ABSTRACT
Models are very important tools when learning and communicating about science. Models used in secondary school biology education range from concrete scale models, such as a model of a skeleton, to abstract concept-process models, such as a visualisation of meiosis. Understanding these concept-process models requires a profound understanding of the concept of models and how they are used in biology. This study evaluates an existing framework for its use in assessing students’ understanding of biological concept-process models. Four additions were required to extend the applicability of the framework to concept-process models. We were also able to give an indication of students’ current level of understanding of these models, showing room for improvement in all aspects of understanding. Since concept-process models have a central place in many scientific disciplines, it is important that students have a deep understanding of the nature, application and limitations of these models. The current study contributes to assessing the way students reason with concept-process models. Knowing how to improve students’ view on the use of concept-process models in biology may lead to higher scientific literacy.
Introduction
Models play a key role in scientific explanations and understanding. They are representations that can describe or simplify complex phenomena, be used to make predictions about future events, facilitate the communication of ideas (Svoboda & Passmore, Citation2013) or make entities visible that are impossible to observe with the naked eye (Francoeur, Citation1997). The idea of a model is not to reach ultimate truth but to make sense of the world. This means that they can be seen as theories, constructed to give meaning to observed phenomena (Duschl & Richard, Citation1990). One could say that models can either be used as representations of something, or that they can be used for something, thereby generating new data (Gouvea & Passmore, Citation2017). When models are used as representations, they often have a retrospective view because they summarise or visualise existing data. When models are used as a tool to predict future events and make sense of the world around us, they have a prospecting view (Krell & Krüger, Citation2017; Passmore, Gouvea, & Giere, Citation2014; Upmeier zu Belzen & Krüger, Citation2010). By using models in both a retrospective and prospective way, they can function as a bridge between scientific theory and the world as experienced (Justi & Gilbert, Citation2003). Models are also present in our everyday life, such as weather models that are presented in the news, models about climate change and models about vaccination and herd immunity. Therefore, knowledge about models and the process of modelling is not only important for scientists. Having a basic understanding of models and their use in science is important for decision making processes that everyone has to go through in daily life (Odenbaugh, Citation2005; Oh & Oh, Citation2011).
Students who have an elaborate understanding of science are believed to understand that both scientific knowledge and models are human constructs, designed to explain and predict parts of phenomena (Schwarz & White, Citation2005). It is important for students to understand both the retrospective and prospective view on modelling. The understanding of scientific models and the process of modelling can not only facilitate the learning of science content, but also aid in developing an understanding of the way science depicts and investigates phenomena. Students’ knowledge about science grows when they are able to define what has been depicted in a model, and are able to relate the choices that were made when creating the model to its use. Understanding the use of models in science is part of scientific meta-knowledge (White, Collins, & Frederiksen, Citation2011) and adds to students’ scientific literacy (Gilbert, Citation1991).
In secondary school biology education, the use of models ranges from concrete scale models to abstract concept-process models. The scale models, such as a model of the human skeleton, can be used to describe and simplify phenomena by depicting superficial features. The abstract concept-process models represent complex scientific processes, such as the interaction of hormones, organs and cells in the formation of sperm cells, and can be used to both make abstract entities visible and make predictions about future events. However, use of models in biology education is often limited to illustrative or communicative purposes, neglecting the scientific practice that accompanies the use of models in science. This is partly because many teachers lack experience with scientific modelling. They view models as a helpful tool for teaching about science content, but not about the nature of science (Windschitl, Thompson, & Braaten, Citation2008). This makes it difficult for students to develop a more sophisticated scientific view on the use of models in biology.
In the learning process of students, concept-process models can be particularly useful. They are process thinking models for understanding and applying important concepts, and are considered to be the most complex type of models (Harrison & Treagust, Citation2000). When these models are being used in biology, they often contain positive or negative feedback loops where depending on the type of stimulus a certain response is being provoked. For example, when we look at concept-process models of the formation of sperm cells (spermatogenesis), a negative feedback loop makes sure that the production of testosterone is inhibited when its level is above a certain value. In concept-process models about this subject, several concepts such as hormones and sperm cells are connected by arrows that represent a part of the process (see below for an example of a model of this process). Because of their abstract nature, these arrows showing the effect of time and movement on a process can be difficult for students to understand. These dynamics of time and movement are specific for concept-process models. It shows that not everything that is represented is always happening at the same time or visible in real life. Whether or not the next step within a depicted process will happen depends on the factors, or concepts, that are present in a specific situation. This situation may vary depending on time and place. For example, when we look at the model of spermatogenesis, we can see that the hormones luteinizing hormone (LH) and follicle stimulating hormone (FSH) are only being released if and after the hypothalamus secreted releasing hormone (RH). When the hypothalamus is being inhibited by oestrogen and testosterone, LH and FSH will not be released. This means that one could look at these concept-process models as a collection of possible events which occur depending on the presence or absence of certain factors.
In scientific practices, such as generating and testing hypotheses about natural processes, concept-process models play a key role. Therefore, such models can be assumed to be helpful in teaching about the nature of science. However, as with other types of models, teachers almost always use concept-process models only as an illustration of a concept or a process (Windschitl et al., Citation2008), missing the opportunity for students to learn about scientific practices. To get students to develop a more sophisticated scientific view on the use of models and be able to improve the way they reason with biological concept-process models, a better insight in students’ understanding of these models is necessary.
To assess students’ understanding of models that are being used in science education, various frameworks have been developed. Where some frameworks focus on practical skills when it comes to modelling (e.g. Louca, Zacharia, Michael, & Constantinou, Citation2011; Schwarz et al., Citation2009), others focus on learners’ understanding of models and their use in science (e.g. Crawford & Cullin, Citation2005; Grosslight, Unger, Jay, & Smith, Citation1991; Justi & Gilbert, Citation2003). Upmeier zu Belzen and Krüger (Citation2010) combined the frameworks from Crawford and Cullin (Citation2005), Grosslight et al. (Citation1991) and Justi and Gilbert (Citation2003) with theoretical approaches from Mahr (Citation2009) and Giere (Citation2004). This resulted in a theoretical framework for assessing and investigating learners’ understanding of models and their use in science. The framework contains five aspects concerning model understanding: nature of models, multiple models, purpose of models, testing models and changing models. In line with other researchers (Crawford & Cullin, Citation2005; Grosslight et al., Citation1991; Schwarz et al., Citation2009) several levels of understanding have been formulated. However, when formulating the different levels of understanding, Upmeier zu Belzen and Krüger (Citation2010) explicitly refer to Mahr (Citation2009), who defines models based on both the creation and the application of a model. This way the framework addresses both the understanding of models as an illustration of something and the understanding of models from a more methodological perspective: as an instrument to test ideas or draw conclusions while reflecting the scientific perspective of models as research tools (Treagust, Chittleborough, & Mamiala, Citation2004). To validate the theoretical framework, Grünkorn, Upmeier zu Belzen, and Krüger (Citation2014) have tested its use in assessing students’ understanding of biological models.
The five aspects within the framework reflect on different characteristics of the understanding of models and their use in science. The aspects nature of models and multiple models focuses on ontological and epistemological concepts of models. These two aspects reflect on the way models can describe and simplify phenomena (model of something). The aspects purpose of models, testing models and changing models focus on cognitive processes and the way these models are used in science. These three aspects together reflect on the fact that models can be used to test hypotheses, make predictions about future events and communicate ideas (model for something) (Grosslight et al., Citation1991; Justi & Gilbert, Citation2003). The aspects can also be related to the cognitive model of science as described by Izquierdo-Aymerich and Adúriz-Bravo (Citation2003) which focuses on how scientists do science using models. Scientists choose a strategy to pursue a scientific goal and select a model that is most appropriate for reaching this goal. The aspects purpose of models can be related to the goal that a researcher is trying to achieve, while the aspects testing models and changing models reflect on the selection of an appropriate model to achieve this goal.
In the aspect nature of models, students compare the model with the original and explain the extent to which the model can be compared with the original. The aspect multiple models refers to the fact that multiple models can be used to represent the same original, focussing on different aspects of the original or reflecting on them in a different way. Since no single model can illustrate an object or process in all its aspects, models are used to explore important and difficult aspects of a concept. For this, the model is often simplified, fitting the questioner’s need and prior knowledge and emphasising only those aspects that are of great importance to explain a certain key idea (Harrison & Treagust, Citation2000). With the aspect purpose of models, the framework tries to differentiate in the way students think about the purpose of models in science and whether the models can be used to predict the outcome of a certain input. The aspect testing models describe the way students think a model is validated, and the aspect changing models reflects on the fact that models are by definition changeable and temporary. For example, when falsification of hypotheses about the original calls for changes in the currently used model. For all of these aspects, up to four levels of students’ understandings were determined, ranging from a low level of understanding to an expert level of understanding (Grünkorn et al., Citation2014).
Since concept-process models can be particularly useful when learning about scientific reasoning but are also conceived as the most difficult models for students to reason with, it is important to know secondary students’ level of understanding for this type of models. Because the framework from Grünkorn et al. (Citation2014) has been tested for its use in assessing students’ understanding of biological models, it seems the right fit for assessing students’ understanding of biological concept-process models. However, to our knowledge, this framework has not specifically been tested for its use in assessing students’ understanding of this type of biological models.
We aim to evaluate the usability of the framework from Grünkorn et al. (Citation2014) – from this point referred to as ‘Grünkorn’s framework’ – for the assessment of students’ understanding of biological concept-process models. Therefore, the research question for this study is: To what extent can Grünkorn’s framework be used to assess students’ understanding of biological concept-process models?
The aspects and levels within the framework have been validated in a previous study (Grünkorn et al., Citation2014). This study focuses on determining whether the given categories of student answers as described in Grünkorn’s framework provide a valid assessment of students’ understanding of biological concept-process models and whether or not these categories need adaptation in this new context of use. Also, since the data that were collected during this study consist of student answers relating to the various levels and aspects within the framework, these answers are used to give an indication of students’ current understanding of biological concept-process models.
Method
To answer our research question, we interviewed students about two different concept-process models. The choice for semi-structured interviews was made so that we were able to get more in-depth insight in the reasons behind students’ answers. This made it easier to judge whether the answers matched the existing descriptions of categories within Grünkorn’s framework, or if an addition to the framework was necessary. The interview questions related to the five aspects within this framework. Student answers were then compared with the description of the levels within Grünkorn’s framework to determine whether the answers matched the existing levels. Since all answers relating to the five different aspects should fit one of the categories within the framework, answers that we could not match with the existing categories resulted in an addition to this framework.
Participants
Forty Dutch eleventh-grade students with a major in biology (16–18 years old, 19 females and 21 males) from four schools in the Netherlands participated in this study. Schools were chosen from different areas in the country. Per school, ten students participated, five on the pre-university level and five on higher general education level. Students were randomly selected, except from one school where students were assigned in class by the researcher.
Interviews
The semi-structured interview scheme was based on the five aspects within Grünkorn’s framework. Questions mostly related directly to one of the aspects. For example, the question ‘How can be determined whether this model is correct?’ relates to the aspect testing models. Other questions, such as ‘What is the meaning of the arrows in this model?’ were meant to get a deeper insight in the way students interpret different aspects or concepts that were mentioned in the model. Two pilots with a total of six secondary students were conducted to optimise the interview scheme. The students in the pilot studies were 16–18 years old and followed a major in biology (3 females and 3 males).
We used two concept-process models: one model showing the process of accumulation of DDT in the food chain () and one model about spermatogenesis, the formation of sperm cells (). Three criteria were used for selecting these models. First, we selected models that used arrows to indicate the dynamics of a certain process. This way both models are concept-process models that use the same type of indicator for the effect of time and movement. Second, we chose models that are not present in schoolbooks to avoid a difference in pre-knowledge concerning how to reason with a specific model. Third, we chose two models that show processes on a different level of biological organisation. As Knippels and Waarlo (Citation2018) stress, students find it difficult to reason between different levels of biological organisation. In this case, the model about the accumulation of DDT is on the level of ecological community and the model about spermatogenesis shows a process on both the molecular and cellular level. By presenting both models, students had to reason with both abstract and concrete concepts.
Figure 1. A concept-process model about the accumulation of DDT in the food chain. The model shows how a substance can accumulate in higher order organisms. Provided by and translated with permission from Biologie voor jou, Malmberg.
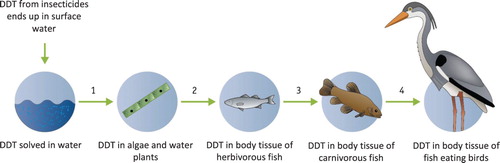
Figure 2. A concept-process model showing the role of hormones in the formation of sperm cells. Reprinted and translated with permission from archive College voor Toetsen en Examens (Citation2016).
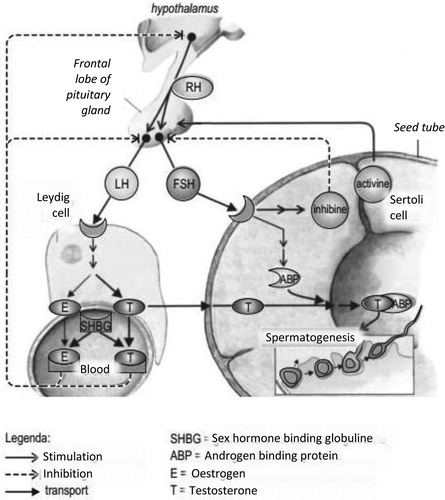
Students were interviewed individually. The interviews took about 20–30 minutes per interview and were audiotaped. To avoid bias, interviews about the two different models were counterbalanced. Half of the students started with the model about the accumulation of DDT, the other half of the students started with the model about spermatogenesis.
Analysis
The interviews were transcribed verbatim and coded using categories based on Grünkorn’s framework. For example, the code ‘Nature II’ was used to indicate that a text fragment said something about the aspect nature of models, and matched with the second level within the framework. For student answers that related to the aspects within the framework, but did not match the description of an existing level, the level of the answer was assessed and an addition to the framework was formulated. This resulted in an extension of the framework, making it suitable for application on concept-process models.
Ten percent of the interviews were coded by a second independent coder. For this, the second coder received the transcribed interview in which the fragments that were coded by the first coder were highlighted. This resulted in a Cohens’ Kappa of 0.73.
Results
Assessment of the framework
Not all student answers fit in the existing descriptions of the levels within Grünkorn’s framework. This resulted in four additions to the existing framework ().
Table 1. The revised framework, based on the framework from Grünkorn et al. (Citation2014).
First, since the models that were used showed processes, students often described these processes mentioning multiple concepts that were depicted in the model. In Grünkorn’s framework, the second and third level of the aspect nature of models fit well with the descriptions students gave about concept-process models. However, the first level of nature of models as described in the framework mentions models to be exact replicas of the original. Since concept-process models often contain drawings of arrows to connect several concepts, no student mentioned that this model including the arrows exactly matched the original. Instead, they talked about the topic of the model, explaining that these concepts together represent the process that is being depicted:
I think this [model] has something to do with food. It shows that the algae use the DDT as a food source. And small fish eat algae. And carnivorous fish eat the smaller fish. And then the heron, a fish eating bird, eats the other fish.
This way of explaining the nature of models is specific for concept-process models since the dynamics of time and movement are incorporated in the model. Students, therefore, describe what the arrows mean and are not just talking about the objects or concepts like they would do for non-dynamic biological models. To highlight this difference we added the description of the process as shown in the model to the aspect nature of models. Since students mentioned that the way the process functions is the same as it functions in the real world, this description of the process can be seen as a matching reference to the original and has therefore been added to the first level of the aspect nature of models.
Second, when looking at the aspect purpose of models, mentioning the different concepts that are present in the model and linking them would seem to lead to a level 2 understanding, since level 2 is defined by the ability to define relationships. However, since these links or relationships between concepts are already depicted in the model and were not thought of by these students themselves, one could say that the students were just reading out loud what the model was showing them instead of identifying relationships:
How could this model be used?
I think, well, perhaps for students. To show them like, here you have producers and here you have consumers. So you can explain that these eat these [pointing at organisms in model], and so on.
Also, by just following the arrows the students neglected the dynamics of space and time that are specific for concept-process models, which explain the relationships between the different concepts within the model. Therefore, just describing the events that are depicted in a model was added to the first level of the aspect purpose of models within the framework.
The third addition was made to the initial level of the aspect testing models. Several students mentioned that the only way to test whether or not the model was correct was to compare the model with their schoolbook or ask the authors of their schoolbooks whether the model was correct:
I think that the creator of the schoolbook, that he knows how it works.
They gave their schoolbooks or the authors authority by viewing their information as the absolute truth. This means that the students were not really testing the model but believed in the authority of teachers and schoolbook authors, and only checked whether the model agreed with what their schoolbook said. Therefore, we added this way of reasoning to the initial level of understanding for this aspect.
The fourth and final addition was made to the aspect changing models. A model can focus on a certain aspect of a process. For example, a model about spermatogenesis can focus on the role of hormones in the formation of sperm cells, but also on the distribution of chromosomes in the process of meiosis. Students mentioned changing a model to shift the focus that is being depicted in the model:
What would be a reason to change this image? And perhaps you can also add what kind of change that would be?
Yes, well, when the purpose of the image is different […] This is quite abstract I think. So in theory, well this is mostly about the nucleus. So you could also make clear how the rest of the cell is being influenced by this process. Or perhaps in which organs this happens […]
This way of reasoning requires knowledge about the fact that a model does not match the original, but is a focused representation. It also shows that the student knows that multiple stories can be told about the same process by shifting the focus of the model. Since level 2 for the aspect changing models only includes alterations when the model does not match the original, we decided that mentioning alterations to shift the focus to a different aspect exceeded this level of reasoning. Therefore, we added this type of alterations to the third level for this aspect. With these four additions, all utterances that referred to model understanding could be classified in one of the levels of the revised framework. This revised version of the framework is shown in .
Indication of level of understanding
In general, almost all students reached level 1 or 2 for all aspects mentioned within the framework. Almost none of the students reached the highest levels within the revised framework, except within the aspect testing models ().
Table 2. Percentage of students that reached a certain level within the framework (n = 40).
Two types of distinctions in students’ understanding can be made. First, a difference in the level of understanding can be observed when looking at the two different models that were used. As shown in , level 3 has been reached much more often by students when asked about the model on the cellular and molecular level than when asked about the model on the level of ecological community. This especially holds for the aspect testing models. For the model on the cellular and molecular level, which shows the formation of sperm cells, students often talk about ‘adding substances’ to find out what that does to the formation of sperm cells. This way of formulating a hypothesis, based on the model that is presented, corresponds to the third level of the aspect testing models:
How has been determined whether this image is correct?
You cannot just say something […] It has to be clear and correct. So, I think people will actually look at it like, is this correct? […] If there are people who want to function as subjects, then they can do tests on them. Like, if I add this substance to your body, how will your hormones respond to that?
So, you add something?
Yes, an inhibiting substance for example. So that they can say, if we add this substance, this particular process will be inhibited. Does it really have that effect?
Oh, we had something like this on an exam! […] They asked what the influence was of extra testosterone on the fertility of a man.
The second distinction in level of understanding can be made between students from pre-university level and students from higher general education level. Pre-university level students show a higher level of understanding for all five aspects within the revised framework ().
Table 3. Percentage of students that reached a certain level within the framework (n = 40).
Conclusion and discussion
Concept-process models can be particularly useful for students when learning about the nature of science. This type of models is the most complex type of models, showing a process in which for example the effect of time, movement and feedback loops is visualised. These models can be helpful for students when learning about scientific practices such as testing hypotheses. However, since concept-process models in biology education are mostly used for illustrative or communicative purposes, the opportunity to let students get more acquainted with the scientific practice that accompanies the use of models in science is often missed. This makes it difficult for students to develop a more sophisticated scientific view on the use of models in biology.
The current study investigated to what extent Grünkorn’s framework can be applied to assess students’ understanding of biological concept-process models. Our results show that additions to the framework were needed. Four descriptions were added to different levels and aspects within the framework:
Nature of models, level 1: displays a process, its components and how they are related
Purpose of models, level 1: model for showing events
Testing models, initial level: perceiving schoolbooks or their authors as authorities providing absolute truth
Changing models, level 3: alterations when the focus of the model shifts to a different aspect of the process
We could not match all of the descriptions present in Grünkorn’s framework to our results. A reason for this could be that some of the descriptions simply do not fit with biological concept-process models. For example, ‘testing the material’ might be an appropriate answer when asked how to test if a scale model of a bird is correct. But when we look at a drawing of a process, testing the material is not a likely thing to do when you want to see if the model is correct. However, our aim was to find out if Grünkorn’s framework could be used to assess students’ understanding of concept-process models, without ruling out the assessment of other types of biological models. Therefore, the only adjustments we made to the framework were additions that were necessary for students’ understanding of biological concept-process models, leaving all the other descriptions in place.
Since we matched students’ answers to the levels within the revised framework, it is possible to give an indication of the current level of students’ understanding of biological concept-process models. We found that in general students reached level 2 for most aspects within this framework. However, two types of distinctions in students’ understanding can be made. First of all, students reached the highest levels within this framework more often when asked about the model on the molecular/cellular level than when asked about the model on the level of ecological community. This especially holds for the aspect testing models. Some students mentioned that a model such as the one we presented on the molecular and cellular level was used in an exam at school. Students had to use the model to explain what happened when a certain substance was added to the original: a human being. This means that they had to formulate a hypothesis, an activity that corresponds with the highest levels of reasoning within the framework. No student mentioned having done an exercise like that when they were confronted with the model on the level of ecological community. The fact that students had done such an exercise with models on the molecular and cellular level of organisation could have triggered answers that reach the highest levels within the framework. However, further research is necessary to confirm whether this is the case.
The second distinction in levels of reasoning could be made between students from higher general education level and students from pre-university level. In general, students from the pre-university level reached the highest levels within the framework more often than students from higher general education level. Students at the pre-university level are being prepared for studying at university, where they are expected to be able to reason with models in a scientific way. Therefore, it is possible that students at pre-university level get confronted more often with situations that trigger reasoning on the highest levels within the framework than students at higher general education level. More research has to focus on reasons to explain this difference.
In this study, we used the framework from Grünkorn et al. (Citation2014) for assessing students’ understanding of biological concept-process models and extended its applicability to these models. Since these types of models are very frequently used in biology education, an adequate understanding of and the ability to reason with concept-process models is crucial for an in-depth understanding of biological phenomena. Outside of biology such models often appear in other sciences, such as physics, chemistry and economy. Given their central place in many scientific disciplines, a deep understanding of nature, application and limitations of concept-process models is an essential part of students’ scientific literacy. The current study contributes to assessing and improving the way students reason with this type of models which may lead to higher scientific literacy and their understanding of the nature of science. A next step would be to investigate how to foster students’ reasoning with concept-process models in secondary biology education in order to leverage their understanding of models of biological processes.
Disclosure statement
No potential conflict of interest was reported by the authors.
ORCID
Susanne Jansen http://orcid.org/0000-0002-4965-0915
Marie-Christine P. J. Knippels http://orcid.org/0000-0003-4989-1863
Wouter R. van Joolingen http://orcid.org/0000-0002-4271-2861
Additional information
Funding
References
- College voor Toetsen en Examens. (2016). VWO examen biologie tweede tijdvak. Retrieved from https://www.examenblad.nl/examen/biologie-vwo-2/2016/vwo?topparent=vg41h1h4i9qe
- Crawford, B., & Cullin, M. (2005). Dynamic assessments of preservice teachers’ knowledge of models and modelling. In Research and the quality of science education. Dordrecht: Springer. doi: 10.1007/1-4020-3673-6_25
- Duschl, R. A., & Richard, A. (1990). Restructuring science education: The importance of theories and their development. New York, NY: Teachers College Press.
- Francoeur, E. (1997). The forgotten tool: The design and use of molecular models. Social Studies of Science, 27, 7–40. doi: 10.1177/030631297027001002
- Giere, R. N. (2004). How models are used to represent reality. Philosophy of Science, 71, 742–752. doi: 10.1086/425063
- Gilbert, S. W. (1991). Model building and a definition of science. Journal of Research in Science Teaching, 28, 73–79. doi: 10.1002/tea.3660280107
- Gouvea, J., & Passmore, C. (2017). ‘Models of’ versus ‘Models for’. Science and Education, 26, 49–63. doi: 10.1007/s11191-017-9884-4
- Grosslight, L., Unger, C., Jay, E., & Smith, C. L. (1991). Understanding models and their use in science: Conceptions of middle and high school students and experts. Journal of Research in Science Teaching, 28, 799–822. doi: 10.1002/tea.3660280907
- Grünkorn, J., Upmeier zu Belzen, A., & Krüger, D. (2014). Assessing students’ understandings of biological models and their use in science to evaluate a theoretical framework. International Journal of Science Education, 36, 1651–1684. doi: 10.1080/09500693.2013.873155
- Harrison, A. G., & Treagust, D. F. (2000). A typology of school science models. International Journal of Science Education, 22, 1011–1026. doi: 10.1080/095006900416884
- Izquierdo-Aymerich, M., & Adúriz-Bravo, A. (2003). Epistemological foundations of school science. Science and Education, 12, 27–43. doi: 10.1023/A:1022698205904
- Justi, R. S., & Gilbert, J. K. (2003). Teachers’ views on the nature of models. International Journal of Science Education, 25, 1369–1386. doi: 10.1080/0950069032000070324
- Knippels, M. C. P. J., & Waarlo, A. J. (2018). Development, uptake, and wider applicability of the yo-yo strategy in biology education research: A reappraisal. Education Sciences, 8(129). doi: 10.3390/educsci8030129
- Krell, M., & Krüger, D. (2017). University students’ meta-modelling knowledge. Research in Science and Technological Education, 35, 261–273. doi: 10.1080/02635143.2016.1274724
- Louca, T. L., Zacharia, Z., Michael, M., & Constantinou, P. C. (2011). Objects, entities, bahaviors and interactions: A typology of student-constructed computer-based models of physical phenomena. Journal of Educational Computing Research, 44, 173–201. doi: 10.2190/EC.44.2.c
- Mahr, B. (2009). Information science and the logic of models. Software and Systems Modeling, 8, 365–383. doi: 10.1007/s10270-009-0119-2
- Odenbaugh, J. (2005). Idealized, inaccurate but successful: A pragmatic approach to evaluating models in theoretical ecology. Biology and Philosophy, 20, 231–255. doi: 10.1007/s10539-004-0478-6
- Oh, P. S., & Oh, S. J. (2011). What teachers of science need to know about models: An overview. International Journal of Science Education, 33, 1109–1130. doi: 10.1080/09500693.2010.502191
- Passmore, C., Gouvea, J. S., & Giere, R. (2014). Models in science and in learning science: Focusing scientific practice on sense-making. In M. Mathews (Ed.), International handbook of research in history, philosophy and science teaching. Dordrecht: Springer. doi: 10.1007/978-94-007-7654-8
- Schwarz, C. V., Reiser, B. J., Davis, E. A., Kenyon, L., Achér, A., Fortus, D., … Krajcik, J. (2009). Developing a learning progression for scientific modeling: Making scientific modeling accessible and meaningful for learners. Journal of Research in Science Teaching, 46, 632–654. doi: 10.1002/tea.20311
- Schwarz, C. V., & White, B. Y. (2005). Meta-modeling knowledge: Developing students’ understanding of scientific modeling. Cognition and Instruction, 23, 165–205. doi: 10.1207/s1532690xci2302_1
- Svoboda, J., & Passmore, C. (2013). The strategies of modeling in biology education. Science and Education, 22, 119–142. doi: 10.1007/s11191-011-9425-5
- Treagust, D. F., Chittleborough, G. D., & Mamiala, T. L. (2004). Students’ understanding of the descriptive and predictive nature of teaching models in organic chemistry. Research in Science Education, 34, 1–20. doi: 10.1023/B:RISE.0000020885.41497.ed
- Upmeier zu Belzen, A., & Krüger, D. (2010). Modellkompetenz im biologieunterricht [model competence in biology education]. Zeitschrift Für Didaktik Der Naturwissenschaften, 16, 41–57.
- White, B. Y., Collins, A., & Frederiksen, J. R. (2011). The nature of scientific meta-knowledge. Models and Modeling. Dordrecht: Springer. doi: 10.1007/978-94-007-0449-7
- Windschitl, M., Thompson, J., & Braaten, M. (2008). Beyond the scientific method: Model-based inquiry as a new paradigm of preference for school science investigations. Science Education, 92, 941–967. doi: 10.1002/sce.20259