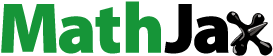
ABSTRACT
This study investigated from a longitudinal retrospective perspective what characterizes and predicts 6th graders (Mage = 12.95, SD = 0.27) with low (LMA) or high (HMA) math achievement concerning the development of early domain-specific and domain-general cognitive abilities. They were examined and compared to average achievers (n = 88) at four-time points from kindergarten (Mage = 6.58, SD = 0.36) to third grade (Mage = 9.53, SD = 0.33). The LMA (n = 27) or HMA (n = 41) children exhibited persistent multi-weakness and multi-strength profiles, respectively, present already prior to formal schooling. The cognitive profiles of the two groups, and their development, were mostly qualitatively similar, but there were also important qualitative differences. Logistic regression analyzes showed that superior verbal arithmetic, logical reasoning, and executive functions are vital for developing superior mathematical skills while inferior verbal arithmetic, logical reasoning, and spatial processing ability constitute unique potential risk factors for low mathematical skills.
In order to deal with many routine activities such as shopping, paying bills, or maintaining a monthly budget, one needs to possess sufficient mathematical competencies (Bottge, Citation2001; Butterworth et al., Citation2011; Duncan et al., Citation2007; see McCloskey, Citation2007 for a review). Individuals failing to acquire these important skills may encounter difficulties with finding employment that makes them self-supporting and they are also at greater risk of developing physical and mental illness (Butterworth, Citation2010; Kucian & von Aster, Citation2015). Against this background, an increasing number of studies have focused on children with severe difficulties in acquiring basic math skills, that is, mathematical learning disabilities (MLD) or developmental dyscalculia (DD). Research shows that these children have severe and persistent difficulties with multiple domains of basic mathematics, such as single-digit arithmetic, arithmetic fact retrieval, written multi-digit calculation, arithmetic problem solving, approximate arithmetic, conceptual understanding of place value, calculation principles and time telling (Andersson, Citation2008, Citation2010; Hanich et al., Citation2001; Jordan & Hanich, Citation2003; Jordan et al., Citation2003; Ostad, Citation1998).
Research aiming at identifying the origin of MLD/DD and its defining deficits remain inconclusive. Some suggest that an impairment in the core number system demarcates MLD/DD, while others argue that an impaired connection between the core number system and the symbolic number system (digits; counting words) better describes the etiology (Landerl et al., Citation2004; Mazzocco et al., Citation2011; Olsson et al., Citation2016; Piazza et al., Citation2010; Rousselle & Noël, Citation2007; Skagerlund & Träff, Citation2014, Citation2016). However, another line of research has found that more general cognitive abilities are involved in MLD/DD, rather than being circumscribed to basic number processing skills. This line of research finds evidence that deficits in working memory, long-term memory, executive functions, spatial processing, general processing speed, phonological processing, or other domain general cognitive abilities might be underlying factors of MLD/DD (Andersson & Östergren, Citation2012; Passolunghi & Siegel, Citation2001, Citation2004; Mammarella et al., Citation2018; Raghubar et al., Citation2010; Skagerlund & Träff, Citation2014; Szücs et al., Citation2013).
In contrast to the increasing number of studies performed on children with MLD/DD, a much smaller amount of research has focused on children with low math achievement (LMA) and even fewer studies have investigated children with high math achievement (HMA). Although it is of utmost importance to investigate the etiology of MLD/DD, one should bear in mind that a substantial portion of children fail at learning adequate mathematical skills while not fulfilling the criteria to be characterized as MLD/DD. This population of children may exhibit different cognitive profiles than MLD/DD prior to formal schooling and during the early school years. Thus, it is important to raise attention to children that are at risk of having to struggle with reaching mathematical competency. Doing this may allow us to identify children that are at risk as early as possible in childhood. Also, by separating children with HMA from typical achievers of math achievement (AMA), we can differentiate what constitutes typical abilities that distinguish AMA from LMA and HMA. The primary purpose of the current study was therefore to explore from a longitudinal retrospective perspective what characterizes children with LMA and HMA relative to children with AMA concerning the development of number and arithmetic skills and general cognitive abilities from 6 years to 9 years of age. The secondary purpose was to identify what skills and abilities that constitute the primary predictors of LMA group membership and HMA group membership.
Mathematical development in children with low math achievement
To date, only three longitudinal studies have investigated mathematical development in children with LMA (Geary et al., Citation2009, Citation2012; Murphy et al., Citation2007). Murphy et al. (Citation2007) followed 42 children with LMA (11th – 25th percentile) and 22 with MLD from kindergarten (Mage = 5.86, SD = 0.49) to third grade. They used the Test of Early Math Ability, (TEMA-2) to monitor the children’s progress in basic mathematics. The TEMA-2 is a comprehensive test tapping several basic number and arithmetic skills (magnitude judgments, counting, reading and writing numerals, calculation, place value, arithmetic facts). The LMA and MLD children lagged behind their average math achieving peers (AMA; above the 25th percentile) from the start in kindergarten up to grade 3, but the LMA children displayed the same “growth rate” as the children with AMA. In contrast, the MLD children displayed a smaller growth on TEMA-2 compared to the AMA and LA groups. Geary et al. (Citation2009) used latent class trajectory analysis to identify children with LMA (n = 123) and MLD (n = 14) to monitor their mathematical development from kindergarten to third grade. The LMA and MLD children’s addition fact retrieval performance was lower compared to the AMA children during all four years, kindergarten up to grade 3. Geary et al.’s (Citation2012) five-year prospective study confirms previous results (i.e., Geary et al., Citation2009; Murphy et al., Citation2007), as their LMA children (n = 29) and MLD children (n = 16), identified by model-based clustering, displayed inferior addition fact retrieval from grade 1 to 5. However, the gap decreased from grade 2 to grade 5. In contrast, in grade 1 the AMA children’s skill to solve simple and complex addition problems were superior relative to the LMA children, who in turn were superior compared to the MLD children. However, these group differences (i.e., AMA group vs. LMA group) disappeared for simple problems in grade 2 and for complex problems in grade 3.
These few longitudinal studies suggest that children with LMA or MLD have persistent difficulties with learning arithmetic facts and/or retrieving these learned facts from long-term-memory (see also Geary et al., Citation2007). Both groups, but especially the MLD group, also appear to have initial (grade 1 and 2) developmental delays concerning mental computation of simple (3 + 4) and complex (16 + 7) addition problems.
Number processing skills in children with low math achievement
Empirical findings indicate that children with LMA also have difficulties with basic number skills in addition to their arithmetic weaknesses. Murphy et al. (Citation2007) found that the counting skills of the AMA group in grade 1 and 2, but not grade 3, were superior relative to the LMA group who in turn were superior compared to the MLD group. Prior studies show that children with LMA perform digit and numerosity comparisons at similar levels as children with AMA (Cowan & Powell, Citation2014; Desoete et al., Citation2012; Mazzocco et al., Citation2011; De Smedt & Gilmore, Citation2011; Stock et al., Citation2010). Thus, children with LMA appear to have an intact core number system and no problems with accessing the underlying magnitue representations from symbols. However, children with LMA have been shown in a number of studies by Geary and colleagues (Geary et al., Citation2009, Citation2007; Cowan & Powell, Citation2014) to display inferior number line estimation performance (mean estimation error) compared to children with AMA. This task requires the child to place a set of Arabic numerals onto a physical number line (e.g., 0–100) in a linear fashion consistent with the formal base-10 number system (Geary et al., Citation2009, Citation2012; Cowan & Powell, Citation2014). Thus, these contradictory findings regarding LMA children suggest that they have difficulties concerning their understanding of the linear base-10 number system (i.e., number line estimation; counting), while their core number system per se (numerosity comparison) and rapid access to this system via symbols (i.e., digit comparison) seem to be intact. Furthermore, longitudinal data suggest that the LMA children’s problems with number line estimation are due to a developmental delay as they, with instruction on the linear base-10 number system, gradually catch up with their AMA peers (Geary et al., Citation2012, Citation2008).
General cognitive abilities in children with low math achievement
Up to now, eight studies have examined general cognitive abilities in children with LMA. Among the abilities, working memory has received most attention, with mixed findings. In Geary et al. (Citation2007, Citation2009) the LMA children’s performance on measures tapping the three components (central executive, phonological loop, visual-spatial sketchpad) of Baddeley’s (Citation1990) working memory model were superior compared to the MLD children, but equal to the AMA children. Almost identical findings were obtained by Geary et al. (Citation2008) as the LMA group performed the tasks measuring the central executive and the visual-spatial sketchpad tasks as good as the AMA group, while their phonological loop performance was poorer than the AMA group. The opposite results were obtained in Geary et al.’s (Citation2012) five-year prospective study. The LMA children’s central executive and visual-spatial sketchpad performances were lower relative to the AMA children, while the two groups’ phonological loop performance were equal (cf. Cowan & Powell, Citation2014). The LMA group displayed superior capacity regarding all three working memory components compared to the MLD group. In a recent study Mammarella et al. (Citation2018) examined visual-spatial working memory capacity in children with LMA and MLD using three different types of tasks. Compared to AMA children, the LMA children displayed lower static visual-spatial short-term memory capacity and sequential visual-spatial short-term memory capacity. In contrast, the LMA children’s visual-spatial working memory capacity were equal to the AMA children’s capacity. The MLD group, on the other hand, displayed inferior performance on all tasks compared to the AMA group, while the MLD and LMA performed equal on all tasks.
In addition to weaknesses in working memory functioning, a number of studies have found that children with LMA have lower intelligence than children with AMA (Geary et al., Citation2009, Citation2012, Citation2008; Cowan & Powell, Citation2014; Murphy et al., Citation2007).
In contrast to weaknesses in working memory and intelligence, children with LMA usually possess average word decoding ability (i.e., reading ability; Geary et al., Citation2008, Citation2012; see also Murphy et al., Citation2007 for different findings) and RAN (Rapid Automatic Naming) ability (Geary et al., Citation2008, Citation2012; Murphy et al., Citation2007; see also Geary et al., Citation2007 for different findings).
In Murphy et al. (Citation2007)’s longitudinal study, both the LMA and the MLD children displayed weak spatial processing ability in kindergarten relative to the AMA children. However, in grade 3, the difference between the LMA and the AMA groups was gone, but the MLD group still displayed weaker spatial processing (cf. Skagerlund & Träff, Citation2014). Murphy et al. (Citation2007)’s finding is consistent with the view that spatial processing and representations support young children’s arithmetic performance during the early phases of arithmetic development (Andersson & Lyxell, Citation2007; McKenzie et al., Citation2003; Rasmussen & Bisanz, Citation2005). It is also assumed that mathematical problems that are complex, novel, ill-defined or require transformations are represented in a spatial format (Andersson & Lyxell, Citation2007; Geary, Citation1993; Kaufmann, Citation1990; Mix & Cheng, Citation2012; Van Garderen, Citation2006).
This combination of general cognitive weaknesses should theoretically have a devastating impact on these children’s mathematical learning (Geary et al., Citation2012; Cirino, Citation2011; LeFevre et al., Citation2010; Träff, Skagerlund et al., Citation2017).
Children with high math achievement
Research focusing on children with HMA are scarce. Heine et al. (Citation2010) provide a rare exception as they examined 22 eight-to-nine-year olds performing at or above the 95th percentile on a standardized math test. The HMA children displayed superior intelligence and working memory capacity. However, the superior working memory capacity was limited to the central executive and phonological loop functioning. They did not demonstrate higher visual-spatial working memory compared to controls. Moreover, they showed superior performance on rapid object and digit naming (i.e., RAN ability). In Geary et al.’s (Citation2009) longitudinal study, the 15 HMA children’s basic arithmetic skills (fact retrieval, number sets test) were superior to the AMA children during all 4 years (kindergarten to third grade). However, the HMA children’s counting and number line estimation performance were comparable to that of the AMA children. Furthermore, HMA children have shown to possess an average numerosity comparison capacity (i.e., core number system; Mazzocco et al., Citation2011). Contrary to Heine et al. (Citation2010), the HMA group in Geary et al. (Citation2009) did not have superior working memory capacity in relation to any of the three components, but they displayed superior intelligence. Due to the almost complete lack of prior studies, it is not possible to draw any firm conclusions regarding domain-specific and domain-general skills in children with HMA. Thus, more research studying cognitive abilities in children with HMA is most needed.
In summary, the available research indicates a multi-weakness view on children with LMA as this population exhibit difficulties in both domain-specific number and math skills as well as domain-general abilities. However, their number system(s) tapped by either numerosity comparison or digit comparison appears to be intact, while their complex number processing skills (number line estimation) are weak. Low working memory capacity and intelligence seem to be other cognitive characteristics of this group of children. However, it should be noted that research focusing on working memory and short-term memory capacity have provided mixed results.
The present study
The present study was founded on two theoretical assumptions usually included in contemporary models of mathematical development (Geary, Citation2013; Cirino et al., Citation2016; Fuchs et al., Citation2010; LeFevre et al., Citation2010; Träff et al., Citation2018; Von Aster & Shalev, Citation2007).
First, a hierarchical view of mathematical development in children is presumed. This means that basic number processing skills and arithmetic skills are believed to be important prerequisites to acquire and master later complex mathematic skills (Cirino et al., Citation2016; Dowker, Citation2005; Namkung & Fuchs, Citation2016; Seethaler et al., Citation2011; Träff et al., Citation2018; Vukovic et al., Citation2014).
Second, the development of different hierarchical levels of mathematics is also dependent upon domain-general cognitive abilities (e.g., logical reasoning, working memory, processing speed). For example, the rate of learning new mathematical concepts and material is influenced by a child’s level of intelligence. Thus, both domain-specific number and arithmetic abilities and domain-general cognitive abilities underlie children’s proficiency in later developing mathematical skills (Cirino et al., Citation2016; Geary, Citation2004; Geary & Hoard, Citation2005; Träff, Citation2013). Given the theoretical assumptions and prior empirical findings, the overall question addressed in the study was: Why do some children have poor math skills while other children have very good math skills? Assuming that part of the answer to this question can be found in these children’s cognitive development, the study explored from a longitudinal retrospective perspective what characterizes 6th graders with LMA and HMA relative to children with AMA concerning the development of number and math skills and general cognitive abilities from 6 years to 9 years of age. The study also explored what skills and abilities that constitute the primary predictors of LMA group membership and HMA group membership.
In contrast to prior research the present study focused on older children. Sixth graders were chosen to reduce the risk of poor math achievement being due to developmental delays or lack of math instructions. Another reason for selecting sixth graders is that they are further along in their mathematical development, that is, beyond arithmetic. All in all, by selecting sixth graders, stable math achievement groups were obtained. These groups were not based on only one aspect of mathematics, that is arithmetic.
The following specific research questions were addressed:
1. Did children with LMA in grade 6 have difficulties with domain specific number and math skills and/or domain general cognitive skills already prior to formal schooling, at age 6, or did their difficulties develop successively during the early years of formal schooling (i.e., grade 1–3)? It was expected that the children with LMA should display a multi-weakness cognitive profile involving difficulties with both domain specific number and math skills and domain general abilities.
2. Did children with HMA in grade 6 have superior domain-specific number and math skills and/or domain-general cognitive skills already prior to formal schooling, at age 6, or did their superior skills develop successively during the early years of formal schooling (i.e., grade 1–3)? It was expected that the children with HMA should display a multi-strength cognitive profile involving both domain specific and domain general abilities.
3. Do the LMA and HMA children develop qualitatively similar or different cognitive profiles? For example, do the LMA children have or develop low working memory capacity while the HMA children have or develop superior working memory capacity compared to the AMA children? Or is low/high working memory capacity only a distinguishing feature for one group?
4. What are the primary predictors of LMA group membership and HMA group membership? It was assumed that both domain specific number and arithmetic skills and domain general abilities should constitute primary predictors of LMA group membership and HMA group membership. Are LMA group membership and HMA group membership predicted by the same skills and abilities?
To address the research questions samples of children with LMA, HMA, and AMA were assessed on a comprehensive test-battery from kindergarten to third grade. The task selection was founded on the above reviewed literature, contemporary theoretical models and research regarding the cognitive mechanism underlying children’s mathematical performance and development (Cirino et al., Citation2016; Fuchs et al., Citation2010; Geary, Citation2004; Geary & Hoard, Citation2005; LeFevre et al., Citation2010; Träff, Citation2013; Träff et al., Citation2018).
Method
Participants
Three-hundred and fifteen six-year-olds attending 22 schools in a city (155 000 inhabitants) located in the southern part of Sweden were initially tested during the first measurement point in kindergarten. Six years later, in grade 6, 261 children remained in the research project and had completed all four measure points, that is, 54 children withdrew. The main reason for withdrawing from the study was that the family moved to another part of the country. The children were recruited by means of a letter of consent that they brought home to their parents from school. All children with written parental consent were included. The children were mostly from middle-class families and all were fluent speakers of Swedish, had normal or corrected-to-normal visual acuity, and no hearing loss. Children diagnosed with neuropsychological disturbances (e.g., ADHD) were excluded from the study.
The large majority of previous studies have used standardized math test and percentile-cutoff criteria to classify children as low, average or high math achievers. A few studies have used latent class trajectory analysis or model-based clustering to classify children. In contrast, the present study used a different approach by using two criteria to classify children as low, average or high math achievers. 1) The curriculum-based math rating on a scale from A-F the child received at the end of 6th grade. This school grade rating is based on the child’s overall math accomplishment during grade 6. As such, it constitutes a reliable (long-term) and broad indicator of achievement (arithmetic, measurement, geometry, fractions, algebra, probability and statistics) based on different sources of information such as participation in classes or written examinations (see Roth et al., Citation2015; Vilia et al., Citation2017). Grade A means that the child has achieved all educational goals stipulated in the curriculum in full, whereas grade E means meeting the minimum requirements for a passing grade. 2) The result (rating from A-F) of a comprehensive curriculum based standardized mathematics test was performed in April in grade 6. This standardized mathematics test is developed and administered by the Swedish National Agency for Education and covers many areas of mathematics: Arithmetic, measurement, geometry, fractions, algebra, probability and statistics. The correlation between the two criteria calculated on the whole initial sample was r = .94, p <.001.
Of the 261 remaining children, 43, 109 and 38 received A, C/D or E on both criteria, respectively, on a scale from A-F. Thirty-five children were excluded as they performed below −1.00 standard deviation on the WISC-IV Matrices according to the norms in Kindergarten, grade 1 or 2. The final sample of 156 children (Kindergarten Mage = 6.58, SD = 0.36; 3th grade Mage = 9.53, SD = 0.33; 6th grade Mage = 12.95, SD = 0.27) consisted of 41 (20 boys) HMA, 88 AMA (51 boys), and 27 LMA (14 boys) as they obtained the same grade, A, C/D or E, on both criteria in 6th grade, respectively.
In the total Swedish population of 6th graders, 10.5% achieved grade A in math, 15.4% achieved grade B, 22.0% achieved grade C, 20.2% achieved grade D, 22.4% achieved grade E. and 9.4% achieved grade F (see the Swedish National Agency for Education). Thus, children belonging to the LMA, AMA and the HMA groups had curriculum-based math rating scores between the 9.50th and the 32th percentile, between the 33th and the 74.0th percentile, and above the 89.50th percentile, respectively. The number of girls and boys in the three groups was approximately equal, .
This research project has been approved by the regional ethics committee in (Blinded), (Blinded, protocol number 33–09).
General data collecting procedure
This longitudinal retrospective study involved four measurement points, starting in kindergarten and proceeded during three consecutive years, grades 1, 2 and 3. During the four measurement points the children performed a total of 14 tasks. Seven of the tasks were administrated during four or three years, from kindergarten to 3th grade or 2nd grade. Seven additional tasks were only administrated during one or two years. The administration of the different tasks during which year are displayed in (see Results section).
Table 1. Mean achievement (SD) on the arithmetic and number processing tasks by achievement group and time.
During the first three measurement points (kindergarten, grades 1 and 2) all tasks were administered in three to six individual sessions. On the 4th measurement point, the tasks were administered in a group session, and two to four individual sessions. Three tasks were administered during the group sessions: reading comprehension, calculation, and addition fluency. The remaining tasks were administered during the individual session.
During all four measurement points the total test time for each child was approximately 120 min. All test sessions included breaks, but the duration varied from 15–60 minutes (i.e., lunch break). Each child’s sessions were performed within one month. The same test order was used for all children and all testing was performed in the child’s school. All instructions regarding the tasks were presented orally. The data collection was performed from October to May each year by ten trained experimenters (including the second and third authors).
Arithmetic tasks and procedure
Nonverbal arithmetic
This task was derived from Jordan et al. (Citation2006). The experimenter and the child were sitting opposite each other. The experimenter placed a number of coins in front of the child (e.g., 4) and said how many coins there were. A screen was set in front of the coins so that the child could not see them. Then the experimenter added (e.g., 4 + 2) or removed (e.g., 4– 2) the number of coins that the problem required. The coins were added/removed one by one, and the experimenter said how many coins that have been added/removed. The task was to say or show with coins how many coins were behind the screen. The test material consisted of eight addition problems (2 + 1, 3 + 2, 4 + 3, 2 + 4, 3 + 5, 6 + 4, 5 + 6, 7 + 8) and eight subtraction problems (3– 1, 7– 3, 5– 2, 6– 4, 9– 5, 10– 6, 11– 5, 13– 7). Testing ended when a child failed three consecutive trials. In this task, the arithmetic problems were represented by coins. Thus, the task was assumed to assess the ability to understand and perform quantitative transformations (i.e., arithmetic operations) without imposing high demands on understanding the words and the syntactic structure of the task. Split-half reliability was r = .76.
Verbal arithmetic
This task was developed by the first author (see Elofsson et al., Citation2016). The child was asked to orally solve presented addition (2 + 1, 3 + 2, 4 + 3, 2 + 4, 3 + 5, 6 + 4, 5 + 6, 7 + 8, 9 + 12, 13 + 12, 14 + 11, 12 + 13, 14 + 13, 17 + 15, 13 + 19, 23 + 18, 21 + 17, 26 + 23) and subtraction problems (3–1, 7–3, 5–2, 6–4, 9–5, 10–6, 11–5, 13–7, 16–9, 21–7, 25–9, 24–12, 23–14, 21–16, 25–13, 28–17, 21–18, 32–13). The tasks were designed such that test items became successively more difficult. Upon the child’s request, the experimenter would present the problem multiple times if needed. The child responded with a number word. All addition problems were phrased, “If you have [n] buns and I have [n] buns, how many buns do we have together?” All subtraction problems were phrased, “If you have [n] buns and you give me [n] buns, how many buns do you have left?” (cf. Jordan et al., Citation2003). All children started with the addition task, which was followed by the subtraction task. Testing ended when a child failed three consecutive trials. Split-half reliability was r = .82.
Addition fluency
The fluency task was a paper-and-pencil test (see Träff et al., Citation2018). The child was instructed to solve as many single-digit addition problems (e.g., 2 + 1; 8 + 4, 9 + 9) as possible during one minute. The material consisted of 75 addition problems presented in three columns on a sheet of paper. The problems were presented horizontally, and the child responded in writing. Test–retest reliability of r = .68 was established by computing the correlation between year 3 and 4.
Written multi-digit calculation
In this paper-and-pencil test, developed by the first author (Träff et al., Citation2020), the child was asked to solve six addition and six subtraction problems (e.g., 57 + 42; 545 + 96; 4203 + 825; 78 – 43; 824 – 488; 11305 – 5786) in eight minutes. The problems were presented horizontally, and the children responded in writing. All problems, except four, involved carrying or borrowing. Split-half reliability was r = .76.
Number processing tasks and procedure
Counting
This task was developed by the first author (Träff et al., Citation2020) and comprised counting to 50, counting to 30 twice and counting from a given number. A starting number was given, and the child was stopped when they had counted to a specific number (8–13, 24–29, 63–68, 85–90). The child had to specify the number following a given number (6, 15, 53, 69, 99). The child was also asked to count backward starting from three different numbers (10, 15, 23), and to name the number prior to the given number (9, 17, 28, 40, 80). Possible scores were between 0 and 20. Cronbach’s alpha was .90.
Digit comparison
This task was developed by the first author and used to assess the child’s ability to quickly and accurately access the underlying core number representation from symbols. The test material consisted of two sheets of paper containing a total of 28 pairs of digits. The first sheet of paper contained digit pairs with a numerical distance of 1 (e.g., 5 6, 4 3, 8 9), and the second sheet of paper contained digit pairs with a numerical distance of 4–5 (e.g., 7 2, 3 8, 6 1). The task was to decide as fast and accurately as possible which of the two digits was numerically larger. The child responded by crossing out the largest of the two digits. Response times were used as the dependent measure. For each child, a total response time was calculated based on the performance on the two sheets of paper. Split-half reliability calculated between the two sheets of paper was rsh = .89.
Number line estimation
This task was developed by the first author (see Andersson & Östergren, Citation2012) and used as an index of the child’s understanding of the linear base-10 number system. The child was instructed to indicate with a pencil where a particular number would go on a 0–100 number line. Materials consisted of a booklet with 16 pages, each with a 21 cm horizontal line printed across the page. Each of the 16 experimental problems had the number 0 printed at the left end of the line and the number 100 printed at the right end of the line. The number to be estimated was presented 2 cm above the center of the line. Accuracy of estimates in relation to a perfect linear function, that is, percentage absolute error was used as the dependent measure. Test–retest reliability of r = .57 was established by computing the correlation between year 1 and 2.
Cognitive tasks and procedure
Matrix reasoning
This subtest is from the Wechsler’s Intelligence Scale for Children (WISC-IV; Wechsler, Citation2003) and was used to assess non-verbal logical reasoning. The task is to select the missing piece in a larger design to complete the design. Responses could be verbal or involve pointing to the correct piece. For every item, there were five different response alternatives. After four consecutive wrong answers or four wrong answers out of five trials, the test was stopped. Test–retest reliability of r = .61 was established by computing the correlation between year 1 and 2.
Verbal working memory
The child was presented with sequences of words. The task was to decide whether each presented word was an animal or not (see Träff, Olsson et al., Citation2017). The child had to answer “yes” (animal) or “no” (not animal) before the next word was presented. At the end of the sequence the child had to recall in correct serial order the words in the sequence. The first span size employed was two, the next was three, four, five, six and seven. All children were presented up to span size four, but if the child managed to recall in correct serial order a trial on span four, testing continued until the child failed both trials of the same span length. Forty-three percent of the words were animals. The experimenter read the sequences of words from a sheet of paper. Verbal working memory span was measured as the longest sequences of words remembered in correct serial order, plus 0.50 points for each trial the child managed to replicate in the same span length. The maximum score was 7.50. Split-half reliability was r = .73.
Phonological fluency
This task was used to assess executive functions such as coordinating and monitoring retrieval processes from long-term memory and inhibiting incorrect responses (Andersson & Lyxell, Citation2007; Passolunghi & Lanfranchi, Citation2012. The child had to generate as many words as possible from two initial phoneme categories (/f/, /s/). Sixty seconds were allowed for each category. The number of words correctly retrieved within the allowed time interval was used as the dependent measure. Split-half reliability was r = .80.
Color-naming task
This task assessed rapid and automatized access to information in long-term memory (see Träff, Skagerlund, et al., Citation2017). The material consisted of two sheets of paper, containing a total of 30 red, green, blue, black and yellow XXX (Arial, 22-point font), presented in two columns with 15 in each column. The task was to name the color of the XXX as quickly as possible without making any errors. A stopwatch was used to measure the total time it took to name the 30 XXX. The combined response times for the two trials were used as the dependent measure. Split-half reliability was r = .92.
Mental rotation task
This task was used as a measure of spatial processing (Rüsseler et al., Citation2005). The material consisted of alphabetic letters, one letter per trial, a total of 16 trials. A target letter was located on the left side, accompanied by four comparison letters located on the right side adjacent to the target. The comparison letters always consisted of two “correct” and two “incorrect” letters. The task was to identify the two matching letters, which prompted a mental rotation, and by marking them with a pen. Inverted versions of the target (i.e., visually mirrored) were used as incorrect comparison stimuli. All letters were rotated only in the picture-plane and in one of six rotation angles (45°; 90°; 135°; 225°; 270°; 315°). Both correct comparison letters had to be marked to yield a point for each trial, yielding a maximum score of 16. The child was allowed 2 minutes to perform the task. Split-half reliability was r = .85.
Word decoding
In this task (Elwér et al., Citation2009), the child had to read as many words as possible from a list of 100 words during 45 seconds. The child was instructed to read as quickly as possible without making any errors. All children performed an A- and B-version beginning with the A-version. The combined number of correctly read words from the two versions was used as an index of word-decoding skill. Split-half reliability was r = .97.
Reading comprehension
This reading comprehension test was developed by Malmquist (Citation1977) and consisted of a short story read by the child. The narrative took the form of a fairy-tale, and scattered throughout the text were single missing words replaced by a blank space and a bracket containing four words. The child then selected which words made the most sense in terms of the sentence and the story, and underlined their answer. This reading test contained twenty items (i.e., missing words) scattered evenly throughout the story. The number of correct items selected within four minutes was the dependent measure. Split-half reliability was r = .96.
Results
Means and standard deviations for the arithmetic and number processing tasks by achievement group and time are displayed in . displays descriptive statistics for the domain-general tasks by achievement group and time. To address research questions 1–3, two-way (groups by measure points) ANOVAs and one-way ANOVAs (groups) were computed to examine group effects, effects of time, and group by time interaction effects. As the main effect of time was of minor interest, this effect was only reported but not further discussed. Moreover, group differences between the LMA and HMA were not reported or discussed.
Table 2. Mean cognitive achievement (SD) by achievement group and time.
The Šidák test procedure were used as post hoc test and and Cohen’s d were used as effect sizes (Cohen, Citation1988). An
of .25 or larger represents a large effect, whereas an
between .10 to .24 is of medium size. An
of .09 or less is considered to be small. A Cohen’s d of 0.80 or larger represents a large effect, whereas a Cohen’s ds between 0.30 and 0.79 is of medium size. A Cohen’s d of 0.20 or less is considered to be small.
As the present study entailed a large number of ANOVAs only significant effect sizes larger than = .05 were considered. Covariates were not included in the ANOVAs because the aim was to describe the characteristics of the LMA and HMA groups at each measure point (cf. Murphy et al., Citation2007). To address research question 4, multiple binary logistic regression analyses were computed using a forward stepwise conditional method.
It was expected that the children with LMA should display a multi-weakness cognitive profile involving difficulties with both domain specific number and math skills and domain general abilities.
Arithmetic
Nonverbal arithmetic
The one-way ANOVA performed on this basic arithmetic task, implemented in kindergarten only, revealed a medium sized group effect, F(2, 153) = 16.744, p <.001, = .180. Post hoc testing showed that the HMA group outperformed the AMA group (d = 0.68), while the AMA group outperformed the LMA group (d = 0.74).
Verbal arithmetic
A 3 (groups) x 3 (time points) ANOVA showed a large group effect, F(2, 152) = 54.800, p <.001, = .419, a large time effect, F(2, 304) = 517.088, p <.001,
= .773, and a small time x group interaction, F(4, 304) = 2.949, p = .020,
= .037. Post hoc testing showed that the HMA group outperformed the AMA group (d = 1.52), while the AMA group outperformed the LMA group (d = 1.04).
Addition fluency
A 3 (groups) x 2 (time points 3 and 4) ANOVA displayed a medium sized group effect, F(2, 147) = 17.5156, p <.001, =.192, a large time effect F(1, 147) = 50.264, p <.001,
=.255, but no time x group interaction, F(2, 147) = 1.767, p = .174,
=.023. Post hoc testing revealed that the LMA group was outperformed by the AMA group (d = 0.76), who in turn was outperformed by the HMA group (d = 0.74).
Written multi-digit calculation
A large group effect was found on the calculation task, F(2, 148) = 30.785, p <.001, = .294, and a large time effect, F(1, 148) = 144.440, p <.001,
= .494, but no interaction effect, F(2, 148) = 1.471, p = .233,
= .019. Sidak testing revealed that the HMA group scored significantly higher than the AMA group (d = 1.09), whereas the LMA group scored significantly lower than the AMA group (d = 0.88).
Number processing
Counting
Counting skill was assessed at the first measure point only and a medium sized group effect was obtained, F(2, 153) = 18.781, p <.001, =.197. The HMA group outperformed the AMA group (d = 0.86), and the AMA group outperformed the LMA group (d = 0.65).
Digit comparison
A 3 (groups) x 4 (time points) ANOVA showed a medium sized group effect, F(2, 148) = 11.527, p <.001, =.135, a large time effect, F(3, 444) = 191.190, p <.001,
= .564, and a small time x group interaction, F(6, 444) = 3.615, p = .002,
= .047. Post hoc testing showed that the AMA group performed faster than the LMA group (d = 0.62), while the AMA and HMA groups were equally fast (p = .059).
Number line estimation
A 3 (groups) x 3 (time points) ANOVA showed a large group effect, F(2, 153) = 26.577, p <.001, = .258, a large time effect, F(2, 306) = 206.464, p <.001,
= .574, and a small time x group interaction effect, F(4, 306) = 4.961, p = .001,
= .061. Post hoc testing showed that the HMA group scored more accurate than the AMA group (d = 1.05), while the AMA group scored more accurate than the LMA group (d = 0.76).
The interaction effect indicates that the groups progressed at different rates during the three years. The LMA children’s error scores decrease with 14.18 points from measure point 1 to 3, whereas the corresponding decrease for the AMA and HMA groups were 10.22 points, and 7.86 points, respectively. Tests of simple effects ANOVAs were computed to evaluate the different growth rates. If the LMA children caught up with the AMA children and if the AMA group caught up with the HMA children. Significant group effects were obtained on the first, F(2, 459) = 30.503, p <.01, the second measure points, F(2, 459) = 16.158, p <.01, but not on the third measure point, F(2, 459) = 4.209, p >.01. During the first and second measure points, the HMA group scored more accurate than the AMA group (d = 0.64; 1.16), while the AMA group scored more accurate than the LMA group on the first measure point (d = 0.71), but not on the second (p >.01).
General cognitive tasks
Matrix reasoning (WISC4)
A 3 (groups) x 3 (time points 1, 2 and 3) ANOVA displayed a large group effect, F(2, 153) = 40.295, p <.001, = .345, a large time effect, F(2, 306) = 148.878, p <.001,
= .493, but no time x group interaction, F(4, 306) = 1.3455, p = .253,
= .017. The HMA group scored higher than the AMA group (d = 1.19), while the AMA group scored higher than the LMA group (d = 0.93).
Verbal working memory
A 3 (groups) x 4 (time points) ANOVA showed a medium sized group effect, F(2, 147) = 8.653, p <.001, = .105, a large time effect, F(3, 441) = 57.342, p <.001,
= .281, but no time x group interaction, F(6, 441) = 0.815, p = .558,
= .011. Post hoc testing showed that the HMA group obtained higher scores than the AMA group (d = 0.59), while the scores of the AMA and LMA groups were equal (p = .143).
Phonological fluency
The 3 (groups) x 4 (time points) ANOVA performed on the phonological fluency task revealed a medium sized group effect, F(2, 145) = 13.193, p <.001, = .154, a large time effect, F(3, 435) = 173.484, p <.001,
= .545, but no time x group interaction, F(6, 435) = 0.692, p = .656,
= .009. Subsequent testing of the group effect revealed that the HMA group had significantly higher scores than the AMA group (d = 0.86). The scores of the AMA and LMA groups were equal (p = .900).
Color naming
A 3 (groups) x 4 (time points) ANOVA showed a small group effect, F(2, 142) 5.440, p = .005, = .071, a large time effect, F(3, 426) = 113.785, p <.001,
= .445, but no time x group interaction, F(6, 426) = 1.956, p = .071,
= .027. Post hoc testing showed that the group effect was due to faster performance of the HMA group compared to the LMA group d = 0.80). The AMA group performed equally to the LMA group (p = .114) and HMA group (p = .165).
Mental rotation
A 3 (groups) x 2 (time points 3 and 4) ANOVA showed a medium sized group effect, F(2, 153) = 13.649, p <.001, = .151, a large time effect F(1, 153) = 55.540, p <.001,
= .266, but no time x group interaction, F(2, 153) = 0.151, p = .860,
= .002. The AMA group outperformed the LMA group (d = 0.94), while the AMA group and HMA groups did not differ significantly (p = .161).
Word decoding
A 3 (groups) x 2 (time points 3 and 4) ANOVA displayed a medium sized group effect, F(2, 146) = 8.405, p <.001, = .103, a large time effect F(1, 146) = 142.278, p <.001,
= .494, but no time x group interaction, F(2, 146) = 2.729, p = .069,
= .036. The HMA group outperformed the AMA group (d = 0.67), while the AMA group and LMA groups did not differ significantly (p = .500).
Reading comprehension
The 3 (groups) x 2 (time points 3 and 4) ANOVA displayed a medium sized group effect, F(2, 149) = 19.866, p <.001, = .211, a large time effect F(1, 149) = 180.701, p <.001,
= .548, but no time x group interaction, F(2, 149) = 2.236, p = .110,
= .029. The HMA group outperformed the AMA group (d = 0.84), and the AMA group outperformed the LMA group (d = 0.60).
Predictors of LMA and HMA in grade 6
The above ANOVAs reveal that the LMA and HMA groups display inferior and superior performance, respectively, on a multitude of tasks. In order to identify the primary predictors of LMA group membership and HMA group membership, multiple binary logistic regression analyses were performed using the pooled mean scores calculated over the different measure points.
Predictors of LMA
The tasks on which the LMA children display inferior performance compared to the AMA children were selected and used in the regression models. As many of the tasks taxed the same abilities, three separate logistic regression models were computed for the arithmetic tasks, the number tasks, and the general cognitive tasks, to identify the strongest predictors within each domain.
The binary logistic regression model computed with the four arithmetic tasks as predictors, turned out to be statistically significant, χ2(2) = 22.644, p <.001, Nagelkerke R2 = .269, Cox and Snell R2 = .179. Verbal arithmetic, B = −0.212, SE = .081, Wald = 6.902, p = .009, and multi-digit calculation, B = −0.350, SE = .179, Wald = 3.840, p = .050, emerged as significant predictors of LMA group membership. The model computed with the three number processing tasks, was also significant, χ2(2) = 20.059, p<.001, Nagelkerke R2 = .241, Cox and Snell R2 = .159. Number line estimation, B = 0.166, SE = .056, Wald = 8.819, p = .003, and digit comparison, B = 0.101, SE = .042, Wald = 5.116, p = .017, were significant predictors. The regression model, χ2(2) = 31.369, p <.001, Nagelkerke R2 = .365, Cox and Snell R2 = .241, computed with the general cognitive tasks, showed that the matrix reasoning task, B = −0.521, SE = .142, Wald = 8.844, p = .003, and the mental rotation task, B = −0.364, SE = .103, Wald = 12.485, p <.001, were significant predictors but not reading comprehension (p = .864). A final logistic regression model including the six significant predictors obtained from the three models reported above was computed. This model was statistically significant, χ2(3) = 33.851, p <.001, Nagelkerke R2 = .384, Cox and Snell R2 = .255. Verbal arithmetic, B = −0.172, SE = .087, Wald = 3.944, p = .047, matrix reasoning, B = −0.293, SE = .147, Wald = 3.951, p = .047, and mental rotation, B = −0.305, SE = .102, Wald = 8.948, p = .003, emerged as significant predictors of LMA group membership.
Predictors of HMA
Similar to the regression analyses performed to identify predictors of the LMA group separate regression models were firstly computed for each domain. The model computed with the arithmetic tasks was significant, χ2(1) = 52.023, p <.001, Nagelkerke R2 = .465, Cox and Snell R2 = .332, with verbal arithmetic, B = 0.460, SE = .086, Wald = 28.887, p <.001, as the only significant predictor. The regression model including the three number processing tasks was significant, χ2(1) = 27.120, p <.001, Nagelkerke R2 = .266, Cox and Snell R2 = .190, and HMA group membership was significantly predicted by the number line estimation task, B = −0.349, SE = .081, Wald = 18.371, p <.001. Of the five general cognitive tasks, the regression model, χ2(2) = 46.669, p<.001, Nagelkerke R2 = .425, Cox and Snell R2 = .304, showed that matrix reasoning, B = 0.360, SE = .080, Wald = 20.298, p = .002, and phonological fluency, B = 0.198, SE = .063, Wald = 9.981, p = .002, predicted HMA group membership. The final logistic regression model including the four significant predictors obtained from the three separate models was statistically significant, χ2(3) = 64.837, p <.001, Nagelkerke R2 = .554, Cox and Snell R2 = .395. Verbal arithmetic, B = 0.349, SE = .092, Wald = 14.260, p <.001, matrix reasoning, B = 0.228, SE = .090, Wald = 6.417, p = .011, and phonological fluency, B = 0.149, SE = .072, Wald = 4.320, p = .038, predicted HMA group membership.
Discussion
The present study was theoretically founded on two assumptions concerning mathematical development in children. Firstly, children’s mathematical development follows a hierarchical progression. Secondly, both domain-specific number skills and math abilities as well as domain general cognitive abilities underlie children’s development of different hierarchical levels of mathematics. Based on these assumptions, this study explored from a longitudinal retrospective perspective what characterizes 6th graders with LMA and HMA relative to children with AMA concerning the development of early domain-specific number and arithmetic skills, and general cognitive abilities. The study also explored what skills and abilities that constitute the primary predictors of LMA group membership and HMA group membership. The results are now discussed in relation to the main research questions.
Predictors and Skill development of children with LMA
Arithmetic
Consistent with prior studies, the 6th graders with LMA displayed inferior skills compared to the children with AMA on all four arithmetic tasks (nonverbal arithmetic; verbal arithmetic; addition fluency; multi-digit calculation (cf. Geary et al., Citation2009, Citation2012; Murphy et al., Citation2007). However, the LMA children developed at similar rates as the AMA children on all four arithmetic tasks.
An important finding was that the LMA children performed poorly on the basic non-symbolic arithmetic task in kindergarten. This task was assumed to impose low demands on the child’s symbolic number system knowledge (number words, digits) and comprehension of the syntactic structure of the task. Despite this, the LMA children’s scores were considerably below the AMA children’s scores (d = 0.74) suggesting that these 6th graders already prior to formal schooling had a weak understanding of the concept of arithmetic even on a basic level. This interpretation is consistent with their even worse performance on the verbal (symbolic) arithmetic task (d = 1.04) attained from kindergarten to second grade.
Given these initial arithmetic difficulties, it is reasonable, in view of a hierarchical view of mathematical development, that the LMA children also performed poorly on the addition fluency task (d = 0.76) and the written multi-digit calculation (d = 0.88) task during measurement points 3–4 (grade 2 and 3). Thus, their arithmetic difficulties continued during the three years of formal schooling (i.e., grades 1–3) when confronted with more complex multi-digit arithmetic problems or are required to solve simple single-digit addition problems by retrieving the answers quickly and automatically from long-term memory. Consistent with these findings, verbal arithmetic emerged as predictor of LMA group membership in 6th grade suggesting that weaknesses in early arithmetic impedes the acquisition of complex mathematical skills and knowledge.
Number processing skills
In addition to weak basic arithmetic skills, the 6th graders with LMA showed weaknesses in all three basic number processing skills examined from kindergarten to third grade. Already in kindergarten they exhibited poor counting skills compared to the AMA children (d = 0.65), which is in line with findings reported by Murphy et al. (Citation2007). This weakness should have been a major obstacle as counting skill is a key factor during early stages of arithmetic learning, as children use various counting strategies (finger counting; verbal counting) to solve simple arithmetic problems (Geary & Hoard, Citation2005; Siegler & Shrager, Citation1984).
The LMA children also displayed an initial, but transient, weakness in their understanding of the formal linear base-10 number system as they were inferior to the AMA children when performing the number line estimation task in kindergarten (d = 0.71), but not in the first and second grade. These findings are consistent with prior longitudinal findings, demonstrating that the LMA children suffer from an initial developmental delay in their conception of the symbolic number system, vital to learning complex mathematical skills (Geary et al., Citation2012, Citation2008; Cowan & Powell, Citation2014).
An important and novel finding was that the LMA children performed poorly (slower) on the digit comparison task during all four years (d = 0.62), from kindergarten to grade 3, but displayed similar rate of development as the AMA children. Thus, contrary to prior studies, 6th graders with LMA seem to have had problems to quickly access the underlying semantic meaning of digits consistently during the first three years of formal schooling (Cowan & Powell, Citation2014; Desoete et al., Citation2012; De Smedt & Gilmore, Citation2011; Stock et al., Citation2010).
Although the LMA group displayed poor basic number processing skills, neither of the tasks emerged as predictors of LMA group membership. That is, weaknesses in early basic number processing skills appear not to be direct key contributing factors of low mathematical skills in grade 6.
General cognitive abilities
In accordance with prior studies, the LMA group displayed consistently lower logical reasoning scores (i.e., WISC-IV Matrices) than the AMA group (d = 0.93) from kindergarten to grade 2 (cf. CitationGeary et al., Citation2008, 2009, Citation2012; Cowan & Powell, Citation2014; Murphy et al., Citation2007). Consistent with their below average logical reasoning scores, the LMA group also displayed lower reading comprehension skills compared to the AMA group in grade 2 and 3 (d = 0.60), while having average word decoding ability (Geary et al., Citation2012, Citation2008). Furthermore, the LMA children demonstrated average verbal working memory capacity (cf. Geary et al., Citation2009, Citation2007), executive functions and RAN performance (cf. Geary et al., Citation2012, Citation2008; Murphy et al., Citation2007), during all four measurement points. Another domain-general cognitive characteristic of children with LMA observed in the present study is weak spatial processing ability (cf. Murphy et al., Citation2007; see also Skagerlund & Träff, Citation2014 for similar findings in dyscalculia). That is, the LMA group was outperformed by the AMA group (d = 0.94) on the mental rotation task implemented in second and third grade.
In addition, the binary logistic regression analysis revealed that logical reasoning ability and spatial processing ability, but not reading comprehension, predicted LMA group membership. The importance of logical reasoning ability is consistent with Geary’s (Citation2013) notion that intelligence facilitates learning new abstract concepts, and processing of information, such as systematic relations among numerals. Thus, it is likely that having lower logical reasoning in kindergarten hampers the subsequent acquisition of complex mathematical skills. Weak spatial processing ability hamper LMA children’s capacity to acquire and succeed in later mathematical domains involving complex, novel, or ill-defined problems or transformation operations (Andersson & Lyxell, Citation2007; Geary, Citation1993; Kaufmann, Citation1990; Mix & Cheng, Citation2012; Van Garderen, Citation2006)
In conclusion, the present 6th graders with LMA exhibited, as expected, a multi-weakness cognitive profile that was present already prior to formal schooling, at age 6. Their weaknesses in arithmetic and number processing and domain general abilities did not get worse or better during the early years of formal schooling (i.e., grade 1–3).
As LMA group membership was predicted by verbal arithmetic skill, logical reasoning ability and spatial processing ability, and that the LMA group continuously lagged behind their average performing peers on these abilities, it likely that this combination of persistent weaknesses contributes to their low mathematical skills in grade 6.
Predictors and skill development of children with HMA
Arithmetic
The 6th graders with HMA demonstrated a superior understanding of the concept of arithmetic during all four measure points (cf. Geary et al., Citation2009). They outperformed the AMA children on nonverbal arithmetic in kindergarten (d = 0.68), and verbal arithmetic from kindergarten to grade 2 (d = 1.52). As there were no group differences in performance development for the verbal arithmetic task, it seems that the HMA children already in kindergarten have developed and acquired superior arithmetic skills compared to the AMA children. These superior kindergarten arithmetic skills may have facilitated their development of addition fluency (d = 0.74), and multi-digit calculation (d = 1.09) skills examined in grade 2 and 3.
Similar to the LMA group, verbal arithmetic turned out as a significant predictor of HMA group membership. In line with the hierarchical view of mathematical development in children, mastering of this basic arithmetic skill should function as a highly efficient stepping stone for acquiring more complex mathematical skills encountered in secondary school (i.e., grades 4-to-6; Cirino et al., Citation2016; Namkung & Fuchs, Citation2016; Träff et al., Citation2018).
Number processing
The HMA children’s performance on the three number processing tasks were mixed. Contrary to Geary et al. (Citation2009), the HMA children displayed excellent counting d = 0.86) and number line estimation performance in kindergarten (d = 0.64) relative to the AMA children. In keeping with Mazzocco et al. (Citation2011), the HMA group performed equal to the AMA group on the digit comparison tasks. Thus, 6th graders with HMA do not appear to have had a superior ability to access the underlying semantic meaning of digits in kindergarten or during the first three years of formal schooling. However, neither of the number processing tasks emerged as significant predictors of HMA group membership, indicating that superior basic number processing skills do not underlie the development of the excellent mathematical skills and knowledge that the HMA group possess.
General cognitive abilities
The HMA group outperformed the AMA group on five cognitive tasks. Similar to Heine et al. (Citation2010) and Geary et al. (Citation2009), the HMA children displayed superior logical reasoning (d = 1.19) from kindergarten to grade 2. They also exhibited above average verbal working memory capacity (d = 0.59), and superior (d = 0.86) executive functions (i.e., phonological fluency) from kindergarten to grade 3. Furthermore, the 6th graders with HMA showed that they, in grade 2 and 3, possessed superior skills in word decoding (d = 0.67), and reading comprehensions (d = 1.84). In contrast, the HMA group demonstrated average RAN performance during all four measurement points (cf. Heine et al., Citation2010), and their spatial processing abilities (i.e., mental rotation) in grade 2 and 3 were also average.
Out of the five superior cognitive abilities, only logical reasoning (matrix reasoning), and executive functions (phonological fluency) predicted HMA group membership. The combination of superior logical reasoning ability and executive functions may act as an efficient general learning mechanism facilitating the development of excellent complex mathematical skills (Geary, Citation2013; Morsanyi & Szücs, Citation2015; Träff et al., Citation2018).
In conclusion, the present results show that the HMA 6th graders possessed several cognitive strengths, vital for learning mathematics, already prior to formal schooling. Furthermore, their growth rates followed the same developmental pattern as the AMA children consistently during the first three years of formal schooling. Their early multi-strength cognitive profile and the results of the logistic regression analysis indicate that in order to develop excellent future mathematical skills, it may not be enough to merely possess exceptional early arithmetic skills. It also appears to be important that the child possess and retains, prior to formal schooling, superior general cognitive abilities (cf. Morsanyi & Szücs, Citation2015).
Differences and similarities between the LMA group and the HMA group
The LMA and HMA groups have qualitatively similar developmental profiles regarding the arithmetic skills as the LMA children and the HMA children had inferior and superior skills on all four arithmetic tasks, respectively. Furthermore, verbal arithmetic emerged as a predictor of both LMA and HMA group membership. Thus, consistent with the assumption of a hierarchical progression of mathematical learning, basic arithmetic skills are critical for the development of both excellent and weak future advanced mathematical skills.
The profiles for the number processing skills were mostly qualitatively similar. The LMA children had inferior skills on all three number processing tasks, while the HMA had superior skills on two tasks (counting; number line estimation). Another similarity was that neither group membership (LMA; HMA) was predicted by the number processing tasks. In contrast, qualitative dissimilarities were observed concerning the general cognitive tasks. The HMA group demonstrated excellence in relation to five out of seven cognitive abilities (logical reasoning, verbal working memory, executive functions, word decoding, reading comprehension). Inferior reading comprehension, spatial processing and logical reasoning were the distinguishing general cognitive characteristics of the LMA group. The logistic regression analyses showed both group similarities and differences as logical reasoning and spatial processing ability predicted LMA membership, while logical reasoning and executive functions predicted HMA membership.
In conclusion, there are both qualitative similarities and differences between the profiles of the LMA and HMA groups. An important similarity is that they, as well as the AMA group, displayed similar rates of development on all tasks (except number line estimation). The profiles for the arithmetic and number processing skills were mostly qualitatively similar, while the profiles for the general cognitive skills were mostly qualitatively dissimilar. Furthermore, the predictor patterns of the LMA and HMA groups were mostly qualitatively similar. Verbal arithmetic, logical reasoning, and spatial processing constitute unique potential risk factors for low future mathematical skills, while verbal arithmetic, logical reasoning, and executive functions are critical for developing excellent future mathematical skills.
General conclusion and educational implications
The present study extends previous findings by showing that LMA and HMA 6th graders (twelve-year-olds) already prior to and during the early years of formal schooling are characterized by multi-weakness and multi-strengths proficiency profiles involving both domain specific and domain general cognitive abilities. The cognitive profiles of the two groups, and their development, were mostly qualitatively similar, but there are also important qualitative differences. Furthermore, the predictor patterns of the LMA and HMA groups were mostly qualitatively similar.
The present findings have at least three educational implications. First, it is possible to detect children in risk of developing low mathematical skills in later school years (i.e., middle school) even before school start. The screening of at-risk children should include measures of basic arithmetic and number skills, but also logical reasoning. Second, interventions aiming at preventing and remediating low mathematical achievement during the early years of schooling should focus on basic arithmetic (i.e., verbal arithmetic), number skills but also general cognitive abilities such as logical reasoning and spatial processing.
Third, interventions aiming at promoting the development of excellent (high) mathematical achievement during the early years of schooling should focus on arithmetic skills (i.e., verbal arithmetic), but also general cognitive abilities such as logical reasoning and executive functions.
Ethics
This research project has been approved by the regional ethics committee in Linköping, Sweden (Protocol number 33–09).
Disclosure Statement
No potential conflict of interest was reported by the author(s).
Data Availability Statement
The main and corresponding author has full access to all the data in the study, and takes responsibility for the integrity of the data and the accuracy of the data analysis.
Additional information
Funding
References
- Andersson, U. (2008). Mathematical competencies in children with different types of learning difficulties. Journal of Educational Psychology, 100(1), 48–66. https://doi.org/10.1037/0022-0663.100.1.48
- Andersson, U. (2010). Skill development in different components of arithmetic and basic cognitive functions: Findings from a three-year longitudinal study of children with different types of learning difficulties. Journal of Educational Psychology, 102(1), 115–134. https://doi.org/10.1037/a0016838
- Andersson, U., & Lyxell, B. (2007). Working memory deficits in children with mathematical difficulties: A general or specific deficit? Journal of Experimental Child Psychology, 96(3), 197–228. https://doi.org/10.1016/j.jecp.2006.10.001
- Andersson, U., & Östergren, R. (2012). Number magnitude processing and basic cognitive functions in children with mathematical learning disabilities. Learning and Individual Differences, 22(6), 701–714. https://doi.org/10.1016/j.lindif.2012.05.004
- Baddeley, A. D. (1990). Human memory: Theory and practice. Lawrence Erlbaum.
- Bottge, B. A. (2001). Reconceptualizing mathematics problem solving for low-achieving students. Rase: Remedial & Special Education, 22(2), 102–112. https://doi.org/10.1177/074193250102200204
- Butterworth, B. (2010). Foundational numerical capacities and the origins of dyscalculia. Trends in Cognitive Sciences, 14(12), 534–541. https://doi.org/10.1016/j.tics.2010.09.007
- Butterworth, B., Varma, S., & Laurillard, D. (2011). Dyscalculia: From brain to education. Science, 332(6033), 1049–1053. https://doi.org/10.1126/science.1201536
- Cirino, P. T. (2011). The interrelationships of mathematical precursors in kindergarten. Journal of Experimental Child Psychology, 108(4), 713–733. https://doi.org/10.1016/j.jecp.2010.11.004
- Cirino, P. T., Tolar, T. D., Fuchs, L. S., & Huston-Warren, E. (2016). Cognitive and numerosity predictors of mathematical skills in middle school. Journal of Experimental Child Psychology, 145, 95–119. https://doi.org/10.1016/j.jecp.2015.12.010
- Cohen, J. (1988). Statistical power analysis for the behavioral sciences. Lawrence Erlbaum Associates.
- Cowan, R., & Powell, D. (2014). The contributions of domain general and numerical factors to third-grade arithmetic skills and mathematical learning disability. Journal of Educational Psychology, 106(1), 214–229. https://doi.org/10.1037/a0034097
- De Smedt, B., & Gilmore, C. K. (2011). Defective number module or impaired access? Numerical magnitude processing in first graders with mathematical difficulties. Journal of Experimental Child Psychology, 108(2), 278–292. https://doi.org/10.1016/j.jecp.2010.09.003
- Desoete, A., Ceulemans, A., De Weerdt, F., & Pieters, S. (2012). Can we predict mathematical learning disabilities from symbolic and non-symbolic comparison tasks in kindergarten? Findings from a longitudinal study. British Journal of Educational Psychology, 82(1), 64–81. https://doi.org/10.1348/2044-8279.002002
- Dowker, A. (2005). Individual differences in arithmetic: Implications for psychology, neuroscience and education. Psychology Press.
- Duncan, G. J., Dowsett, C. J., Claessens, A., Magnuson, K., Huston, A. C., Klebanov, P., Pagani, L. S., Feinstein, L., Engel, M., Brooks-Gunn, J., Sexton, H., Duckworth, K., & Japel, C. (2007). School readiness and later achievement. Developmental Psychology, 43(6), 1428–1446. https://doi.org/10.1037/0012-1649.43.6.1428
- Elofsson, J., Gustafson, S., Samuelsson, J., & Träff, U. (2016). Playing number board games supports 5-year-old children’s early mathematical development. Journal of Mathematical Behavior, 43, 134–147. https://doi.org/10.1016/j.jmathb.2016.07.003
- Elwér, Å., Fridolfsson, I., Samuelsson, S., & Wiklund, C. (2009). LäSt –Handledning – Test i läsning- och stavning för åk 1 – 5. VasaTryck AB.
- Fuchs, L. S., Geary, D. C., Compton, D. L., Fuchs, D., Hamlett, C. L., Seethaler, P. M., Bryant, J. D., & Schatschneider, C. (2010). Do different types of school mathematics development depend on different constellations of numerical versus general cognitive abilities? Developmental Psychology, 46(6), 1731–1746. https://doi.org/10.1037/a0020662
- Geary, D. C. (1993). Mathematical disabilities: Cognitive, neuropsychological, and genetic components. Psychological Bulletin, 114(2), 345–362. https://doi.org/10.1037/0033-2909.114.2.345
- Geary, D. C. (2004). Mathematics and learning disabilities. Journal of Learning Disabilities, 37(1), 4–15. https://doi.org/10.1177/00222194040370010201
- Geary, D. C., & Hoard, M. (2005). Learning disabilities in arithmetic and mathematics: Theoretical and empirical perspectives. In J. I. D. Campbell (Ed.), Handbook of mathematical cognition (pp. 253–267). Psychology Press.
- Geary, D. C. (2013). Early foundations for mathematics learning and their relations to learning disabilities. Current Directions in Psychological Science, 22(1), 23–27. https://doi.org/10.1177/0963721412469398
- Geary, D. C., Bailey, D. H., Littlefield, A., Wood, P., Hoard, M. K., & Nugent, L. (2009). First-grade predictors of mathematical learning disability: A latent class trajectory analysis. Cognitive Development, 24(4), 411–429. https://doi.org/10.1016/j.cogdev.2009.10.001
- Geary, D. C., Hoard, M. K., Byrd-Craven, J., Nugent, L., & Numtee, C. (2007). Cognitive mechanisms underlying achievement deficits in children with mathematical learning disability. Child Development, 78(4), 1343–1359. https://doi.org/10.1111/cdev.2007.78.issue-4
- Geary, D. C., Hoard, M. K., Nugent, L., & Bailey, D. H. (2012). Mathematical cognition deficits in children with learning disabilities and persistent low achievement: A five-year prospective study. Journal of Educational Psychology, 104(1), 206–223. https://doi.org/10.1037/a0025398
- Geary, D. C., Hoard, M. K., Nugent, L., & Byrd-Craven, J. (2008). Development of number line representations in children with mathematical learning disability. Developmental Neuropsychology, 33(3), 277–299. https://doi.org/10.1080/87565640801982361
- Hanich, L. B., Jordan, N. C., Kaplan, D., & Dick, J. (2001). Performance across different areas of mathematical cognition in children with learning difficulties. Journal of Educational Psychology, 93(3), 615–626. https://doi.org/10.1037/0022-0663.93.3.615
- Heine, A., Tamm, S., De Smedt, B., Schneider, M., Thaler, V., Torbeyns, J., Elsbeth., S., Verschaffel, L., & Jacobs, A. (2010). The numerical stroop effect in primary school children: A comparison of low, normal, and high achievers. Child Neuropsychology, 16(5), 461–477. https://doi.org/10.1080/09297041003689780
- Jordan, N. C., & Hanich, L. B. (2003). Characteristics of children with moderate mathematics deficiencies: A longitudinal perspective. Learning Disabilities Research & Practice, 18(4), 213–221. https://doi.org/10.1111/ldrp.2003.18.issue-4
- Jordan, N. C., Hanich, L. B., & Kaplan, D. (2003). A longitudinal study of mathematical competencies of children with specific mathematics difficulties versus children with comorbid mathematics and reading difficulties. Child Development, 74(3), 834–850. https://doi.org/10.1111/cdev.2003.74.issue-3
- Jordan, N. C., Kaplan, D., Nabors Ola´h, L., & Locuniak, M. (2006). Number sense growth in kindergarten: A longitudinal investigation of children at risk for mathematics difficulties. Child Development, 77(1), 153–175. https://doi.org/10.1111/cdev.2006.77.issue-1
- Kaufmann, G. (1990). Imagery effects on problem solving. In P. J. Hampson, D. E. Marks, & J. T. E. Richardson (Eds.), Imagery: Current developments (pp. 169–197). Routledge.
- Kucian, K., & von Aster, M. (2015). Developmental dyscalculia. European Journal of Pediatrics, 174(1), 1–13. https://doi.org/10.1007/s00431-014-2455-7
- Landerl, K., Bevan, A., & Butterworth, B. (2004). Developmental dyscalculia and basic numerical capacities: A study of 8–9-year-old students. Cognition, 93(2), 99–125. https://doi.org/10.1016/j.cognition.2003.11.004
- LeFevre, J., Fast, L., Skwarchuk, S., Smith-Chant, B., Bisanz, J., Kamawar, D., & Penner-Wilger, M. (2010). Pathways to mathematics: Longitudinal predictors of performance. Child Development, 81(6), 1753–1767. https://doi.org/10.1111/cdev.2010.81.issue-6
- Malmquist, E. (1977). Reading and writing difficulties in children. Analysis and treatment. Gleerups.
- Mammarella, I. C., Caviola, S., Giofrè, D., & Szücs, D. (2018). The underlying structure of visuospatial working memory in children with mathematical learning disability. British Journal of Developmental Psychology, 36(2), 220–235. https://doi.org/10.1111/bjdp.2018.36.issue-2
- Mazzocco, M. M. M., Feigenson, L., & Halberda, J. (2011). Impaired acuity of the approximate number system underlies mathematical learning disability (Dyscalculia). Child Development, 82(4), 1224–1237. https://doi.org/10.1111/cdev.2011.82.issue-4
- McCloskey, M. (2007). Quantitative literacy and developmental dyscalculias. In D. B. Berch & M. M. M. Mazzocco (Eds.), Why is math so hard for some children? The nature and origins of mathematical learning difficulties and disabilities (pp. 415–429). Paul H Brookes Publishing.
- McKenzie, B., Bull, R., & Gray, C. (2003). The effects of phonological and visual–spatial interference on children’s arithmetical performance. Educational and Child Psychology, 20(3), 93–108.
- Mix, K. S., & Cheng, Y.-L. (2012). The relation between space and math: Developmental and educational implications. In J. B. Benson (Ed.), Advances in child development and behavior (Vol. 42, pp. 197–243). Academic Press.
- Morsanyi, K., & Szücs, D. (2015). The link between mathematics and logical reasoning. In S. Chinn (Ed.), The Routledge international handbook of Dyscalcylia and mathematical learning difficulties (pp. 101–114). Routledge.
- Murphy, M. M., Mazzocco, M. M. M., Hanich, L. B., & Early, M. C. (2007). Cognitive characteristics of children with mathematics learning disability (MLD) vary as a function of the cutoff criterion used to define MLD. Journal of Learning Disabilities, 40(5), 458–478. https://doi.org/10.1177/00222194070400050901
- Namkung, J. M., & Fuchs, L. S. (2016). Cognitive predictors of calculations and number line estimation with whole numbers and fractions among at-risk students. Journal of Educational Psychology, 108(2), 214–228. https://doi.org/10.1037/edu0000055
- Olsson, L., Östergren, R., & Träff, U. (2016). Developmental dyscalculia: A deficit in the approximate number system or an access deficit? Cognitive Development, 39, 154–167. https://doi.org/10.1016/j.cogdev.2016.04.006
- Ostad, S. A. (1998). Developmental differences in solving simple arithmetic word problems and simple number-fact problems: A comparison of mathematically normal and mathematically disabled children. Mathematical Cognition, 4(1), 1–19. https://doi.org/10.1080/135467998387389
- Passolunghi, M. C., & Lanfranchi, S. (2012). Domain-specific and domain-general precursors of mathematical achievement: A longitudinal study from kindergarten to first grade. British Journal of Educational Psychology, 82(1), 42–63. https://doi.org/10.1111/bjep.2012.82.issue-1
- Passolunghi, M. C., & Siegel, L. S. (2001). Short-term memory, working memory, and inhibitory control in children with difficulties in arithmetic problem solving. Journal of Experimental Child Psychology, 80(1), 44–57. https://doi.org/10.1006/jecp.2000.2626
- Passolunghi, M. C., & Siegel, L. S. (2004). Working memory and access to numerical information in children with disability in mathematics. Journal of Experimental Child Psychology, 88(4), 348–367. https://doi.org/10.1016/j.jecp.2004.04.002
- Piazza, M., Facoetti, A., Trussardi, A. N., Berteletti, I., Conte, S., Lucangeli, D., Dehaene, S., & Zorzi, M. (2010). Developmental trajectory of number acuity reveals a severe impairment in developmental dyscalculia. Cognition, 116(1), 33–41. https://doi.org/10.1016/j.cognition.2010.03.012
- Raghubar, K. P., Barnes, M. A., & Hecht, S. A. (2010). Working memory and mathematics: A review of developmental, individual difference, and cognitive approaches. Learning and Individual Differences, 20(2), 110–122. https://doi.org/10.1016/j.lindif.2009.10.005
- Rasmussen, C., & Bisanz, J. (2005). Representation and working memory in early arithmetic. Journal of Experimental Child Psychology, 91(2), 137–157. https://doi.org/10.1016/j.jecp.2005.01.004
- Roth, B., Becker, N., Romeyke, S., Schäfer, S., Domnick, F., & Spinath, F. M. (2015). Intelligence and school grades. A Meta-analysis. Intelligence, 53, 118–137. https://doi.org/10.1016/j.intell.2015.09.002
- Rousselle, L., & Noël, M. P. (2007). Basic numerical skills in children with mathematics learning disabilities: A comparison of symbolic vs non-symbolic number magnitude processing. Cognition, 102(3), 361–395. https://doi.org/10.1016/j.cognition.2006.01.005
- Rüsseler, J., Scholz, J., Jordan, K., & Quasier-Pohl, C. (2005). Mental rotation of letters, pictures, and three-dimensional objects in german dyslexic children. Child Neuropsychology, 11(6), 497–512. https://doi.org/10.1080/09297040490920168
- Seethaler, P. M., Fuchs, L. S., Star, J. R., & Bryant, J. (2011). The cognitive predictors of computational skill with whole versus rational numbers: An exploratory study. Learning and Individual Differences, 21(5), 536–542. https://doi.org/10.1016/j.lindif.2011.05.002
- Siegler, R. S., & Shrager, J. (1984). Strategy choice in addition and subtraction: How do children know what to do? In C. Sophian (Ed.), Origins of cognitive skills (pp. 229–293). Hillsdale, NJ: Erlbaum.
- Skagerlund, K., & Träff, U. (2014). Development of magnitude processing in children with developmental dyscalculia: Space, time, and number. Frontiers in Psychology, 5, 675. https://doi.org/10.3389/fpsyg.2014.00675
- Skagerlund, K., & Träff, U. (2016). Number processing and heterogeneity of developmental dyscalculia: Subtypes with different cognitive profiles and deficits. Journal of Learning Disabilities, 49(1), 36–50. https://doi.org/10.1177/0022219414522707
- Stock, P., Desoete, A., & Roeyers, H. (2010). Detecting children with arithmetic disabilities from kindergarten: Evidence from a 3-year longitudinal study on the role of preparatory arithmetic abilities. Journal of Learning Disabilities, 43(3), 250–268. https://doi.org/10.1177/0022219409345011
- Szücs, D., Devine, A., Soltesz, F., Nobes, A., & Gabriel, F. (2013). Developmental dyscalculia is related to visuo-spatial memory and inhibition impairment. Cortex, 49(10), 2674–2688. https://doi.org/10.1016/j.cortex.2013.06.007
- Träff, U. (2013). The contribution of general cognitive abilities and number abilities to different aspects of mathematics in children. Journal of Experimental Child Psychology, 116(2), 139–156. https://doi.org/10.1016/j.jecp.2013.04.007
- Träff, U., Olsson, L., Östergren, R., & Skagerlund, K. (2017). Heterogeneity of developmental dyscalculia: Cases with different deficit profiles. Frontiers in Psychology, 7, 2000. https://doi.org/10.3389/fpsyg.2016.02000
- Träff, U., Olsson, L., Skagerlund, K., & Östergren, R. (2018). Cognitive mechanisms underlying third graders’ arithmetic skills: Expanding the pathways to mathematics model. Journal of Experimental Child Psychology, 167, 369–387. https://doi.org/10.1016/j.jecp.2017.11.010
- Träff, U., Olsson, L., Skagerlund, K., & Östergren, R. (2020). Kindergarten domain-specific and domain-general cognitive precursors of hierarchical mathematical development: A longitudinal study. Journal of Educational Psychology, 112(1), 93–109. https://doi.org/10.1037/edu0000369
- Träff, U., Skagerlund, K., Olsson, L., & Östergren, R. (2017). Pathways to mathematics in adolescents. British Journal of Educational Psychology, 87(4), 647–663. https://doi.org/10.1111/bjep.12170
- Van Garderen, D. (2006). Spatial visualization, visual imagery, and mathematical problem solving of students with varying abilities. Journal of Learning Disabilities, 39(6), 496–506. https://doi.org/10.1177/00222194060390060201
- Vilia, P. N., Candeias, A. A., Neto, A. S., Franco, M. S., & Melo, M. (2017). Academic achievement in physics-chemistry: The predictive effect of attitudes and reasoning abilities. Frontiers in Psychology, 8, 1064. https://doi.org/10.3389/fpsyg.2017.01064
- Von Aster, M. G., & Shalev, R. S. (2007). Number development and developmental dyscalculia. Developmental Medicine & Child Neurology, 49(11), 868–873. https://doi.org/10.1111/dmcn.2007.49.issue-11
- Vukovic, R. K., Fuchs, L. S., Geary, D. C., Jordan, N. C., Gersten, R., & Siegler, R. S. (2014). Sources of individual differences in children’s understanding of fractions. Child Development, 85(4), 1461–1478. https://doi.org/10.1111/cdev.2014.85.issue-4
- Wechsler, D. (2003). Wechsler intelligence scale for children (4th ed., WISC-IV). Harcourt Assessment.