results for Ti15TB10DA, which was recovered from an A-shaped wound within 30 min. To investigate the healing status of the scratches, changes in the scratches over time are confirmed (). The order of scratch healing occurs in the following order: Linear scratches first exchange many points and then the points heal. In contrast, the scratch test of Ti15TB10 is incubated for 24 h at 130°C, which is equivalent to an E of 75°C for Ti15TB10DA (). However, the scratches on Ti15TB10 did not recover. These results suggest that the healing process not only filled the scratch by the fluidization of the PBMA matrix, but also by the cooperative effect of the recombination reaction of the Diels-Alder on the crosslinking point. However, it the range of wounds that can be recovered from this self-healing method is narrow. No recovery of the fully cut film was observed, which could be attributed to the high viscosity of acrylic and the short polymer flow distance of the PBMA matrix. Consequently, mutual penetration was incomplete and recovery was impossible. However, the transparency, UV-shielding ability, and rapid scratch recovery performance demonstrated the utility of this system as a coding material.
Figure 4. DMA curve of each hybrid film. Ti15TB0 (red line), Ti15TB10 (blue line), and Ti15TB10DA (black line).
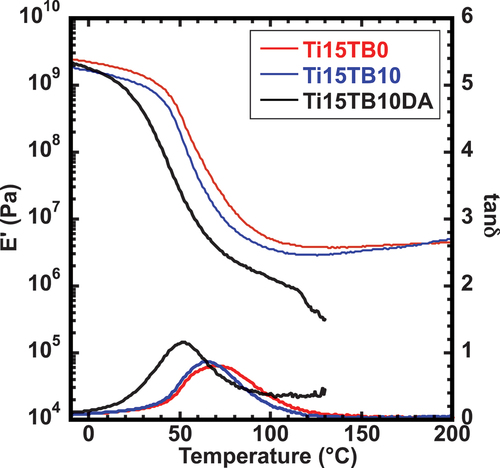
Figure 5. (a) Image of self-healing of Ti10TB10DA incubated at 75°C for 30 min: black bar is 200 μm. The black arrow is an A-shaped wound. (b) Time dependence of self-healing of Ti10TB10DA incubated at 75°C: black bar is 50 μm. (c) Image of Ti10TB10 incubated at 120°C for 30 min: black bar is 200 μm.
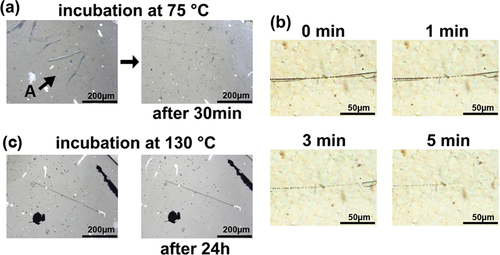
4. Conclusion
In this study, a polymer was synthesized by copolymerizing BMA with a monomer containing methacrylic, Diels – Alder, and silyl groups, and a titania hybrid film was fabricated using the sol – gel method. The hybrid film prepared by the sol – gel method can be introduced without the phase separation of titania. TBPC suppressed the charge transfer of titania by inhibiting the interaction between titania and butyl methacrylate, resulting in improved mechanical properties. Therefore, the hybrid film exhibited UV shielding and high visible light transparency. Furthermore, by designing a cross-linking point between the titania and Diels – Alder parts, we demonstrated that scratches could be repaired within 3 min by heating at 75°C. Therefore, this system contributes to the design of intelligent materials that integrate different functions including transparency, UV protection, and self-healing.
Author contributions
Conceptualization, S.H.; methodology, M.I.; investigation, A.F.writing – original draft preparation, S.H.; writing – review and editing, S.H.; supervision, S.S. and T.T.; project administration, H.I.
Supporting information (1).docx
Download MS Word (998.9 KB)Disclosure statement
No potential conflict of interest was reported by the author(s).
Supplementary material
Supplemental data for this article can be accessed online at https://doi.org/10.1080/09276440.2024.2346672
Notes
1. Tetrabutylphosphonium chloride (TBPC); Poly(methyl methacrylate) (PMMA); Butyl methacrylate (BMA); Tert-butoxycarbonyl (BOC); DMA.
References
- Chung DDL. A review of multifunctional polymer-matrix structural composites, compos. B Eng. 2019;160:644–660. doi: 10.1016/j.compositesb.2018.12.117
- Gaikwad N, Gadekar P, Kandasubramanian B, et al. Advanced polymer-based materials and mesoscale models to enhance the performance of multifunctional supercapacitors. J Energy Storage. 2023;58:106337. doi: 10.1016/j.est.2022.106337
- Wu B, Xu X, Tang Y, et al., Multifunctional optical polymeric films with photochromic, fluorescent, and ultra-long room temperature phosphorescent properties, Adv Opt Mater. 2021;9: 2101266. 10.1002/adom.202101266.
- Pan X, Debije MG, Schenning APHJ. High thermal conductivity in anisotropic aligned polymeric materials. ACS Appl Polym Mater. 2021;3:578–587. doi: 10.1021/acsapm.0c01340
- White SR, Sottos NR, Geubelle PH, et al. Autonomic healing of polymer composites. Nature. 2001;409(6822):794–797. doi: 10.1038/35057232
- Nakahata M, Mori S, Takashima Y, et al. Self-healing materials formed by cross-linked polyrotaxanes with reversible bonds. Chem. 2006;1(5):766–775. https://www.cell.com/chem/pdf/S2451-9294(16)30158-9.pdf
- Althues H, Henle J, Kaskel S. Functional inorganic nanofillers for transparent polymers. Chem Soc Rev. 2007;36:1454–1465. doi: 10.1039/B608177K
- Kyprianidou-Leodidou T, Caseri W, Suter UW. Size variation of PbS particles in high-refractive-index nanocomposites. J Phys Chem. 1994;98:8992–8997. doi: 10.1021/j100087a029
- Walter C. Nanocomposites of polymers and metals or semiconductors: historical background and optical properties, Macromol. Macromol Rapid Commun. 2001;21(11):705–722. doi: 10.1002/1521-3927(20000701)21:11<705:AID-MARC705>3.0.CO;2-3
- Yang Y, Urban MW. Self-healing polymeric materials. Chem Soc Rev. 2013;42:7446–7467. doi: 10.1039/C3CS60109A
- Wu DY, Meure S, Soloman D. Self-healing polymeric materials: a review of recent developments, prog. Polym Sci. 2008;33(5):479–522. doi: 10.1016/j.progpolymsci.2008.02.001
- Feula A, Pethybridge A, Giannakopoulos I, et al. A thermoreversible supramolecular polyurethane with excellent healing ability at 45 °C. Macromolecules. 2015;48(17):6132–6141. doi: 10.1021/acs.macromol.5b01162
- Ghosh B, Urban MW. Self-repairing oxetane-substituted chitosan polyurethane networks. Science. 2009;323(5920):1458–1460. doi: 10.1126/science.1167391
- Adachi K, Achimuthu AK, Chujo Y. Synthesis of Organic−Inorganic polymer hybrids controlled by Diels−Alder reaction. Macromolecules. 2004;37(26):9793–9797. doi: 10.1021/ma0400618
- Hara S, Ishizu M, Watanabe S, et al. Improvement of the transparency, mechanical, and shape memory properties of polymethylmethacrylate/titania hybrid films using tetrabutylphosphonium chloride. Polym Chem. 2019;10(35):4779–4788. doi: 10.1039/C9PY00783K
- Hara S, Tomono M, Fukumoto K, et al. Melt-moldable copolymethacrylate/titania thermoreversible polymer networks with shape memory. ACS Appl Polym Mater. 2020;2(12):5654–5663. doi: 10.1021/acsapm.0c00967
- Ikake H, Hara S, Kurebayashi S, et al. Development of a magnetic hybrid material capable of photoinduced phase separation of iron chloride by shape memory and photolithography. J Mater Chem C. 2022;10(20):7849–7856. doi: 10.1039/D1TC06055D
- Ikake H, Hara S, Kubodera M, et al. Macroscopic property evaluation of titania nanocomposite polymer capable of drawing double-network macrostructure using photolithography. ACS Macro Lett. 2023;12(7):943–948. doi: 10.1021/acsmacrolett.3c00230
- Wulf M, Grundke K, Kwok DY, et al. Influence of different alkyl side chains on solid surface tension of polymethacrylates. J Appl Polym Sci. 2000;77(11):2493–2504. doi: 10.1002/1097-4628(20000912)77:11<2493:AID-APP19>3.0.CO;2-H
- Safaei A, Terryn S, Vanderborght B, et al. Toughening and stiffening in thermoreversible Diels–Alder polymer network blends. Macromolecules. 2023;56(11):4325–4335. doi: 10.1021/acs.macromol.2c02558
- Wang A, Niu H, He Z, et al. Thermoreversible cross-linking of ethylene/propylene copolymer rubbers. Polym Chem. 2017;8(31):4494–4502. doi: 10.1039/C7PY00896A
- Chen X, Dam MA, Ono K, et al. A thermally re-mendable cross-linked polymeric material. Science. 2002;295(5560):1698–1702. doi: 10.1126/science.1065879
- Davidson JR, Appuhamillage GA, Thompson CM, et al. Design paradigm utilizing reversible Diels–Alder reactions to enhance the mechanical properties of 3D printed materials. ACS Appl Mater Interfaces. 2016;8(26):16961–16966. doi: 10.1021/acsami.6b05118