ABSTRACT
Engaging students in active learning is linked to positive learning outcomes. This study aims to synthesise the peer-reviewed literature about ‘active learning’ in college science classroom settings. Using the methodology of an integrative literature review, 337 articles archived in the Educational Resources Information Center (ERIC) are examined. Four categories of in-class activities emerge: (i) individual non-polling activities, (ii) in-class polling activities, (iii) whole-class discussion or activities, and (iv) in-class group activities. Examining the collection of identified in-class activities through the lens of a theoretical framework informed by constructivism and social interdependence theory, we synthesise the reviewed literature to propose the active learning strategies (ALSs) model and the instructional decisions to enable active learning (IDEAL) theory. The ALS model characterises in-class activities in terms of the degrees to which they are designed to promote (i) peer interaction and (ii) social interdependence. The IDEAL theory includes the ALS model and provides a framework for conceptualising different levels of the general concept ‘active learning’ and how these levels connect to instructional decision-making about using in-class activities. The proposed ALS model and IDEAL theory can be utilised to inform instructional decision-making and future research about active learning in college science courses.
Introduction
Lecture is a method of transmitting information to college students that has been used since Western European universities were first conceived (Brockliss, Citation1996). Knowing transmitted facts and other information forms the basis for further learning, but learning involves more than the ability to recall information. Contemporary college science education goals are concerned with developing higher order thinking skills (Bloom, Citation1956; Krathwohl, Citation2002), modelling what scientists do (Handelsman et al., Citation2004), science literacy and the ability to apply scientific knowledge to decision-making (Earth Science Literacy Initiative, Citation2010; US Global Change Research Program, Citation2009), and self-efficacy in science disciplines (Trujillo & Tanner, Citation2014).
While lecture remains a time-honoured tradition in college science instruction, two features in the educational landscape changed markedly in the past ∼30 years. The first is the widespread acceptance (but not necessarily adoption) of learning theories that emphasise the process of actively constructing knowledge (Bransford, Brown, & Cocking, Citation1999). The second is easy information access through means other than lecture, especially via the internet (Poushter, Citation2016).
These changes challenge the value of lecture for achieving contemporary science education goals. Prolonged periods of lecture set up students for passive learning, which focuses students on information (Harlen & James, Citation1997), which is now often accessible online. Also, traditional lectures exceed average attention spans (Wankat, Citation2002), thus impairing even the short-term recall of information presented in lectures (Hartley & Davies, Citation1978).
Moving basic information transfer to outside of class time opens up in-class time to engage students in more active learning opportunities. The term ‘active learning’, however, is used throughout the literature in a variety of contexts and often is not explicitly defined, thus leading to a lack of consensus and even confusion about what constitutes ‘active learning’. According to Freeman et al. (Citation2014, pp. 4–5), ‘Active learning engages students in the process of learning through activities and/or discussion in class, as opposed to passively listening to an expert. It emphasises higher order thinking and often involves group work.’ We adopt this definition of active learning for this study. In-class activities that promote active learning are more effective than lecture alone at developing students’ higher order cognitive skills (Halpern, Citation1999), soft skills such as communication and collaboration (Bosworth, Citation1994; Zekeri, Citation2004), and self-efficacy (DiBenedetto & Bembenutty, Citation2013). Furthermore, in-class activities have greater potential to model how scientists approach doing and thinking about their science than is possible through lecture alone.
Despite persistent calls for science education reform to incorporate active learning opportunities (Handelsman & Brown, Citation2016; Olson & Riordan, Citation2012), the subject can be polarising among faculty (Prince, Citation2004). Evidence also suggests that college science courses remain dominated by traditional lecture-based instruction (National Research Council, Citation1999, Citation2003). Nevertheless, teaching-innovation adopters (Henderson, Dancy, & Niewiadomska-Bugaj, Citation2012) in science instruction have responded in practical terms to calls for instructional reform by incorporating in-class activities that promote active learning in classroom instruction.
The efforts of teaching-innovation adopters to transform college science instruction are evidenced by the peer-reviewed literature that describes the in-class activities they use to replace and/or accompany lectures and enable active learning in classroom settings. In-class activities take many forms and go by many names. The purpose of this study is to examine the peer-reviewed literature to identify, characterise, summarise, and organise in-class activities used in college science courses to enable active learning. In particular, this study answers the following research questions:
Based on the peer-reviewed literature, what in-class activities do instructors use to enable active learning in college science classroom settings?
How do college science instructors implement these in-class activities?
How do these in-class activities relate to existing theories about how people learn and what more general strategies, models, or theories emerge from the analysis?
Examination of what instructors do in online, hybrid, lab, or field settings is beyond the scope of the present study. This is not a meta-analysis of published statistical results, thus an examination of the impact that these activities have on learning gains is beyond the scope of this study.
Theoretical framework
This study’s theoretical framework makes explicit governing assumptions about how people learn and simultaneously provides the lens through which the reviewed information are organised and interpreted. Specifically, constructivism and social interdependence theory provide the basis of this theoretical framework.
Constructivism
Over the past ∼30 years, research in cognitive science, brain science, and educational psychology have collectively advanced our understanding of how people learn (Bransford et al., Citation1999). Learning happens in the mind of the learner, and it can be surficial or deep. Surface learning is characterised by an individual’s ‘intention to be able to reproduce content as required, passive acceptance of ideas and information, lack of recognition of guiding principles or patterns, [and a focus on learning to satisfy] assessment requirements’ (Harlen & James, Citation1997, p. 368). Deep learning, on the other hand, is characterised by an individual’s ‘intention to develop personal understanding; active interaction with the content, particularly in relating new ideas to previous knowledge and experience; linking ideas together using integrating principles; and relating evidence to conclusions’ (Harlen & James, Citation1997, p. 368).
Surface learning is the product of activities that demand little effort, such as listening to lecture (Freeman et al., Citation2014). Deep learning, on the other hand, arises as the result of activities that challenge the learner within their zone of proximal development (Vygotsky, Citation1934, 1986) and that activate, engage, and build on their prior knowledge and experience (Bransford et al., Citation1999). In this way, individuals construct personally meaningful knowledge. The notion that individuals construct knowledge led to the advance in the ‘constructivist’ approach to instruction. Deep learning and construction of knowledge is facilitated when individuals have the opportunity to actively engage with the subject matter as well as with other individuals.
Social interdependence theory
Although learning happens in the mind of the individual, it is influenced by and enhanced with social interactions that occur in context. Social learning theories explain how people learn from one another in socio-cultural contexts. Individuals learn through their interactions and communications with peers, instructors, and experts (Vygotsky, Citation1934, Citation1978, Citation1986), and culture is the main determining factor in constructing knowledge (Vygotsky, Citation1978). The construction of knowledge occurs within and cannot be separated from socio-cultural contexts (Vygotsky, Citation1978).
The social interdependence theory proposed by Lewin (Citation1935) states that shared goals among individuals can generate an interdependence among group members. Deutsch (Citation1949, Citation1962) extends this idea by explaining that ‘the tension systems of different people arising from their goals may be either positively or negatively interrelated’ (Johnson & Johnson, Citation2011). Johnson and Johnson (Citation2011) summarise Deutsch’s ideas in the following way:
Positive interdependence exists when there is a positive correlation among individuals’ goal attainments; individuals perceive that they can attain their goals if and only if the other individuals with whom they are cooperatively linked attain their goals. Negative interdependence exists when there is a negative correlation among individuals’ goal achievements; individuals perceive that they can obtain their goals if and only if the other individuals with whom they are competitively linked fail to obtain their goals. No interdependence exists when there is no correlation among individuals’ goal achievements; individuals perceive that the achievement of their goals is unrelated to the goal achievement of others. (p. 42)
Methodology and methods
The research questions are addressed within a pragmatist world view (Creswell, Citation2014), and the methodology is an integrative literature review (Torraco, Citation2005). An integrative literature review is a systematic review that synthesises the literature to develop new theories or models from existing theories (Torraco, Citation2005). The aim of this literature review is not to present an exhaustive list of all types of activities used to teach college science courses, but to (i) summarise such activities as they are described in the peer-reviewed literature, (ii) describe how such activities are implemented, and (iii) propose new theories or models that deepen or expand our knowledge of activities used in college science instruction to enable active learning.
This is an exploratory study that reviews peer-reviewed articles published from 1994 to 2014 and archived in the Educational Resources Information Center (ERIC) database. Peer-reviewed articles were selected because they meet accepted standards for quality. The time period 1994–2014 was selected because it is more directly relevant to current science instruction practices while allowing time for articles published in that time period to be archived in the ERIC. The ERIC database was selected because it is reputable and widely used for searching education research. This study is based on a staged review of the literature.
During the first stage, the ERIC database was searched for peer-reviewed articles using combinations of different terms () in the time period 1994–2014. This search yielded 337 articles. All abstracts were read to determine whether the article discussed college science classroom instruction. More than half of the articles were excluded from further analysis because they did not meet the inclusion criteria of college science classroom instruction. Additional articles were identified using a snowballing method (Wohlin, Citation2014). In all, the search produced 127 articles that satisfied the inclusion criteria for further analysis.
Table 1. Different combinations of the terms were used in the title, abstract, and key words in ERIC’s filterable fields to search for appropriate literature.
During the second stage, a coding manual was iteratively developed and used to analyse the 127 articles in terms of discipline, class size, in-class activities, and how activities were implemented. The authors conducted a constant comparative analysis (Charmaz, Citation2014) to identify emergent categories and subcategories of activities for the coding manual. Both authors coded all 127 articles, with >93% agreement.
Results
The literature reveals that a variety of in-class activities are implemented in college science courses to enable active learning. Four main categories emerged from the analysis of the literature: (i) individual non-polling activities, (ii) in-class polling activities, (iii) whole-class discussions or activities, and (iv) in-class group activities.
Individual non-polling activities
A popular classroom assessment technique, such as those compiled by Angelo and Cross (Citation1993), is the minute paper. Students have one minute to write a response to an open-ended question (Armbruster, Patel, Johnson, & Weiss, Citation2009; McConnell, Steer, & Owens, Citation2003), which is collected and provides feedback about student progress to the instructor.
More time-intensive activities include writing exercises to improve students’ written expression and argumentation skills (Dengler, Citation2008), prompt students to reflect on questions they have about what they are learning (Lunsford & Herzog, Citation1997), and provide peer reviews of student work (Dengler, Citation2008; Odom, Glenn, Sanner, & Cannella, Citation2009). Still other activities involve individually solving a conceptual or a quantitative problem (Gutwill-Wise, Citation2001; Klein, Citation2003), using maps (Klein, Citation2003), and organising information by drawing a concept map (Armbruster et al., Citation2009; Klein, Citation2003; McConnell et al., Citation2003).
More creative activities include building physical models using Play-Doh (Marbach-Ad, Seal, & Sokolove, Citation2001), store-purchased kits such as those used to build molecular structures (Hageman, Citation2010), or schematic images of components to an analytical instrument (Algar & Krull, Citation2010); creating films or documentaries (Anderson, Citation2013); and participating in guided discovery activities (Schultz, Citation1997).
In-class polling activities
In-class polling activities enable instructors to elicit students’ prior knowledge, confront prior knowledge that contradicts accepted scientific understandings, and resolve mental contradictions even in very large science classes (Lin, Liu, & Chu, Citation2011). In-class polling is implemented in a wide variety of ways, depending on available polling mechanisms, placement of the activity in the lesson, types of polling questions, and polling structures.
High- and low-tech polling mechanisms display polling questions and receive responses. High-tech options include using software packages, such as PowerPoint (Armbruster et al., Citation2009) and audience response systems or clickers (Nicol & Boyle, Citation2003). Low-tech options involve writing the multiple-choice question on the board (Paulson, Citation1999) or projecting it on a transparency (Kovac, Citation1999) and receiving student responses using cards with A, B, C, and D printed on them (Freeman et al., Citation2007) or hand raising (Kovac, Citation1999).
The placement of a polling activity within a lesson is highly variable. It may occur at the beginning of a lecture (Crossgrove & Curran, Citation2008; Freeman et al., Citation2007) or at any other time (Addison, Wright, & Milner, Citation2009; Crouch & Mazur, Citation2001; Freeman et al., Citation2007; Gok, Citation2012; Smith, Trujillo, & Su, Citation2011). The polling questions are multiple-choice items whose questions may be conceptual (McConnell et al., Citation2003) or factual (Crossgrove & Curran, Citation2008). Polling is also used to administer quiz questions (Donohue, Citation2014; Slish, Citation2005).
Polling structures can be more or less formalised. Formalised activities follow specified steps, such as those Mazur (Citation1999) outlines for peer instruction (PI). PI involves students individually answering a challenging conceptual multiple-choice question by voting, discussing the question with a peer, and then answering the question again. After students’ second vote for the answer, the instructor facilitates whole-class discussion about students’ reasoning (Duncan, Citation2006; Miller, Lasry, Lukoff, Schell, & Mazur, Citation2014; Perez et al., Citation2010; Turpen & Finkelstein, Citation2010). Another formalised implementation involves displaying a sequence of three questions (Reay, Bao, Li, Warnakulasooriya, & Baugh, Citation2005; Reay, Li, & Bao, Citation2008). The first question is relatively easy and serves as a warm-up question. The next two questions are more challenging. The questions are answered either individually or in groups.
Other polling activities do not follow such formalised steps. Some instructors facilitate peer discussion following independent voting but do not have students revote (Hoekstra, Citation2008). Others ask several questions in a row without peer discussion (Coca & Slisko, Citation2013; Meltzer & Manivannan, Citation2002). Instead of following a poll with discussion, some students complete an activity in groups (Irons, Citation2012; Levesque, Citation2011; Linton, Pangle, Wyatt, Powell, & Sherwood, Citation2014; Reay et al., Citation2005; Shaver, Citation2010). Such activities include, for example, writing about their answer choice or reading a related article (Bandyopadhyay, Citation2013; Flynn, Citation2011; Linton et al., Citation2014).
Whole-class discussions or activities
Whole-class discussions or activities do not necessarily take the entire class period but do involve the whole-class participating in one way or another. They are facilitated by an instructor, a student, or group of students (Middlecamp & Nickel, Citation2000; Orvis & Orvis, Citation2005). Whole-class discussions follow the same general steps for implementation: (i) students complete an activity, (ii) someone facilitates a whole-class discussion, and (iii) students contribute to the discussion by answering and/or asking questions. When whole-class activities involve different student roles, the instructor may assign roles or students may self-select their roles. Examples of whole-class activities are drawn from the work of Farrer, Monk, Heron, Lough, and Sadler (Citation2010), Orvis and Orvis (Citation2005), and Middlecamp and Nickel (Citation2000).
Farrer et al. (Citation2010) implement a two-part whole-class activity. In the first part, each student is assigned a chemical element and asked to prepare answers to questions about that element. In the second part, students use coloured ping pong balls to perform role plays of their assigned elements, which is intended to help students distinguish between elements that will donate electrons from those that will accept electrons. The last part of the role play involves students positioning themselves relative to one another’s assigned elements to form a human representation of the periodic table.
Orvis and Orvis (Citation2005) implement a whole-class activity intended to help students learn about chemical reactions. Wads of paper represent chemical reactants and chemical products. Using the wads of paper, students work through a series of questions about chemical reactions. Middlecamp and Nickel (Citation2000) use a student-generated questionnaire on the board. Students agree on the questions and multiple-choice answers. Completion of the questionnaire is followed with an analytical chemistry exercise. These three examples of whole-class activities are concluded with an instructor-led debriefing about each activity’s learning goals and key concepts.
In-class group activities
Four kinds of in-class group activities emerged from the literature review: (i) process-oriented guided inquiry learning, (ii) lecture tutorials (LTs), (iii) problem-based learning, and (iv) jigsaws.
Process-oriented guided inquiry learning
POGIL activities are chemistry-specific and can largely replace lecture (Eberlein et al., Citation2008). A brief (∼15 min) lecture precedes a POGIL activity to orient students to the learning goals and criteria for successful completion (Hanson, Citation2006). POGIL activities are multi-page worksheets that are based on ‘a learning cycle design of exploration and concept formation followed by application’ (Hanson, Citation2006, p. iii).
In the exploration phase, students are given a model to explore and find patterns (Eberlein et al., Citation2008), thus creating the basis of knowledge for the remainder of the activity (Hu & Shepherd, Citation2013). In the concept invention phase, students further identify patters and recognise relationships and construct knowledge (Eberlein et al., Citation2008). In the application phase, students extend and apply their gained knowledge to new situations (Eberlein et al., Citation2008) and problems (Hu & Shepherd, Citation2013).
POGIL activities can be completed individually but their completion in groups is recommended (Hanson, Citation2006). Groups can be determined by students (Eberlein et al., Citation2008) or the instructor (Hu & Shepherd, Citation2013). Each group has three to four members, where each member holds a role. Three roles are included in all activities: manager, recorder, and spokesperson (Bailey, Minderhout, & Loertscher, Citation2012; Hu & Shepherd, Citation2013; Simonson & Shadle, Citation2013). The instructor serves as a guide who monitors group interactions and supports students’ efforts (Bailey et al., Citation2012; Eberlein et al., Citation2008; Simonson & Shadle, Citation2013). At the end of the activity, the instructor may call the class together and debrief the activity or collect the completed activities and provide feedback in the next class meeting.
Lecture tutorials
LTs deepen students’ conceptual understandings and are usually completed in pairs (Gray & Steer, Citation2012; LoPresto & Murrell, Citation2009). These worksheets are available for astronomy, geoscience, and physics courses. Some are one or two pages long, designed to be completed in 10–20 minutes during a single pause in lecture (Brogt, Citation2007; Kortz, Smay, & Murray, Citation2008). Others are longer, designed to be completed over multiple pauses in a lecture or over series of lectures. As students work through an LT, the instructor may ask additional questions to facilitate student thinking (Koenig, Endorf, & Braun, Citation2007). According to Prather, Slater, Brissenden, Adams, and Dostal (Citation2008):
Each lecture tutorial presents a structured series of questions designed to confront and resolve student difficulties with a particular topic. Confronting difficulties often means answering questions incorrectly; this is expected. When this happens, the activities make use of additional questions or situated student debates designed to help a student understand where her or his reasoning went wrong and to develop a more thorough understanding as a result. Therefore, while completing the activities, students are encouraged to focus more on their reasoning and less on trying to guess an expected answer. These activities are meant to be completed by students working in pairs who ‘talk out’ their answers and reasoning with each other to make their thinking explicit. (p. v)
Problem-based learning
PBL activities pose an authentic real-life problem to students before new content is presented via lecture (Amador, Miles, & Peters, Citation2007). In PBL, a problem drives learning. PBL activities are generally designed to replace a lecture. Groups engage in an iterative cycle of answering three questions until they are able to solve a problem: (i) What do we know? (ii) What else do we need to know? (iii) How will we learn it? (Amador et al., Citation2007). PBL mirrors actual practices of scientists working to solve authentic problems. As such, they are a vehicle for developing valuable professional skills, learning about the nature of science, and motivating learning.
PBL activities are often instructor-designed (Keller, Citation2002). They utilise conceptual questions (Christiansen, Citation2014; Enghag, Gustafsson, & Jonsson, Citation2007; Robinson, Citation2001) and/or calculation-based questions (González-Sancho et al., Citation2013; Ramsier, Citation2001). Groups may be asked to consider information provided in case studies (Gardner & Belland, Citation2012; González-Sancho et al., Citation2013), primary literature (Eberlein et al., Citation2008), and/or textbooks (Enghag et al., Citation2007).
Groups are assigned by the instructor, determined via random assignment (Ramsier, Citation2001), or by student self-selection (Fardilha, Schrader, da Cruz e Silva, & da Cruz e Silva, Citation2010; Slish, Citation2005). The life of groups is variable; some instructors have groups disband at the end of one activity and later form new groups (Enghag et al., Citation2007; Kovac, Citation1999; Ramsier, Citation2001), whereas others set up permanent groups in which students are expected to work throughout the semester (Armbruster et al., Citation2009; Keller, Citation2002). While groups work together to solve a problem, the instructor circulates around the room to monitor groups’ progress, probe student reasoning, and/or provide additional information (Christiansen, Citation2014; Hein, Citation2012).
PBL activities are completed and submitted in different ways. For example, groups may work through a worksheet or other handout that they submit at the end of the class (Eberlein et al., Citation2008; Hein, Citation2012; Slish, Citation2005). Alternatively, groups may be given a dry erase board to record and share answers as they work through the problem (Ramsier, Citation2001). Groups can also submit their completed activities electronically (Kovac, Citation1999; Nogaj, Citation2013; Robinson, Citation2001). In some implementations of PBL, groups share their answers in class during an instructor-facilitated debriefing (Armbruster et al., Citation2009; Nogaj, Citation2013).
Jigsaws
In a jigsaw activity, the class is divided into several groups, with each group preparing separate but related assignments. When all group members are prepared, the class is re-divided into mixed teams, with one member from each of the earlier groups. Each person in the new team teaches the rest of the team what was learned in their first group, and then the team tackles a task or problem that requires pulling all of the pieces together, hence the name ‘jigsaw’. Jigsaw activities help develop a community of learning, teamwork skills, and individual learning. A jigsaw may be designed for implementation during a single class meeting or over multiple class meetings, depending on the level of sophistication of the work involved with the initial groups and the final teams. Jigsaw activities can replace a lecture.
Tewksbury (Citation1995) and Slish (Citation2005) state that jigsaw activities can be designed for students to share notes or solve problems, respectively. In sharing notes, students in the initial groups have and discuss a certain set of notes. Then, in the reconstituted teams, each member from a different initial group shares the information in their notes with their team (Slish, Citation2005). In solving problems, students in the initial groups are asked to work together to solve different problems and, then, the reconstituted teams are asked to solve a problem whose solution requires that the team members share and build on the work completed in their initial groups (Tewksbury, Citation1995). Jigsaw activities typically conclude with a whole-class instructor-facilitated debriefing to discuss any potential lingering questions students might have.
Discussion
The purpose of an integrative literature review is to identify trends, patterns, and gaps as well as to synthesise the literature to develop new models or theories (Torraco, Citation2005). These are discussed in the discussion below.
Trends
Our review reveals a positive upward trend between 1994 and 2014 in the number of peer-reviewed articles about in-class activities in college science instruction (). Furthermore, when disaggregated by disciplines, an upward trend is also evidenced in each of the four dominant disciplines (biology, chemistry, Earth and space science, and physics) represented in the corpus of literature reviewed. This study also reveals that in-class activities are implemented in a wide range of class sizes, from relatively small classes (<25 students) to very large classes (>300 students).
Patterns
Four patterns or categories of in-class activities emerged from the literature: (i) individual non-polling activities, (ii) in-class polling activities, (iii) whole-class discussion or activities, and (iv) in-class group activities. Within each category, exist different kinds of specific activities (see Results section). In the present study, an active learning activity is defined as a specific process or action(s) intended to enable active learning in the classroom setting. An activity focuses on specific logistical details for implementation.
Using descriptions of peer interactions in the literature and the study’s theoretical framework, we characterise in-class activities in terms of the type of peer interactions they require and in terms of whether they foster positive interdependence or not ((a)). Loose peer interactions are short-lived and often do not involve the same peers, whereas tight peer interactions involve permanent group membership that is sustained throughout the semester. (a) illustrates different patterns of activities. For example, those with no peer interaction and no social interdependence plot in the lower left-hand corner of the graph.
Figure 2. The active learning strategies (ALSs) model connects in-class activities with active learning strategies (see text for explanation of activity versus strategy). (a) Different kinds of in-class activities are characterised according to the extent to which they use peer interaction and social interdependence to facilitate active learning. The kinds of activities are superimposed above the categories to which they belong. Note: ‘CATs’ are classroom assessment techniques and ‘PI’ is peer instruction. (b) Four types of active learning strategies emerge from the characterisation of in-class activities.
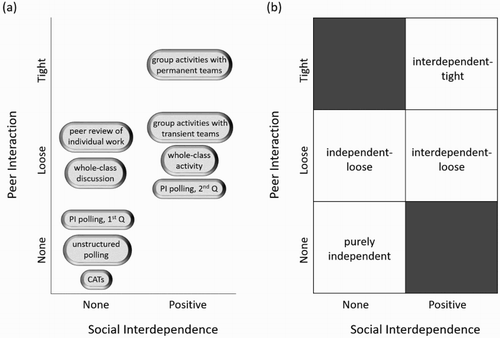
Juxtaposed adjacent to (a), (b) illustrates the active learning strategies (ALSs) that emerged from our categorisation of in-class activities. In the present study, an ALS is defined as a technique for accomplishing the aim of enabling active learning in the classroom. A strategy is more general than an activity in that a strategy is less concerned with implementation logistics and is more concerned with how, philosophically and strategically, to enable active learning in the classroom. The four patterns of ALSs that emerged from the analysis of in-class activities are: (i) purely independent, (ii) independent-loose, (iii) interdependent-loose, and (iv) interdependent-tight. The first strategy accounts for active learning that takes place only in the mind of the individual learner, without peer interactions.
also illustrates the following findings that emerge from our review of the literature. (i) Two-stage polling bridges the divide between no interdependence and positive interdependence. (ii) There is a large variety of in-class activities that depend on loose social interactions and that overlap with three out of the four major categories of in-class activities. (iii) The more positive interdependence and the tighter the peer interactions required for in-class activities, the greater the fraction of class time is invested in the activity.
A new model and theory
represents the ALSs model that connects in-class activities with ALSs, which are defined above. In other words, the ALS model summarises a variety of instructional practices that were implemented to enable active learning. The ALS model is also a component of a proposed theory about instructional decisions to use active learning (). For the purposes of this article, the term ‘model’ is defined as a conceptual simplification of the actual phenomenon being studied (i.e. instructional practices that enable active learning in an undergraduate science classroom environment), and the term ‘theory’ is defined as an explanation for how the phenomenon being studied may actually come to pass. As such, the ALS model is one component of the proposed theory. In the proposed theory, an activity is a lower order concept (concerned with details of implementation), a strategy is a mid-level concept (concerned with philosophical and strategic considerations), and an approach is a higher order concept. An approach is defined as a general principle for guiding instructional decisions, such as constructivism (e.g. a constructivist approach).
Figure 3. Within the sphere of the catch-all term ‘active learning’, three distinctions are made: active learning activity, active learning strategy, and active learning approach (see text for definitions). These distinctions represent different concepts of active learning and different levels of instructional decisions that enable active learning. The arrows indicate that instructional decision-making may originate from and lead to other levels of decision-making.
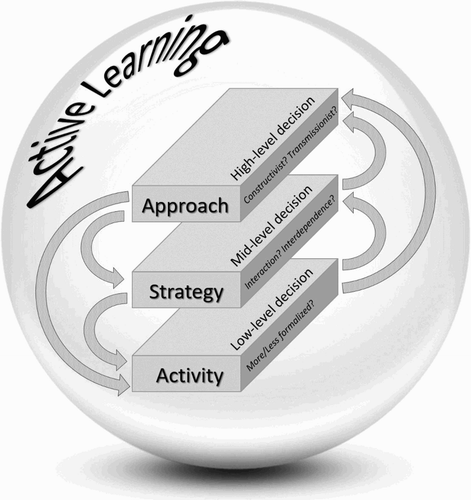
The relationships between these three ‘active learning’ concepts and levels of instructional decision-making are summarised in . In it, the approach relates to high-level instructional decisions about the general path to enable active learning; it involves deciding to use a transmissionist (teacher-centred) or constructivisit (student-centred) approach to instruction, or some combination of the two approaches. The activity pertains to low-level instructional decisions that deal with implementation details. The strategy involves mid-level instructional decisions about how in-class activities connect to more constructivist approaches to teaching, and it involves decisions about the extent to which an instructor aims to promote peer interaction and social interdependence. It is at this mid-range level that instructors may find the ALS model () useful for identifying in-class activities that facilitate active learning appropriate to their context and learning goals.
Together, and represent the proposed instructional decisions to enable active learning (IDEAL) theory. In addition to facilitating instructional design decisions as described above, the IDEAL theory can be applied to discussing timely research issues, such as instructional decision-making. For example, it can be used to classify responses to questions such as, ‘What informs an instructor’s decision to enable active learning?’ A response such as ‘the simplicity of implementation’ could be classified as a low-level decision using this theory, whereas a response such as ‘the desire to improve students’ collaborative skills using cooperative learning experiences’ could be classified as mid-level, and a response such as ‘the aim to have one’s students be creators of knowledge’ could be classified as high-level.
The theory provides a structure for dissecting the catch-all phrase of ‘active learning’ into different concepts (i.e. activity, strategy, and approach) that can be applied to instructional decisions. It is theoretically grounded in constructivist (Bransford et al., Citation1999) and socio-cultural (Johnson & Johnson, Citation2011; Vygotsky, Citation1978) learning theories. These theories form the basis of the approach and the strategy levels in the IDEAL theory, while descriptions of in-class activities drawn from the present literature review form the basis of the activity level. The IDEAL theory suggests that instructors may begin thinking about active learning at any one of the three levels and, then, move fluidly between these levels in any order and even recursively as they engage in instructional decision-making about activity design and implementation. The flexible entry point into instructional decisions that enable active learning and the possibly recursive approach to decision-making is represented by the arrows in .
At a time when college science instructors are called upon to promote active learning in their courses (Olson & Riordan, Citation2012) and are potentially overwhelmed or confused by the myriad kinds of ‘active learning’ (Prince, Citation2004), the IDEAL theory provides a framework for conceptualising the range of possible ALSs and examples of in-class activities that support those strategies. In particular, for example, they can be used to inform the instructional design of a course. Furthermore, the IDEAL theory can be used to support research about instructional decisions about active learning.
Gaps
Our review reveals an important gap in the literature. In particular, information is lacking about what leads instructors to decide to promote active learning at all. In light of ongoing calls to promote active learning in college science courses (e.g. Handelsman & Brown, Citation2016), much remains to be learned about what facilitates instructional change at the instructor level. To that end, the following questions represent gaps: (i) Why do college science instructors decide to use the in-class activities that they do? (ii) To what extent do science instructors explicitly draw upon ALSs, constructivism, and their related learning theories?
Limitations
One of the limitations to this study is that the articles reviewed were derived from only one database, ERIC. Nevertheless, the ERIC is a prominent and respected database for studies in education. As such, the literature drawn from it for this review is considered representative of the larger corpus of college science education.
Another limitation of the study is the number of articles reviewed. Undoubtedly, many more articles about in-class activities used in college science classrooms exist, particularly in discipline-based education journals such as the CBE-Life Sciences Education, Chemistry Education Research and Practice, Journal of Geoscience Education, and Physical Review Physics Education Research. However, the number of articles reviewed was appropriate for the scope and the exploratory nature of this study, providing valuable information to synthesise the literature in the form of the ALS model and the IDEAL theory.
Conclusions
Lecture alone is largely incongruent with what we know about how people learn and contemporary college science education goals. Although college instructor resistance to incorporating active learning opportunities in science courses is widespread (Pundak & Rozner, Citation2008; Walker, Cotner, Baepler, & Decker, Citation2008), our literature review suggests growing awareness and implementation of in-class activities that facilitate active learning in college science classrooms. To help combat the often confusing and overwhelming flurry of terms associated with ‘active learning’, we propose an ALSs model and the IDEAL theory. Their utilisation may facilitate instructional decision-making to enable active learning in college science classroom settings. They can also provide a framework for future research on what instructors do to promote active learning in college science classroom settings and how they arrive at those instructional decisions. Given the national calls to implement more active learning in college science classrooms (Handelsman & Brown, Citation2016; Olson & Riordan, Citation2012), the findings of this study can be applied to support and study IDEAL.
Disclosure statement
No potential conflict of interest was reported by the authors.
Additional information
Funding
References
- Addison, S., Wright, A., & Milner, R. (2009). Using clickers to improve student engagement and performance in an introductory biochemistry class. Biochemistry and Molecular Biology Education, 37(2), 84–91. doi: 10.1002/bmb.20264
- Algar, W. R., & Krull, U. J. (2010). Promoting active learning by practicing the “self-assembly” of model analytical instruments. Journal of Chemical Education, 87(9), 942–944. doi: 10.1021/ed100242e
- Amador, J. A., Miles, L., & Peters, C. B. (2007). The practice of problem based learning. Bolton, MA: Anker.
- Anderson, J. (2013). Active learning through student film: A case study of cultural geography. Journal of Geography in Higher Education, 37(3), 385–398. doi: 10.1080/03098265.2013.792041
- Angelo, T. A., & Cross, K. P. (1993). Classroom assessment techniques: A handbook for college teachers. San Francisco, CA: Jossey-Bass.
- Armbruster, P., Patel, M., Johnson, E., & Weiss, M. (2009). Active learning and student-centered pedagogy improve student attitudes and performance in introductory biology. Cell Biology Education, 8(3), 203–213. doi: 10.1187/cbe.09-03-0025
- Bailey, C. P., Minderhout, V., & Loertscher, J. (2012). Learning transferable skills in large lecture halls: Implementing a POGIL approach in biochemistry. Biochemistry and Molecular Biology Education, 40(1), 1–7. doi: 10.1002/bmb.20556
- Bandyopadhyay, A. (2013). Measuring the disparities between biology undergraduates’ perceptions and their actual knowledge of scientific literature with clickers. The Journal of Academic Librarianship, 39(2), 194–201. doi: 10.1016/j.acalib.2012.10.006
- Bloom, B. S. (1956). Taxonomy of educational objectives: The classification of education goals by a committee of college and university examiners. New York, NY: David McKay.
- Bosworth, K. (1994). Developing collaborative skills in college students. New Directions for Teaching and Learning, 1994(59), 25–31. doi: 10.1002/tl.37219945905
- Bransford, J. D., Brown, A. L., & Cocking, R. R. (1999). How people learn: Brain, mind, experience, and school. Washington, DC: National Academy Press.
- Brockliss, L. (1996). Curricula. In H. de Ridder-Symoens (Ed.), A history of the university in Europe (Vol. II, pp. 565–620). Cambridge: Cambridge University Press.
- Brogt, E. (2007). A theoretical background on a successful implementation of lecture-tutorials. Astronomy Education Review, 6(1), 50–58. doi: 10.3847/AER2007005
- Charmaz, K. (2014). Constructing grounded theory. Washington, DC: Sage.
- Christiansen, M. A. (2014). Inverted teaching: Applying a new pedagogy to a university organic chemistry class. Journal of Chemical Education, 91(11), 1845–1850. doi: 10.1021/ed400530z
- Coca, D. M., & Slisko, J. (2013). Software Socrative and smartphones as tools for implementation of basic processes of active physics learning in classroom: An initial feasibility study with prospective teachers. European Journal of Physics Education, 4(2), 17–24.
- Creswell, J. W. (2014). Research design: Qualitative, quantitative, and mixed methods approaches. Washington, DC: Sage.
- Crossgrove, K., & Curran, K. L. (2008). Using clickers in nonmajors-and majors-level biology courses: Student opinion, learning, and long-term retention of course material. Cell Biology Education, 7(1), 146–154. doi: 10.1187/cbe.07-08-0060
- Crouch, C. H., & Mazur, E. (2001). Peer instruction: Ten years of experience and results. American Journal of Physics, 69(9), 970–977. doi: 10.1119/1.1374249
- Dengler, M. (2008). Classroom active learning complemented by an online discussion forum to teach sustainability. Journal of Geography in Higher Education, 32(3), 481–494. doi: 10.1080/03098260701514108
- Deutsch, M. (1949). A theory of cooperation and competition. Human Relations, 2, 129–152. doi: 10.1177/001872674900200204
- Deutsch, M. (1962). Cooperation and trust: Some theoretical notes. In M. R. Jones (Ed.), Nebraska symposium on motivation (pp. 275–319). Lincoln: University of Nebraska Press.
- DiBenedetto, M. K., & Bembenutty, H. (2013). Within the pipeline: Self-regulated learning, self-efficacy, and socialization among college students in science courses. Learning and Individual Differences, 23, 218–224. doi: 10.1016/j.lindif.2012.09.015
- Donohue, S. (2014). Supporting active learning in an undergraduate geotechnical engineering course using group-based audience response systems quizzes. European Journal of Engineering Education, 39(1), 45–54. doi: 10.1080/03043797.2013.833169
- Duncan, D. (2006). Clickers: A new teaching aid with exceptional promise. Astronomy Education Review, 5(1), 70–88. doi: 10.3847/AER2006005
- Earth Science Literacy Initiative. (2010). Earth science literacy principles: The big ideas and supporting concepts of Earth science. Retrieved from www.earthscienceliteracy.org/es_literacy_6may10_.pdf
- Eberlein, T., Kampmeier, J., Minderhout, V., Moog, R. S., Platt, T., Varma-Nelson, P., & White, H. B. (2008). Pedagogies of engagement in science. Biochemistry and Molecular Biology Education, 36(4), 262–273. doi: 10.1002/bmb.20204
- Enghag, M., Gustafsson, P., & Jonsson, G. (2007). From everyday life experiences to physics understanding occurring in small group work with context rich problems during introductory physics work at university. Research in Science Education, 37(4), 449–467. doi: 10.1007/s11165-006-9035-4
- Fardilha, M., Schrader, M., da Cruz e Silva, O. A. B., & da Cruz e Silva, E. F. (2010). Understanding fatty acid metabolism through an active learning approach. Biochemistry and Molecular Biology Education, 38(2), 65–69. doi: 10.1002/bmb.20330
- Farrer, N. J., Monk, N., Heron, J., Lough, J. A., & Sadler, P. J. (2010). (RSC) 2: Chemistry, performance, and pedagogy – an interactive approach to periodic trends. Chemistry Education Research and Practice, 11(4), 308–313. doi: 10.1039/C0RP90011G
- Flynn, A. B. (2011). Developing problem-solving skills through retrosynthetic analysis and clickers in organic chemistry. Journal of Chemical Education, 88(11), 1496–1500. doi: 10.1021/ed200143k
- Freeman, S., Eddy, S. L., McDonough, M., Smith, M. K., Okoroafor, N., Jordt, H., & Wenderoth, M. P. (2014). Active learning increases student performance in science, engineering, and mathematics. Proceedings of the National Academy of Sciences, 111(23), 8410–8415. doi: 10.1073/pnas.1319030111
- Freeman, S., O’Connor, E., Parks, J. W., Cunningham, M., Hurley, D., Haak, D., & Wenderoth, M. P. (2007). Prescribed active learning increases performance in introductory biology. Cell Biology Education, 6(2), 132–139. doi:10.1187/cbe.06–09–0194 doi: 10.1187/cbe.06-09-0194
- Gardner, J., & Belland, B. R. (2012). A conceptual framework for organizing active learning experiences in biology instruction. Journal of Science Education and Technology, 21(4), 465–475. doi: 10.1007/s10956-011-9338-8
- Gok, T. (2012). The effects of peer instruction on students’ conceptual learning and motivation. Asia-Pacific Forum on Science Learning and Teaching, 13(1), 1–17.
- González-Sancho, J. M., Sánchez-Pacheco, A., Lasa, M., Molina, S., Vara, F., & del Peso, L. (2013). The use of an active learning approach to teach metabolism to students of nutrition and dietetics. Biochemistry and Molecular Biology Education, 41(3), 131–138. doi: 10.1002/bmb.20684
- Gray, K., & Steer, D. N. (2012). Personal response systems and learning: It is the pedagogy that matters, not the technology. Journal of College Science Teaching, 41(5), 80–88.
- Gutwill-Wise, J. P. (2001). The impact of active and context-based learning in introductory chemistry courses: An early evaluation of the modular approach. Journal of Chemical Education, 78(5), 684–690. doi: 10.1021/ed078p684
- Hageman, J. H. (2010). Use of molecular models for active learning in biochemistry lecture courses. Journal of Chemical Education, 87(3), 291–293. doi: 10.1021/ed800092d
- Halpern, D. F. (1999). Teaching for critical thinking: Helping college students develop the skills and dispositions of a critical thinker. New Directions for Teaching and Learning, 1999(80), 69–74. doi: 10.1002/tl.8005
- Handelsman, J., & Brown, Q. (2016). A call to action: Incorporating active STEM learning strategies into K-12 and higher education. Retrieved from https://www.whitehouse.gov/blog/2016/08/17/call-action-incorporating-active-stem-learning-strategies-k-12-and-higher-education
- Handelsman, J., Ebert-May, D., Beichner, R., Bruns, P., Chang, A., DeHaan, R., & Wood, W. B. (2004). EDUCATION: Scientific teaching. Science, 304(5670), 521–522. doi: 10.1126/science.1096022
- Hanson, D. M. (2006). Foundations of chemistry: Applying POGIL principles. Lisle: Pacific Crest.
- Harlen, W., & James, M. (1997). Assessment and learning: Differences and relationships between formative and summative assessment. Assessment in Education: Principles, Policy & Practice, 4(3), 365–379. doi: 10.1080/0969594970040304
- Hartley, J., & Davies, I. K. (1978). Note-taking: A critical review. Programmed Learning and Educational Technology, 15(3), 207–224. doi: 10.1080/0033039780150305
- Hein, S. M. (2012). Positive impacts using POGIL in organic chemistry. Journal of Chemical Education, 89(7), 860–864. doi: 10.1021/ed100217v
- Henderson, C., Dancy, M., & Niewiadomska-Bugaj, M. (2012). Use of research-based instructional strategies in introductory physics: Where do faculty leave the innovation-decision process? Physical Review Special Topics – Physics Education Research, 8(2), 101. doi: 10.1103/PhysRevSTPER.8.020104
- Hoekstra, A. (2008). Vibrant student voices: Exploring effects of the use of clickers in large college courses. Learning, Media and Technology, 33(4), 329–341. doi: 10.1080/17439880802497081
- Hu, H. H., & Shepherd, T. D. (2013). Using POGIL to help students learn to program. ACM Transactions on Computing Education, 13(3), 1–23. doi: 10.1145/2499947.2499950
- Irons, S. H. (2012). The Monty Hall Problem as a class activity using clickers. The Physics Teacher, 50(1), 14–16. doi: 10.1119/1.3670075
- Johnson, D. W., & Johnson, R. T. (2011). Intellectual legacy: Cooperation and competition. In Conflict, interdependence, and justice (pp. 41–63). New York, NY: Springer.
- Keller, G. E. (2002). Using problem-based and active learning in an interdisciplinary science course for non-science majors. The Journal of General Education, 51(4), 272–281. doi: 10.1353/jge.2003.0013
- Klein, P. (2003). Active learning strategies and assessment in world geography classes. Journal of Geography, 102(4), 146–157. doi: 10.1080/00221340308978539
- Koenig, K. M., Endorf, R. J., & Braun, G. A. (2007). Effectiveness of different tutorial recitation teaching methods and its implications for TA training. Physical Review Special Topics – Physics Education Research, 3(1), 24. doi: 10.1103/PhysRevSTPER.3.010104
- Kortz, K. M., Smay, J. J., & Murray, D. P. (2008). Increasing learning in introductory geoscience courses using lecture tutorials. Journal of Geoscience Education, 56(3), 280–290. doi: 10.5408/1089-9995-56.3.280
- Kovac, J. (1999). Student active learning methods in general chemistry. Journal of Chemical Education, 76(1), 120–124. doi: 10.1021/ed076p120
- Krathwohl, D. R. (2002). A revision of Bloom’s taxonomy: An overview. Theory into Practice, 41(4), 212–218. doi: 10.1207/s15430421tip4104_2
- Levesque, A. A. (2011). Using clickers to facilitate development of problem-solving skills. Cell Biology Education, 10(4), 406–417. doi: 10.1187/cbe.11-03-0024
- Lewin, K. (1935). A dynamic theory of personality. New York, NY: McGraw-Hill.
- Lin, Y. C., Liu, T. C., & Chu, C. C. (2011). Implementing clickers to assist learning in science lectures: The clicker-assisted conceptual change model. Australasian Journal of Educational Technology, 27(6), 979–996. doi: 10.14742/ajet.924
- Linton, D. L., Pangle, W. M., Wyatt, K. H., Powell, K. N., & Sherwood, R. E. (2014). Identifying key features of effective active learning: The effects of writing and peer discussion. Cell Biology Education, 13(3), 469–477. doi: 10.1187/cbe.13-12-0242
- LoPresto, M. C., & Murrell, S. R. (2009). Using the star properties concept inventory to compare instruction with lecture tutorials to traditional lectures. Astronomy Education Review, 8, doi: 10.3847/AER2009014
- Lunsford, B. E., & Herzog, M. J. R. (1997). Active learning in anatomy & physiology: Student reactions & outcomes in a nontraditional A&P course. The American Biology Teacher, 59, 80–84. doi: 10.2307/4450254
- Marbach-Ad, G., Seal, O., & Sokolove, P. (2001). Student attitudes and recommendations on active learning. Journal of College Science Teaching, 30(7), 434–438.
- Mazur, E. (1999). Peer instruction: A user’s manual. Upper Saddle River, NJ: Prentice-Hall.
- McConnell, D. A., Steer, D. N., & Owens, K. D. (2003). Assessment and active learning strategies for introductory geology courses. Journal of Geoscience Education, 51(2), 205–216. doi: 10.5408/1089-9995-51.2.205
- Meltzer, D. E., & Manivannan, K. (2002). Transforming the lecture-hall environment: The fully interactive physics lecture. American Journal of Physics, 70(6), 639–654. doi: 10.1119/1.1463739
- Middlecamp, C. H., & Nickel, A. M. L. (2000). Doing science and asking questions: An interactive exercise. Journal of Chemical Education, 77(1), 50. doi: 10.1021/ed077p50
- Miller, K., Lasry, N., Lukoff, B., Schell, J., & Mazur, E. (2014). Conceptual question response times in peer instruction classrooms. Physical Review Special Topics – Physics Education Research, 10(2), 51. doi: 10.1103/PhysRevSTPER.10.020113
- National Research Council. (1999). Transforming undergraduate education in science, mathematics, engineering, and technology. Washington, DC: The National Academies Press. doi: 10.17226/6453
- National Research Council. (2003). Evaluating and improving undergraduate teaching in science, technology, engineering, and mathematics. Washington, DC: The National Academies Press. doi: 10.17226/10024
- Nicol, D. J., & Boyle, J. T. (2003). Peer instruction versus class-wide discussion in large classes: A comparison of two interaction methods in the wired classroom. Studies in Higher Education, 28(4), 457–473. doi: 10.1080/0307507032000122297
- Nogaj, L. A. (2013). Using active learning in a studio classroom to teach molecular biology. Journal of College Science Teaching, 42(6), 50–55. doi: 10.2505/4/jcst13_042_06_50
- Odom, S., Glenn, B., Sanner, S., & Cannella, K. A. (2009). Group peer review as an active learning strategy in a research course. International Journal of Teaching and Learning in Higher Education, 21(1), 108–117.
- Olson, S., & Riordan, D. G. (2012). Engage to excel: Producing one million additional college graduates with degrees in science, technology, engineering, and mathematics. Report to the President. Executive Office of the President.
- Orvis, J. N., & Orvis, J. A. (2005). Throwing paper wads in the chemistry classroom: Really active student learning. Journal of College Science Teaching, 35(3), 23.
- Paulson, D. R. (1999). Active learning and cooperative learning in the organic chemistry lecture class. Journal of Chemical Education, 76(8), 1136. doi: 10.1021/ed076p1136
- Perez, K. E., Strauss, E. A., Downey, N., Galbraith, A., Jeanne, R., & Cooper, S. (2010). Does displaying the class results affect student discussion during peer instruction? Cell Biology Education, 9(2), 133–140. doi: 10.1187/cbe.09-11-0080
- Poushter, J. (2016). Smartphone ownership and internet usage continues to climb in emerging economies. Pew Research Center: Global Attitudes & Trends. Retrieved from Pew Research Center website: http://s1.pulso.cl/wp-content/uploads/2016/02/2258581.pdf
- Prather, E. E., Slater, T. F., Adams, J. P., Bailey, J. M., Jones, L. V., & Dostal, J. A. (2004). Research on a lecture-tutorial approach to teaching introductory astronomy for non-science majors. Astronomy Education Review, 3(2), 122–136. doi: 10.3847/AER2004019
- Prather, E. E., Slater, T. F., Brissenden, G., Adams, J. P., & Dostal, J. A. (2008). Lecture tutorials for introductory astronomy. Boston, MA: Addison-Wesley.
- Prince, M. (2004). Does active learning work? A review of the research. Journal of Engineering Education, 93(3), 223–231. doi: 10.1002/j.2168-9830.2004.tb00809.x
- Pundak, D., & Rozner, S. (2008). Empowering engineering college staff to adopt active learning methods. Journal of Science Education and Technology, 17(2), 152–163. doi: 10.1007/s10956-007-9057-3
- Ramsier, R. D. (2001). A hybrid approach to active learning. Physics Education, 36(2), 124–128. doi: 10.1088/0031-9120/36/2/307
- Reay, N. W., Bao, L., Li, P., Warnakulasooriya, R., & Baugh, G. (2005). Toward the effective use of voting machines in physics lectures. American Journal of Physics, 73(6), 554–558. doi: 10.1119/1.1862638
- Reay, N. W., Li, P., & Bao, L. (2008). Testing a new voting machine question methodology. American Journal of Physics, 76(2), 171–178. doi: 10.1119/1.2820392
- Robinson, G. D. (2001). How many years does it take for a mountain to wash into the sea? An introductory geology exercise. Journal of Geoscience Education, 49(2), 135–139. doi: 10.5408/1089-9995-49.2.135
- Schultz, E. (1997). A guided discovery approach for learning glycolysis. Biochemical Education, 25(4), 205–208. doi: 10.1016/S0307-4412(97)00118-0
- Shaver, M. P. (2010). Using low-tech interactions in the chemistry classroom to engage students in active learning. Journal of Chemical Education, 87(12), 1320–1323. doi: 10.1021/ed900017j
- Simonson, S. R., & Shadle, S. E. (2013). Implementing process oriented guided inquiry learning (POGIL) in undergraduate biomechanics: Lessons learned by a novice. Journal of STEM Education: Innovations and Research, 14(1), 56.
- Slish, D. F. (2005). Assessment of the use of the jigsaw method and active learning in non-majors, introductory biology. Bioscene: Journal of College Biology Teaching, 31(4), 4–10.
- Smith, M. K., Trujillo, C., & Su, T. T. (2011). The benefits of using clickers in small-enrollment seminar-style biology courses. Cell Biology Education, 10(1), 14–17. doi: 10.1187/cbe.10-09-0114
- Tewksbury, B. J. (1995). Specific strategies for using the “jigsaw” technique for working in groups in non-lecture-based courses. Journal of Geological Education, 43, 322–326. doi: 10.5408/0022-1368-43.4.322
- Torraco, R. J. (2005). Writing integrative literature reviews: Guidelines and examples. Human Resource Development Review, 4(3), 356–367. doi: 10.1177/1534484305278283
- Trujillo, G., & Tanner, K. D. (2014). Considering the role of affect in learning: Monitoring students’ self-efficacy, sense of belonging, and science identity. Cell Biology Education, 13(1), 6–15. doi: 10.1187/cbe.13-12-0241
- Turpen, C., & Finkelstein, N. D. (2010). The construction of different classroom norms during peer instruction: Students perceive differences. Physical Review Special Topics – Physics Education Research, 6(2), 020123. doi: 10.1103/PhysRevSTPER.6.020123
- US Global Change Research Program. (2009, March). Climate literacy: The essential principles of climate science Version 2. Retrieved from http://cpo.noaa.gov/sites/cpo/Documents/pdf/ClimateLiteracyPoster-8_5×11_Final4-11LR.pdf
- Vygotsky, L. S. (1934, 1986). Thought and language. Boston, MA: MIT Press.
- Vygotsky, L. S. (1978). Mind in society: The development of higher psychological processes. Cambridge, MA: Harvard University Press.
- Walker, J. D., Cotner, S. H., Baepler, P. M., & Decker, M. D. (2008). A delicate balance: Integrating active learning into a large lecture course. Cell Biology Education, 7(4), 361–367. doi: 10.1187/cbe.08-02-0004
- Wankat, P. C. (2002). The effective, efficient professor: Teaching, scholarship, and service. Boston, MA: Allyn and Bacon.
- Wohlin, C. (2014, May). Guidelines for snowballing in systematic literature studies and a replication in software engineering. Proceedings of the 18th international conference on evaluation and assessment in software engineering (p. 38). New York, NY: ACM.
- Zekeri, A. A. (2004). College curriculum competencies and skills former students found essential to their careers. College Student Journal, 38(3), 412.