ABSTRACT
Demethylnobiletins are nobiletin metabolites, with 4′-demethylnobiletin being the most abundant in the demethylated compounds upon feeding with nobiletin. Previous studies have repeatedly shown that demethylnobiletin has higher physiological activity than nobiletin; however, no studies have yet suggested that 4′-demethylnobiletin could affect biological rhythms. Therefore, in the present study, we analyzed the effects of fermented Citrus reticulata, containing a large amount of 4′-demethylnobiletin, on the locomotor activity level, locomotor activity rhythms under normal light-dark and constant-dark conditions, and peripheral clock rhythms. We demonstrated that fermented Citrus reticulata not only modified the changes in the locomotor activity rhythms caused by high-fat diet feeding in mice but also inhibited the phase shift of the peripheral clock rhythms caused by high-fat diet feeding. Taken together, the results of this study suggest that fermented Citrus reticulata could potentially be a new functional food that could help to maintain normal biological rhythms.
Introduction
The circadian clock system is an inherent mechanism in almost all species. In mammals, this system is composed of several clock genes, such as period 1/2 (Per1/2), cryptochrome 1/2 (Cry1/2), brain and muscle arnt-like protein 1 (Bmal1), and circadian locomotor output cycles kaput (Clock) (Buhr and Takahashi Citation2013). These clock genes generate a rhythmic cycle of approximately 24 h by creating a transcriptional and translational negative feedback loop (Reppert and Weaver Citation2002). This system has central and peripheral clocks (Hastings Citation1997; Weaver Citation1998). The central clock, which dominates in the suprachiasmatic nucleus (SCN) in the hypothalamus, is entrained by the environmental light-dark cycle (Nelson and Zucker Citation1981). Subsequently, the central clock synchronizes the peripheral clocks, located in almost all peripheral organs, through neural signals, hormonal signals, and other pathways (Albrecht Citation2012; Bass and Takahashi Citation2010). Peripheral clocks can be organized not only by the central clock, but also by environmental stimuli, such as feeding, temperature, and stress (Hara et al. Citation2001; Ohnishi et al. Citation2014; Tahara et al. Citation2015). The central and peripheral clocks play an important role in modulating physiological functions, metabolism, body temperature, and sleep-wake behavior (Gachon et al. Citation2004). Accordingly, we need to control not only the timing of light exposure but also the timing of environmental stimulation in order to maintain healthy physiological functions and a well-balanced circadian clock.
Circadian clocks, especially the peripheral clocks, are affected not only by meal timing but also by some nutrients. Nobiletin (Nob) is a nutrient that affects clock gene expression rhythms. Many previous studies have indicated that Nob prolongs the period length and shifts the phases of PER2 expression rhythm in mouse embryonic fibroblasts (MEFs) (Shinozaki et al. Citation2017), and that Nob prevents a decrease in locomotor activity levels caused by high-fat diet (HFD) feeding in mice (He et al. Citation2016). Previous studies have suggested that these effects are mediated through the inhibition of adenosine receptors, the increase in intracellular cyclic adenosine monophosphate (cAMP) by non-selective phosphodiesterase inhibition, and other signaling pathways, such as the retinoic acid receptor-related orphan receptor (ROR) nuclear receptor-related and extracellular signal-regulated kinase (ERK)/cAMP response element-binding protein (CREB) pathways (Kawahata et al. Citation2013; He et al. Citation2016). Taken together, multiple pieces of evidence suggest that some nutrients affect circadian clocks by themselves.
Only a few studies have shown that Nob can negatively impact health. Almost all Nob is transformed into demethylnobiletin, especially 4′-demethylnobiletin (4DMNob) (Wu et al. Citation2015a, Yasuda et al. Citation2003). Indeed, in rat colonic mucosa, 4DMNob is the most abundant demethylated metabolite upon Nob feeding (Wu et al. Citation2015b). Moreover, many studies have demonstrated that in comparison with Nob, demethylnobiletin has a more relevant contribution to various processes, such as anti-inflammatory and anti-cancer mechanisms, and carbohydrate or lipid metabolism (Lo et al. Citation2010; Wu et al. Citation2018, Wu et al. Citation2015a). Taken together, these studies suggest that fermented Citrus reticulata (FCR), a functional food containing increased amounts of 4DMNob, would have stronger effects on certain physiological functions than Nob.
To the best of our knowledge, no studies to date have demonstrated the impact of 4DMNob and FCR on circadian clocks. Shinozaki et al. suggested that not only does Nob affect PER2 expression rhythm but also that the effects of flavonoids with similar chemical structures do not always similarly affect the circadian clocks (Kawahata et al. Citation2013; He et al. Citation2016; Shinozaki et al. Citation2017). Therefore, 4DMNob and FCR might not always affect the circadian clock in the same manner as Nob. In the current study, we investigated the effect of 4DMNob and FCR on circadian clocks using both in vitro and in vivo experimental approaches.
Materials and methods
Nob, 4DMNob, and FCR
For the in vitro experiments, 4DMNob was dissolved in 0.1% dimethyl sulfoxide (DMSO; Wako Pure Chemical Industries, Ltd., Japan). For the in vivo experiments, FCR, containing a large amount of 4DMNob and little Nob, was blended with HFD (D12451M; RESEARCH DIETS Inc., Japan) (Kawahata et al. Citation2017). FCR preparation has been described previously (Kawahata et al. Citation2017). 4DMNob and FCR were provided by Fuji Sangyo Co., Ltd. (Japan).
Cell culture
MEFs were derived from PERIOD2::LUCIFERASE (PER2::LUC) knock-in mice (Yoo et al. Citation2004). MEFs were cultured in Dulbecco’s modified Eagle’s medium (D-MEM, Wako Pure Chemical Industries, Ltd.) supplemented with 10% FBS (Bio West, USA) with heat-inactivation and 1% penicillin/streptomycin (Wako Pure Chemical Industries, Ltd.), and were passaged twice a week. MEFs were incubated at 37°C under a 5% CO2 atmosphere.
PER2::LUC MEF bioluminescence measurement
The rhythmic expression of PER2::LUC in MEFs was measured using a real-time LUCIFERASE assay. MEFs were passaged at a concentration of 6 × 104 cells onto 35-mm dishes (AGC Techno Glass Co. Ltd., Japan). The following day, MEFs were stimulated with 100 nM dexamethasone (Sigma-Aldrich Corp, USA) for 2 h to synchronize the clock gene expression rhythm between cells, before being replaced with D-MEM as mentioned above, containing 0.1 mM D-luciferin sodium salt (Invitrogen, USA). MEFs were incubated at 37°C, and bioluminescence was monitored for 1 min at each 10-min interval with a dish-type luminometer (LumiCycle 32, Actimetrics, USA). For chronic treatment, MEFs were treated with the vehicle (0.1% DMSO) or each nutrient after dexamethasone stimulation, and bioluminescence was monitored for 6 days.
Circadian rhythm evaluation in MEFs
Original data were smoothed by an adjusting-averaging method using 2-h running means, as previously described (Hayasaka et al. Citation2007; Ohta et al. Citation2008). Then, the data set was de-trended by subtracting the 24-h running average from the raw data using R software (http://www.r-project.org/). Bioluminescence was measured for six consecutive days. The first, second, and third peaks were defined as peaks 1, 2, and 3, respectively (). The amplitude was calculated based on peak 1 bioluminescence. During the MEF bioluminescence measurements, the period length was calculated as half the interval between peaks 1 and 3 of the waveform.
Figure 1. PER2::LUC expression rhythm effects on MEFs. (A) Representative de-trended data for cells treated with 0.1% DMSO as a vehicle or with 4DMNob (1–30 μM). (B) Effect of each treatment on the period length of the rhythmic PER2::LUC expression. (C) Effect of each treatment on PER2::LUC bioluminescence amplitude of peak 1. Data are presented as means ± SEM (n = 4). * p < 0.05, versus vehicle (one-way ANOVA with Tukey’s multiple comparison test). # p < 0.05, ## p < 0.01 (Kruskal–Wallis test with Dunn’s multiple comparison test).
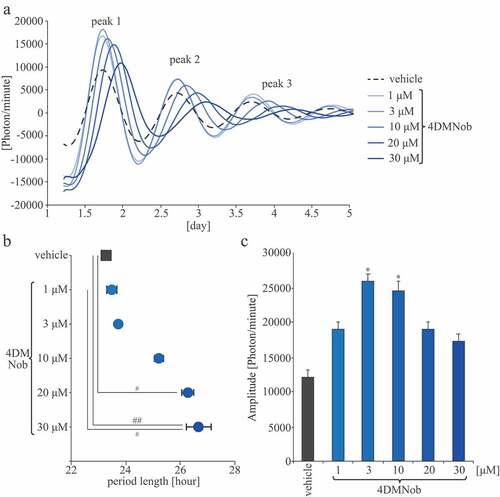
Animals
Eight-week-old male ICR mice and heterozygous PER2::LUC mice (background: ICR mice) were housed in an animal room maintained at a temperature of 22 ± 2°C, a humidity level of 60 ± 5%, and under a 12-h light/dark cycle (LD condition; lights on from 08:00 to 20:00). Zeitgeber time 0 (ZT0) and 12 were defined as the lights-on and light-off times, respectively. PER2::LUC mice were bred in our laboratory and housed in an animal room as previously described (Tahara et al. Citation2012). They were provided with an EF mouse chow (Oriental Yeast Co. Ltd., Japan) – mouse food whose exact composition is unknown – and water was provided ad libitum before the experiments. During the experiments, mice were provided with HFD or AIN-93 M (Oriental yeast Co., Ltd., Japan) – mouse food with known exact composition – and water was provided ad libitum before the experiments. Experimental animal care was conducted with the permission of the Animal Welfare Committee of Waseda University (2018-A026 and 2019-A056).
Locomotor activity rhythm analysis
Locomotor activity was monitored using a SE-10 infrared radiation sensor (Akizuki Denshi Tsusho Co. Ltd., Japan) and analyzed using CLOCKLAB software (Actimetrics), as previously described (Haraguchi et al. Citation2018). Using the CLOCKLAB software, the locomotor activity level was analyzed in each minute while mice were kept under LD conditions, and the free-running period length, which is regarded as an endogenous rhythm, was calculated while mice were kept under a constant dark (DD) condition. In the locomotor activity rhythm under LD conditions, original data (1-min bins) were smoothed by an adjusting-averaging method with 2-h running means. The free-running period length under DD conditions was calculated using the chi-squared periodogram (Sokolove and Bushell Citation1978).
In vivo monitoring system protocol and data analysis
The experimental procedure and data analysis of the in vivo monitoring system were performed as described previously (Haraguchi et al. Citation2018). In short, PER2::LUC mice were anesthetized with isoflurane (Mylan Inc., Japan) using an in vivo imaging system (IVIS, PerkinElmer, Inc., USA). Mice were anesthetized before being injected subcutaneously on the back and near the neck with D-luciferin potassium salt (Promega, USA) at a concentration of 15 mg/kg body weight. Images were taken at 8 and 10 min post-injection in the dorsal-up position for the kidney and the ventral-up position for the liver and the submandibular gland (sub gla), respectively. The imaging exposure time was 1 min. Images from the same mice were taken at 6 consecutive time points per day at 4-h intervals. Mice were recovered quickly from isoflurane anesthesia, following the imaging session, and returned to their home cages. Bioluminescence emitted by each organ was calculated automatically using Living Image 3.2 software (PerkinElmer, Inc.). For each organ in each mouse, the average photon/min level from 6 consecutive time points was calculated as 100%, and the bioluminescent rhythm was expressed as a percentage. The acrophase of the bioluminescent rhythm was analyzed using the single cosinor procedure program (Acro.exe, 3.5) (Refinetti et al. Citation2007). These interventions did not affect peripheral clocks in a previous study (Tahara et al. Citation2012).
Data analysis
All values are expressed as the mean ± standard error of the mean (SEM). Statistical analyses were performed using the GraphPad Prism version 6.03 software (USA). We verified whether the data showed a normal or non-normal distribution and equal or biased variation, by assessing with the D’Agostino-Pearson normality test/one-sample t-test and F-value test/Bartlett’s test, respectively. The parametric analysis was performed using one-way analysis of variance (ANOVA) with Tukey’s multiple comparison test, and non-parametric analysis was conducted using the Mann Whitney test, t-test with false discovery rate (FDR) multiple testing correction, or Kruskal-Wallis test with Dunn’s multiple comparison test.
Results
4DMNob strongly affects the PER2::LUC expression rhythm in MEFs
To analyze the effects of 4DMNob on the PER2::LUC expression rhythm, MEFs were treated with the vehicle (0.1% DMSO) or 4DMNob (1–30 μM) (). Compared with the vehicle group, the 4DMNob (10–30 μM) group extended the PER2::LUC expression rhythm circadian period. There were significant differences between the vehicle group and the 4DMNob (20 or 30 μM) groups (). Moreover, these results suggest that 4DMNob has a stronger prolongation effect on the PER2::LUC expression rhythm period length compared with the prolongation effect of Nob in a previous study. The respective previous study showed similar PER2::LUC expression rhythm circadian period of the vehicle and the 30 μM Nob groups, and found that 100 μM of Nob significantly extended the PER2::LUC expression rhythm circadian period compared with that observed in the vehicle group (Shinozaki et al. Citation2017). The PER2::LUC expression rhythm amplitudes in the 4DMNob group were higher than those in the vehicle group. There were significant differences between the vehicle group and the 4DMNob (3 or 10 μM) groups (). These results indicate that 4DMNob has increasing and strong prolongation effects on the PER2::LUC expression rhythm and that this effect of 4DMNob is stronger than that of Nob. Therefore, in the following in vivo experiments, we addressed the effects of FCR, containing a large amount of 4DMNob, on biological rhythms, such as the locomotor activity rhythm and peripheral clock rhythms.
FCR attenuates locomotor activity rhythm disturbance caused by HFD feeding
To confirm whether FCR affects locomotor activity rhythms, mice were fed with HFD containing 0.257% FCR, which corresponded to 0.06% 4DMNob, and the locomotor activity levels were monitored under LD conditions. In this experiment, mice were acclimated to HFD feeding for the first week and then divided into HFD and HFD+FCR groups, in which they were fed HFD or 0.257% FCR-supplemented HFD, respectively. Mice were kept under each food condition for 1 week under LD conditions and then released under DD conditions for the next 4 weeks (). First, we analyzed locomotor activity levels under LD conditions. The total activity levels in the HFD+FCR group tended to be higher than those in the HFD group throughout the day (), especially at the beginning of the active period (), and the percentage of the activity levels during the active period in the HFD+FCR group tended to be lower than that in the HFD group (). In addition, we examined the effects of FCR on mice fed a normal diet (ND; AIN-93 M) under the same experimental schedule (Supplemental Figure S1). The ND+FCR group showed similar locomotor activity rhythms, total activity levels, and percentage of activity levels during the active period compared to those observed in the ND group (Supplemental Figure S1A-1D). The HFD+FCR group showed higher activity levels at the beginning of the active period than those observed in the HFD group (Supplemental Figure S1B), consistent with the results shown in . The total activity levels in the HFD+FCR group were similar to those in the HFD group, and the percentages of activity levels during the active period in the HFD+FCR group were higher than those in the HFD group (Supplemental Figure S1C and 1D). However, there were no significant differences between the HFD and HFD+FCR groups (Supplemental Figure S1C and 1D). Moreover, in the additional experimet, the tolal locomotor activity levels in the ND group were higher than those in the HFD group and the percentage of the activity levels during the active period in the ND group were higher than those in the HFD group (Supplemental Figure S1C and 1D). Taken together, our first experiment showed the preventive effects of FCR on the decrease in the total locomotor activity levels caused by HFD feeding (); an additional experiment showed the preventive effects of FCR on the decrease in the percentage of the activity levels during the active period caused by HFD feeding (Supplemental Figure S1). In the first experiment, the percentage of activity levels during the active period in the HFD group was approximately 90%, and such high values may be unaffected by HFD feeding (). These results indicated that FCR modified the locomotor activity under HFD feeding.
Figure 2. The effects of FCR on the locomotor activity levels and the free-running period length of the locomotor activity rhythm. (A) Representative double-plotted actograms for mice fed with HFD (the HFD group) or with HFD supplemented with 0.257% FCR (the HFD+FCR group). The horizontal lines indicate the local time and the vertical lines indicate the passing day. The gray shadow on the actograms indicates the dark period. (B) The locomotor activity rhythm under LD condition. We averaged the locomotor activity rhythm for 7 days. Open and closed bars indicate light and dark periods, respectively. (C) The average of total locomotor activity levels for 7 days in both groups under LD condition. (D) The average percentage of activity levels during the active period under LD condition. (E) The free-running period of locomotor activity rhythm was analyzed for all days during DD condition. Data are presented as means ± SEM (n = 5). * p < 0.05 vs the HFD group (t-test with FDR multiple testing correction). $ p < 0.05 (Mann Whitney test).
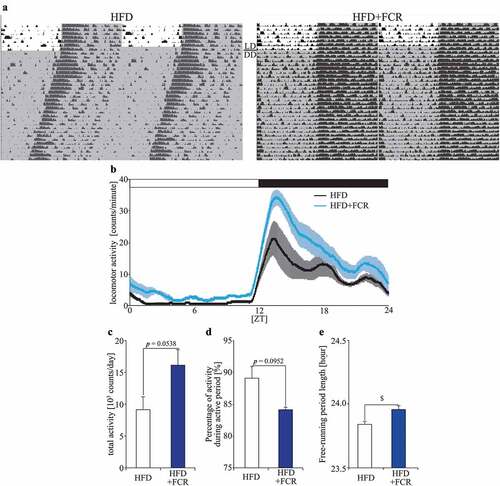
In the next experiment, we analyzed the free-running period length of the locomotor activity rhythm under DD conditions (). The free-running period length in the HFD+FCR group was significantly longer than that in the HFD group (). We also examined the effects of FCR on mice fed with ND under the same experimental schedule (Supplemental Figure S1). The ND+FCR group showed a free-running period length similar to that of the ND group (Supplemental Figure S1E). In the additional experiment, the HFD+FCR group showed a longer free-running period length than the HFD group (Supplemental Figure S1E), consistent with the results shown in , but there wes no significant difference by two-way ANOVA with Tukey’s multiple comparison test. On the other hand, the free-running period length in the HFD+FCR group was similar to that of the ND and ND+FCR groups. These results indicate that FCR has a preventive effect on the changing of the free-running period length caused by HFD feeding.
We monitored food intake and body weight in an additional experiment (Supplemental Figure S2). The ND+FCR group consumed more food than the ND group, and the HFD+FCR group consumed the amount of food similar to the HFD group (Supplemental Figure S2A). The ND+FCR group showed body weight similar to that of the ND group, and the HFD+FCR group showed body weight similar to that of the HFD group (Supplemental Figure S2B). Taken together, these results demonstrate that FCR would prevent the disturbance of locomotor activity rhythms caused by HFD feeding, regardless of the effects of FCR on food intake and body weight.
FCR attenuates peripheral clock impairment caused by HFD feeding
To analyze whether FCR affects the peripheral clocks, PER2::LUC mice were fed with 0.257% FCR-supplemented HFD under LD conditions, and the PER2::LUC expression rhythms in peripheral organs were monitored using IVIS (). We included an ND group in this experiment, in which mice of the HFD and HFD+FCR groups were fed with AIN-93 M. After 2 weeks under each food condition, the peripheral clocks were monitored using IVIS. HFD feeding reduced the PER2::LUC expression rhythm amplitude compared with ND feeding, while FCR inhibited the HFD feeding-mediated amplitude decrease only in the kidneys. However, no significant differences were observed (). Regarding the acrophase, the HFD group delayed the PER2::LUC expression rhythm phases in the peripheral organs, and there were significant differences between the ND and HFD groups in the kidney and sub gla. Interestingly, both the HFD+FCR and ND groups showed a similar acrophase in the peripheral organs, and there were significant differences in the kidney and sub gla between the HFD and HFD+FCR groups (). Previous studies have suggested that HFD feeding reduces the amplitude and shifts the peripheral clock acrophase compared to ND feeding (Kohsaka et al. Citation2007; Pendergast et al. Citation2013). These results demonstrate that FCR attenuates the peripheral clock impairment caused by HFD feeding.
Figure 3. The effects of FCR on PER2::LUC expression rhythm in peripheral organs. Representative images (A and B), analyzed waveforms (C–E), amplitudes (F–H), and peak phases (I–K) of in vivo PER2::LUC bioluminescence. Open and closed bars indicate light and dark periods, respectively. Data are presented as the mean ± SEM (the ND and HFD+FCR groups, n = 6; the HFD group, n = 5). * p < 0.05, ** p < 0.01 (one-way ANOVA with Tukey’s multiple comparison test).
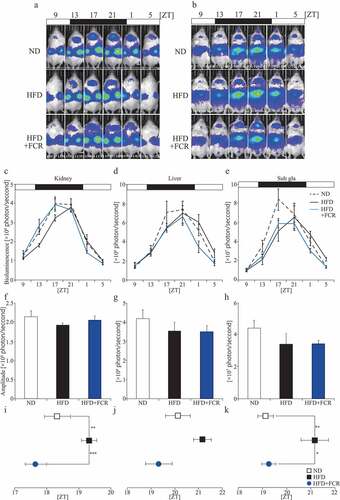
Discussion
Our present study demonstrated that 4DMNob increased the PER2::LUC expression rhythm amplitude and prolonged its period in MEFs. Moreover, we showed that FCR modified HFD feeding-induced locomotor activity rhythm disruption and prevented phase delay of the peripheral clock caused by HFD feeding. Overall, this study suggests that FCR can be a new functional food that contributes to the maintenance of a normal biological rhythm and that 4DMNob is a key FCR effector.
We revealed that 4DMNob increased the PER2::LUC expression rhythm amplitude and period length, and that FCR prevented the HFD feeding-induced disturbance of locomotor activity rhythms. A previous study with MEFs showed that Nob significantly increased the PER2::LUC expression rhythm amplitude and extended its period length compared with the vehicle (Shinozaki et al. Citation2017). Moreover, previous studies on mice have shown that ND with Nob prevents the locomotor activity level decrease induced by aging and that HFD with Nob prevents the same induced by HFD feeding (He et al. Citation2016; Nohara et al. Citation2019). These studies suggest that such effects can be obtained through the action of Nob as an ROR nuclear receptor agonist (He et al. Citation2016; Nohara et al. Citation2019). ROR is one of the clock genes and has been identified as a Bmal1 transcription activator, leading to the enhancement of Per and Cry transcription. The effects of Nob on locomotor activity levels could be induced by the existence of circadian clocks, as Nob had no protective effects on the locomotor activity level decrease caused by HFD feeding in ClockΔ19/Δ19 mutant mice, which is regarded as a model of circadian clock disruption (He et al. Citation2016). Taken together, these results suggest that the effects of 4DMNob on the PER2 expression rhythm and the effects of FCR on locomotor activity rhythm may be mediated through the stimulation of the ROR nuclear receptor.
We also found that FCR modified the HFD feeding-induced locomotor activity level decrease, especially at the beginning of the active period. Previous studies have shown that Nob and 4DMNob stimulate ERK/CREB signaling to increase Cre-mediated transcription levels in neuronal cell lines and hippocampal neurons in primary cultures (Nagase et al. Citation2005; Al Rahim et al. Citation2009). Indeed, synRas mice, in which endogenous Ras activity continues to be regulated and ERK phosphorylation level is upregulated, were found to be more sensitive to light than wild-type mice, because synRas mice showed higher phosphorylation levels of CREB in the SCN induced by light pulses than wild-type mice, especially during the early subjective night period (Serchov et al. Citation2016). Moreover, CBPKIX/KIX mice that do not show stimulus-induced activation of CREB, showed lower locomotor activity levels at the beginning of the active period (Chatterjee et al. Citation2020). Taken together, these results suggest that FCR modified the HFD feeding-induced locomotor activity level decrease at of the time LD switching via the activation of the ERK/CREB signaling.
In this study, we also showed that FCR prevented the HFD-induced impairment of circadian clocks coupled with low amplitude and abnormal phase. As mentioned above, the FCR effect may be explained by the 4DMNob effects on the ROR nuclear receptors and ERK/CREB signaling pathways. In addition to these effects, previous studies indicated that Nob and 4DMNob also have antioxidative effects. Nob reduces intracellular reactive oxygen species (ROS) levels in C2C12 myoblast cells treated with the oxidant tert-butyl hydroperoxide (Nohara et al. Citation2019), while 4DMNob induces anti-oxidative enzymes, such as heme oxygenase-1 and NADH quinone oxidoreductase 1, in RAW 264.7 macrophages treated with lipopolysaccharide (Wu et al. Citation2015a). HFD feeding induces oxidative stress, which prolongs the clock gene expression rhythm in PER2::LUC MEFs (Hsu and Yen Citation2007; Imamura et al. Citation2018); however, in this experiment, we did not observe any prolongation of the free-running period induced by HFD feeding. Therefore, the findings of the present and the related past studies taken together suggest that FCR may be a new potential functional food, which improves the HFD feeding-induced disruption of the circadian clock system through not only the ROR nuclear receptor and ERK/CREB signaling pathway agonists, but also through the enhancement of anti-oxidative effects.
We found that FCR modified the HFD-induced decrease in locomotor activity level and prevented the HFD-induced impairment of peripheral circadian clocks coupled with a phase delay. The circadian clock system closely interacts with the feeding rhythm and regulates it (Kalsbeek et al. Citation2001); feeding is the strongest entrainment factor for peripheral clocks (Tahara and Shibata Citation2016). Previous studies have demonstrated that male mice and ovariectomized mice fed an HFD for 1 week showed dispersed eating behavior, locomotor activity rhythm, and advanced phase of liver molecular rhythm (Pendergast et al. Citation2013; Palmisano et al. Citation2017). Other studies found that mice fed with HFD under a time-restricted feeding regimen showed increased activity toward the end of the active period and improved oscillations of circadian clock components in the liver compared with mice fed with HFD under an ad libitum regimen (Hatori et al. Citation2012). Taken together, these results suggest that the disruption of locomotor activity rhythms interacts with the phase shift of peripheral clocks. However, it is difficult to determine whether the phase shift of the peripheral clock by FCR supplementation is associated with activity rhythm changes or not.
In conclusion, our study revealed that FCR containing a substantial amount of 4DMNob may be a new functional food that maintains healthy biological rhythms, such as clock gene expression rhythm and locomotor activity rhythm. Indeed, FCR modified HFD feeding-induced locomotor activity rhythm disturbance and peripheral clock rhythm delay. However, FCR contains substances other than 4DMNob (Kawahata et al. Citation2017) which could also contribute to its effects; however, the analysis of the same is beyond the scope of this study. Regardless, FCR could still be expected to improve the biological rhythm disturbances caused by aging and those caused by lifestyle-related factors such as work shifts or jetlag.
Supplemental Material
Download PDF (1.4 MB)Acknowledgments
This work was partially supported by a Grant-in-Aid for Early-Career Scientists (19K14018) from JSPS (A.H.), the Council for Science, Technology and Innovation, SIP, “Technologies for creating next-generation agriculture, forestry and fisheries” (funding agency: Bio-oriented Technology Research Advancement Institution, NARO) (S.S.), and a Grant-in-Aid for Scientific Research (S) (26220201) from the Ministry of Education, Culture, Sports, Science and Technology of Japan (S.S.).
Disclosure statement
Atsushi Haraguchi, Shuhei Sato, Tomohiro Yamazaki, Con Ryan, Masataka Sekiguchi, and Shigenobu Shibata: Declarations of interest: None. Shuichi Kusano is an employee of Fuji Sangyo Co., Ltd. The authors declare no other conflicts of interest associated with this manuscript.
Supplementary material
Supplemental data for this article can be accessed here
Additional information
Funding
References
- Al Rahim M, Nakajima A, Saigusa D, Tetsu N, Maruyama Y, Shibuya M, Yamakoshi H, Tomioka Y, Iwabuchi Y, Ohizumi Y, et al. 2009. 4ʹ-Demethylnobiletin, a bioactive metabolite of nobiletin enhancing PKA/ERK/CREB signaling, rescues learning impairment associated with NMDA receptor antagonism via stimulation of the ERK cascade. Biochemistry. 48(32):7713–7721. doi:10.1021/bi901088w
- Albrecht U. 2012 Apr26. Timing to perfection: the biology of central and peripheral circadian clocks. Neuron. 742:246–260. Epub 2012/ 05/01. doi:10.1016/j.neuron.2012.04.006
- Bass J, Takahashi JS. 2010 Dec3. Circadian integration of metabolism and energetics. Science. 330:1349–1354. Epub 2010/ 12/04. doi:10.1126/science.1195027
- Buhr ED, Takahashi JS. 2013. Molecular components of the Mammalian circadian clock. Handb Exp Pharmacol. (217):3–27. Epub 2013/ 04/23. doi:10.1007/978-3-642-25950-0_1
- Chatterjee S, Angelakos CC, Bahl E, Hawk JD, Gaine ME, Poplawski SG, Schneider-Anthony A, Yadav M, Porcari GS, Cassel JC, et al. 2020. The CBP KIX domain regulates long-term memory and circadian activity. BMC Biol. Oct 29;18:155. Epub 2020/ 10/31. 1 10.1186/s12915-020-00886-1
- Gachon F, Nagoshi E, Brown SA, Ripperger J, Schibler U. 2004 Sep. The mammalian circadian timing system: from gene expression to physiology. Chromosoma. 113(3):103–112. Epub 2004/ 09/01. doi:10.1007/s00412-004-0296-2.
- Hara R, Wan K, Wakamatsu H, Aida R, Moriya T, Akiyama M, Shibata S. 2001. Restricted feeding entrains liver clock without participation of the suprachiasmatic nucleus. Genes to Cells: Devoted to Molecular & Cellular Mechanisms. 6(3):269–278. doi:10.1046/j.1365-2443.2001.00419.x.
- Haraguchi A, Fukuzawa M, Iwami S, Nishimura Y, Motohashi H, Tahara Y, Shibata S. 2018. Night eating model shows time-specific depression-like behavior in the forced swimming test. Sci Rep. 8(1):1081. doi:10.1038/s41598-018-19433-8.
- Hastings MH. 1997 Nov1. Circadian clocks. Curr Biol. 711:R670–672. Epub 1998/ 02/28. doi:10.1016/S0960-9822(06)00350-2
- Hatori M, Vollmers C, Zarrinpar A, DiTacchio L, Bushong EA, Gill S, Leblanc M, Chaix A, Joens M, Fitzpatrick JA, et al. 2012. Time-restricted feeding without reducing caloric intake prevents metabolic diseases in mice fed a high-fat diet. Cell Metab. Jun 6;15:848–860. Epub 2012/ 05/23. 6 10.1016/j.cmet.2012.04.019
- Hayasaka N, Yaita T, Kuwaki T, Honma S, Honma K-I, Kudo T, Shibata S. 2007. Optimization of dosing schedule of daily inhalant dexamethasone to minimize phase shifting of clock gene expression rhythm in the lungs of the asthma mouse model. Endocrinology. 148(7):3316–3326. Epub 2007/ 04/05. doi:10.1210/en.2007-0010.
- He B, Nohara K, Park N, Park Y-S, Guillory B, Zhao Z, Garcia JM, Koike N, Lee CC, Takahashi JS, et al. 2016. The small molecule nobiletin targets the molecular oscillator to enhance circadian rhythms and protect against metabolic syndrome. Cell Metab. 23(4):610–621. doi:10.1016/j.cmet.2016.03.007
- Hsu C-L, Yen G-C. 2007. Effect of gallic acid on high fat diet-induced dyslipidaemia, hepatosteatosis and oxidative stress in rats. Br J Nutr. 98(4):727–735. Epub 2007/ 05/03. doi:10.1017/S000711450774686X.
- Imamura K, Yoshitane H, Hattori K, Yamaguchi M, Yoshida K, Okubo T, Naguro I, Ichijo H, Fukada Y. 2018. ASK family kinases mediate cellular stress and redox signaling to circadian clock. Proc Natl Acad Sci U S A. 115(14):3646–3651. Epub 2018/ 03/19. doi:10.1073/pnas.1719298115.
- Kalsbeek A, Fliers E, Romijn JA, La Fleur SE, Wortel J, Bakker O, Endert E, Buijs RM. 2001 Jun. The suprachiasmatic nucleus generates the diurnal changes in plasma leptin levels. Endocrinology. 142(6):2677–2685. Epub 2001/ 05/18. doi:10.1210/endo.142.6.8197.
- Kawahata I, Suzuki T, Rico EG, Kusano S, Tamura H, Mimaki Y, Yamakuni T. 2017. Fermented Citrus reticulata (ponkan) fruit squeezed draff that contains a large amount of 4ʹ-demethylnobiletin prevents MK801-induced memory impairment. J Nat Med. 71(4):617–631. Epub 2017/ 05/09. doi:10.1007/s11418-017-1091-8.
- Kawahata I, Yoshida M, Sun W, Nakajima A, Lai Y, Osaka N, Matsuzaki K, Yokosuka A, Mimaki Y, Naganuma A, et al. 2013. Potent activity of nobiletin-rich Citrus reticulata peel extract to facilitate cAMP/PKA/ERK/CREB signaling associated with learning and memory in cultured hippocampal neurons: identification of the substances responsible for the pharmacological action. J Neural Transm (Vienna). 120(10):1397–1409. Epub 2013/ 04/16. doi:10.1007/s00702-013-1025-x.
- Kohsaka A, Laposky AD, Ramsey KM, Estrada C, Joshu C, Kobayashi Y, Turek FW, Bass J. 2007. High-fat diet disrupts behavioral and molecular circadian rhythms in mice. Cell Metab. 6(5):414–421. doi:10.1016/j.cmet.2007.09.006.
- Lo Y-H, Pan M-H, Li S, Yen J-H, Kou M-C, Ho C-T, Wu M-J. 2010. Nobiletin metabolite, 3ʹ,4ʹ-dihydroxy-5,6,7,8-tetramethoxyflavone, inhibits LDL oxidation and down-regulates scavenger receptor expression and activity in THP-1 cells. Biochim Biophys Acta. 1801(2):114–126. Epub 2009/ 10/13. doi:10.1016/j.bbalip.2009.10.002.
- Nagase H, Yamakuni T, Matsuzaki K, Maruyama Y, Kasahara J, Hinohara Y, Kondo S, Mimaki Y, Sashida Y, Tank AW, et al. 2005. Mechanism of neurotrophic action of nobiletin in PC12D Cells†. Biochemistry. 44(42):13683–13691. doi:10.1021/bi050643x
- Nelson RJ, Zucker I. 1981 May. Photoperiodic control of reproduction in olfactory-bulbectomized rats. Neuroendocrinology. 32(5):266–271. Epub 1981/ 05/01. doi:10.1159/000123171.
- Nohara K, Mallampalli V, Nemkov T, Wirianto M, Yang J, Ye Y, Sun Y, Han L, Esser KA, Mileykovskaya E, et al. 2019. Nobiletin fortifies mitochondrial respiration in skeletal muscle to promote healthy aging against metabolic challenge. Nat Commun. 10(1):392. doi:10.1038/s41467-019-11926-y
- Ohnishi N, Tahara Y, Kuriki D, Haraguchi A, Shibata S, Ebihara S. 2014. Warm water bath stimulates phase-shifts of the peripheral circadian clocks in PER2:: lUCIFERASEmouse. PLoS One. 9(6):e100272–e100272. doi:10.1371/journal.pone.0100272.
- Ohta H, Xu S, Moriya T, Iigo M, Watanabe T, Nakahata N, Chisaka H, Hanita T, Matsuda T, Ohura T, et al. 2008. Maternal feeding controls fetal biological clock. PLoS One. 3(7):e2601–e2601. doi:10.1371/journal.pone.0002601
- Palmisano BT, Stafford JM, Pendergast JS. 2017. High-Fat feeding does not disrupt daily rhythms in female mice because of protection by ovarian hormones. Front Endocrinol (Lausanne). 8:44. Epub 2017/ 03/30. doi:10.3389/fendo.2017.00044.
- Pendergast JS, Branecky KL, Yang W, Ellacott KL, Niswender KD, Yamazaki S. 2013 Apr. High-fat diet acutely affects circadian organisation and eating behavior. Eur J Neurosci. 37(8):1350–1356. Epub 2013/ 01/22. doi:10.1111/ejn.12133.
- Refinetti R, Lissen GC, Halberg F. 2007. Procedures for numerical analysis of circadian rhythms. Biol Rhythm Res. 38(4):275–325. doi:10.1080/09291010600903692.
- Reppert SM, Weaver DR. 2002. Coordination of circadian timing in mammals. Nature. 418(6901):935. 08/29/online. doi:10.1038/nature00965.
- Serchov T, Jilg A, Wolf CT, Radtke I, Stehle JH, Heumann R. 2016 Apr. Ras activity oscillates in the mouse suprachiasmatic nucleus and modulates circadian clock dynamics. Mol Neurobiol. 53(3):1843–1855. Epub 2015/ 03/13. doi:10.1007/s12035-015-9135-0.
- Shinozaki A, Misawa K, Ikeda Y, Haraguchi A, Kamagata M, Tahara Y, Shibata S, Oster H. 2017. Potent effects of flavonoid nobiletin on amplitude, period, and phase of the circadian clock rhythm in PER2:: lUCIFERASEMouse embryonic fibroblasts. PLoS One. 12(2):e0170904–e0170904. doi:10.1371/journal.pone.0170904.
- Sokolove PG, Bushell WN. 1978 May8. The chi square periodogram: its utility for analysis of circadian rhythms. J Theor Biol. 721:131–160. Epub 1978/ 05/08. doi:10.1016/0022-5193(78)90022-X
- Tahara Y, Kuroda H, Saito K, Nakajima Y, Kubo Y, Ohnishi N, Seo Y, Otsuka M, Fuse Y, Ohura Y, et al. 2012. In vivo monitoring of peripheral circadian clocks in the mouse. Curr Biol. Jun 5;22:1029–1034. Epub 2012/ 05/15. 11 10.1016/j.cub.2012.04.009
- Tahara Y, Shibata S. 2016 Apr. Circadian rhythms of liver physiology and disease: experimental and clinical evidence. Nat Rev Gastroenterol Hepatol. 13(4):217–226. Epub 2016/ 02/26. doi:10.1038/nrgastro.2016.8.
- Tahara Y, Shiraishi T, Kikuchi Y, Haraguchi A, Kuriki D, Sasaki H, Motohashi H, Sakai T, Shibata S. 2015 Jun15. Entrainment of the mouse circadian clock by sub-acute physical and psychological stress. Sci Rep. 51:11417. Epub 2015/ 06/16. doi:10.1038/srep11417
- Weaver DR. 1998 Apr. The suprachiasmatic nucleus: a 25-year retrospective. J Biol Rhythms. 13(2):100–112. Epub 1998/ 04/29. doi:10.1177/074873098128999952.
- Wu X, Song M, Qiu P, Li F, Wang M, Zheng J, Wang Q, Xu F, Xiao H. 2018. A metabolite of nobiletin, 4ʹ-demethylnobiletin and atorvastatin synergistically inhibits human colon cancer cell growth by inducing G0/G1 cell cycle arrest and apoptosis. Food Funct. 9(1):87–95. doi:10.1039/C7FO01155E.
- Wu X, Song M, Rakariyatham K, Zheng J, Wang M, Xu F, Gao Z, Xiao H. 2015a. Inhibitory effects of 4′-demethylnobiletin, a metabolite of nobiletin, on 12-O-Tetradecanoylphorbol-13-acetate (TPA)-Induced Inflammation in mouse ears. J Agric Food Chem. 63(51):10921–10927. Epub 2015/ 12/19. doi:10.1021/acs.jafc.5b05156.
- Wu X, Song M, Wang M, Zheng J, Gao Z, Xu F, Zhang G, Xiao H. 2015b. Chemopreventive effects of nobiletin and its colonic metabolites on colon carcinogenesis. Mol Nutr Food Res. 59(12):2383–2394. Epub 2015/ 10/07. doi:10.1002/mnfr.201500378.
- Yasuda T, Yoshimura Y, Yabuki H, Nakazawa T, Ohsawa K, Mimaki Y, Sashida Y. 2003. Urinary metabolites of nobiletin orally administered to rats. Chem Pharm Bull (Tokyo). 51(12):1426–1428. doi:10.1248/cpb.51.1426.
- Yoo S-H, Yamazaki S, Lowrey PL, Shimomura K, Ko CH, Buhr ED, Siepka SM, Hong H-K, Oh WJ, Yoo OJ, et al. 2004. PERIOD2::LUCIFERASEreal-time reporting of circadian dynamics reveals persistent circadian oscillations in mouse peripheral tissues. Proc Natl Acad Sci U S A. 101(15):5339–5346. Epub 2004/ 02/12. doi:10.1073/pnas.0308709101.