ABSTRACT
‘A lack of prior knowledge in the other discipline’ is often given by geoscience instructors to explain why students struggle to apply knowledge from other disciplines to the Earth. We examine this assumption by considering the disciplinary crossing of buoyancy (physics) to isostasy (geoscience). We investigate the teachers’ perspective through workshops, and use the theoretical framework of Interdisciplinary Reasoning and Communication to explore what geoscience textbooks ask of students. Most introductory textbooks rely on students applying pre-existing knowledge of hydrostatics in a geoscience context (forwards transfer), while intermediate and advanced textbooks develop the necessary physics explicitly in a geoscientific context (backwards transfer). Moreover, introductory textbooks frequently use analogies for concrete translation between physics and geoscience ideas, but the analogical relations and where they break down (relational translations), receive little attention. This places high demands on students’ prior understanding of hydrostatics to successfully integrate and transfer knowledge from physics to geoscience. If students lack the relevant physics background, introductory textbooks may force them to rely on intuitive ideas of physics, potentially reinforcing old, and giving rise to new misconceptions. Our results move the focus from perceived shortcomings of students to the demands made on them in interdisciplinary learning.
Introduction
Geoscience combines a wide range of concepts, models and insights from other natural sciences, e.g. chemistry, physics, and biology, and applies them to the Earth system. As a result, ‘undergraduate curricula [in geosciences] typically require more cognate science and mathematics courses than other science domains’ (Pyle et al., Citation2018). Topics that originate from physics and chemistry have been identified as particularly problematic for geoscience students. This can reflect the students’ prior level of understanding of the cognate topics (King, Citation2012), and/or the prevalence of misconceptions (Anderson & Libarkin, Citation2016). A different way of approaching the challenges students face in applying e.g. physics or chemistry to the Earth system is to consider what happens as topics from cognate sciences cross over to geoscience. This moves the focus from what teachers think students ought to know from before to how topics are presented to the students in geoscience courses and the demands made on students in interdisciplinary (sensu Shen et al., Citation2015) learning in geoscience.
To date, most Discipline-Based Education Research has focused on transfer of learning within single disciplines, and most work on knowledge transfer (between courses) has been in chemistry (Singer et al., Citation2012 Ch. 7). In geoscience education, the physics topic of elasticity has been found to cause students difficulty in its application to the Earth as students may not ordinarily think of rocks as elastic (Dolphin & Benoit, Citation2016; Hubenthal, Citation2018). However, these discussions did not explore the possible origins of student difficulties. Asking why students appear to struggle with the cross-disciplinary knowledge transfer was an important motivating factor of this study.
In this study, we investigate isostasy as an example of a topic based on physics (buoyancy) that is applied to the Earth system in the form of isostasy, where it can cause geoscience students difficulties. Isostasy is typically introduced in introductory geoscience courses and further explored in intermediate and advanced geophysics courses. It has wide-ranging explanatory power, from the first order topography of the Earth, as expressed in the relative elevations of continental and oceanic crust, to basin subsidence in response to sedimentation, uplift in response to erosion, and post-glacial rebound.
The focus of this paper is not so much to emphasise that isostasy is a troublesome topic for students, but that it is an example of an interdisciplinary topic that can be challenging for students. By analysing this example of a topic crossing a disciplinary boundary, we can get a better understanding of what it is about the disciplinary crossing that can cause students trouble, and show how such topics can be investigated systematically. To guide the investigation we use the recently developed theoretical framework of Interdisciplinary Reasoning and Communication (Shen et al., Citation2015).
Isostasy
In the introduction to his book Isostasy and flexure of the lithosphere Watts (Citation2001) lays out the importance of isostasy:
Isostasy is a concept that is fundamental to the Earth Sciences. It is based on the idea that the light crust is floating on the denser underlying mantle. Isostasy is an ideal state: a condition of rest that the crust and mantle would tend to in the absence of disturbing forces. […] [T]he modern ideas of isostasy [are] that the outer layers of the Earth are strong and capable of supporting loads for long periods of geological time. The strength manifests itself in the flexing of the crust and mantle in the region of loads such as volcanoes and sediments. Some loads are large enough that they cause the crust and upper mantle to break rather than flex. (Watts, Citation2001 Pg. ix-x)
From an historical perspective, and restricted to the equilibrium situation of isostasy, the relevant physics topic is buoyancy. Buoyant equilibrium is achieved on continental scales, and buoyancy models explain variations of bathymetry and topography over large distances (upwards of c. 1000 km), including the elevation of continental crust and mountain chains, and increasing ocean depth away from mid-oceanic ridges. Flexure models explain depression and rise structures associated with e.g. continental ice sheets, seamounts, orogenic foreland basins and subduction zone trenches, in which lithospheric elasticity is an important mechanism (Watts, Citation2001).
Introductory textbooks in geoscience aim to cover processes ranging from the atomic to the macro scale, across timescales ranging from near instantaneous to billions of years, and isostasy is usually afforded only a small amount of space. In Scandinavia, it can be assumed that students have some background in physics from secondary school, but little background in geoscience; assumptions that seem to hold true for much of higher education internationally. For teaching based in Scandinavia, isostasy is particularly relevant due to Palaeozoic (vertical movements associated with the Caledonian orogeny), Cenozoic (regional uplift) and contemporaneous (post-glacial rebound) geological processes that shape much of the geography and geology of the countries. In this paper, we consider mainly the historic, buoyant form of isostasy since this is most common in introductory textbooks, and where the interdisciplinary references are particularly clear.
Theoretical framework: Interdisciplinary reasoning and communication
The theoretical framework used for analysing the discipline-crossing in this paper is that of Interdisciplinary Reasoning and Communication (Shen et al., Citation2015). This relatively recent framework identifies and connects four processes – transformation, integration, translation and transfer of knowledge – that operate across three boundaries – syntactic, semantic and pragmatic – required for successful interdisciplinary work. Processes are facilitated by shared ‘boundary objects’ that mediate the communication processes. The basic framework is sketched in (adapted from Fig. 4 in Shen et al., Citation2015).
Figure 1. Summary of the Interdisciplinary Reasoning and Communication framework developed in Shen et al. (Citation2015). The different processes, mediated by boundary objects, are all interconnected. Transformation aspects (grey) are not of major concern in introductory courses dealing with well-established knowledge. Our focus is therefore on how Translation, Integration and Transfer of knowledge takes place.
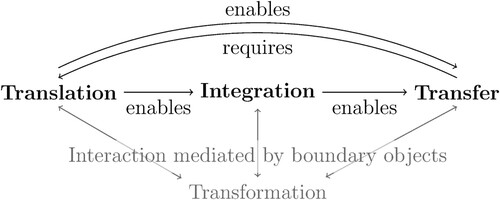
According to Shen et al. (Citation2015), transformation occurs when an existing system is modified for application in a new context. This is where there is the greatest opportunity for creative and conceptual shifts to occur. The transformation is the result of incompatible views from different disciplinary perspectives and can be exemplified by the argumentation used by G. B. Airy when he introduced the ideas that became isostasy (Airy, Citation1855). To achieve transformation, whether of a specific system or a concept, three other interconnected processes are required; knowledge transfer, integration and translation.
The framework was developed by Shen et al. (Citation2015) in connection to a case study of disciplinary experts working in a multi-disciplinary team. In this paper, we use it to expand our understanding of the demands made on students in interdisciplinary learning in geoscience. Within most (introductory) educational contexts, the focus is on students learning already established knowledge; hence, our primary concern is not transformation, but the three processes required for topics from cognate sciences to successfully cross over to geoscience. These are briefly introduced below.
Knowledge transfer can be either ‘forwards’, where knowledge from one’s own disciplinary area is used in a new context, or ‘backwards’ when one applies knowledge from a different discipline to further understand one’s area of expertise. In this paper, we examine both of these routes of transfer. Being able to transfer knowledge from one domain to another requires that the knowledge is first incorporated into and understood in relation to one’s cumulative body of knowledge, and is the result of successful knowledge integration.
Knowledge integration can be subdivided into two parts. Commonality integration is the knowledge that is shared between or common to several disciplines. Differential integration, meanwhile, involves the organisation of concepts from different disciplines into a coherent whole, but one in which the different areas of knowledge are recognised as distinct. Thus integration is a prerequisite for transfer. When a concept is used in different disciplines and contexts, it may go by different names, or be used in slightly different ways, and therefore require translation.
Translation may be terminological, i.e. simply introducing terms from one discipline in another, or relational, in which the relationships between terms are described and explained. Translation may also be concrete, in which the translation is contextualised trough concrete examples that the interlocutors can relate to, drawing on shared knowledge. Commonality integration may require minimal translation for successful transfer, while differential integration often requires considerable amounts of translation. Shen et al. (Citation2015) argue that translation often is overlooked in interdisciplinary activities, and should receive more attention.
Mediating the processes are boundary objects, which may also be of a variety of types. For example: symbolic meta-representations that aid integration (e.g. equations in physics); specific concepts requiring translation (e.g. buoyancy, from hydrostatics); models, both existing and novel, that support reasoning (that, in physics, may be described using equations).
Research aims and questions
The goal of this exploratory study is to develop an understanding of why isostasy may be perceived as difficult for students by exploring the different ways in which a seemingly simple physics topic (buoyancy) is introduced to students in relation to a specific geoscience concept (isostasy). Disciplinary perspectives are provided by university teachers and researchers, and introductory and intermediate geoscience textbooks provide examples of possible ways in which the topic can be introduced. The Interdisciplinary Reasoning and Communication framework (Shen et al., Citation2015) is used to scaffold the analysis. The following research questions are addressed:
What are some of the discipline-specific differences between the typical presentation of buoyancy in physics and its realisation in the Earth context as isostasy?
What are some of the routes by which the physics topic of buoyancy ‘becomes’ the geoscience topic of isostasy?
What interdisciplinary actions are being asked of students when the physics topic of buoyancy is applied to the Earth as isostasy?
Methods
This study employs a mixed-methods approach, combining workshop data on the qualitative understanding of teaching and learning isostasy from the teacher’s point of view and content analysis of textbooks.
Data sources
Isostasy was chosen as an example of a physics-based topic that can cause students difficulty on the suggestion of AML who teaches the second semester introductory geoscience course at the University of Oslo. The topic was explored as part of two workshops with staff from the Department of Geosciences, University of Oslo. The participants were informed ahead of the workshops that the results, an anonymous collective account of the disciplinary crossing from physics to geoscience, and a conceptual map of troublesome aspects of isostasy, would contribute to a research project. The workshops provide a perspective related to backwards transfer – the use of knowledge from another discipline in geoscience.
The first workshop was attended by eight geoscience teachers, and provided an account of what happens in the move from buoyancy to isostasy. The second workshop was attended by a subset of five teachers from the first workshop, during which a concept map of connected troublesome topics involving isostasy was created, and reasons for why students may struggle with isostasy were explored from the teachers’ perspective.
One author (KD) has a research background in physics and is currently pursuing research in science education. As a newcomer to geoscience (less than one year working with geoscience education at the time of reviewing the disciplinary transfer), this provides a novice geoscientist perspective, and given the physics background, a forwards transfer perspective. One of the ways in which the presentation of isostasy in the introductory textbooks was considered was therefore explicitly on the basis of a physics-first perspective. The self-set question was: ‘What questions do I have if I examine this presentation strictly through my physics disciplinary knowledge?’
The 14 introductory textbooks (dated 1996–2022) were those available in the department’s textbook collection. Several editions were available of many of these textbooks, and the latest edition was chosen. Only textbooks with ‘isostasy’ in the index were considered; where there were several textbooks with different titles but the same authors, the most recent and most general (e.g. geosciences or Earth sciences rather than geology) was chosen. This reduces overlap and bias due to multiple occurrences of individual authors. Up to the fact that all textbooks are written by US-based authors and focus on descriptive presentations, generally avoiding mathematical descriptions, the textbooks can be taken to provide examples of a range of possible presentations.
The six intermediate and the advanced textbook were found in the University of Oslo library; some of them are listed as course textbooks for intermediate Bachelor’s and Master’s level courses at the department, others were found through browsing, or recommended by staff. The editions of the intermediate textbooks were dated 2000–2014. The presentation in the advanced textbook is very similar to what is implemented in a modelling programme used for research into post-glacial rebound (Spada, Citation2003; Spada et al., Citation2003). Therefore, the advanced textbook is taken to reflect the understanding that underlies modern research. The most relevant pages introducing isostasy were identified using index entries. A list of all 21 textbooks consulted is given in the Supplementary Material.
Analysis
The geoscience teachers’ reflections on disciplinary crossing and physicist’s (KD) interpretation of the comparison of a mountain range with blocks of wood in water were organised thematically before analysis. The concept map developed by the geoscience teachers was condensed and ‘inverted’ to focus on isostasy as the common concept.
The introductory textbooks were examined to identify the range of presentations of the topic. From this, we constructed a concept map of some of the ways in which buoyancy and isostasy are connected.
Intermediate textbooks were subjected to a content analysis to explore how these more technical introductions to isostasy are structured. An initial inductive thematic analysis (Braun & Clarke, Citation2006) of the introductory textbooks generated a list of topics. This list was used to guide the writing of summaries of the presentations of isostasy in intermediate textbooks. These summaries were then reviewed and the order in which the topics were introduced noted. This led to a list of topics for each intermediate textbook, ordered according to the sequence in which they occurred. This was also applied to the table of contents of the advanced textbook (Watts, Citation2001).
The Interdisciplinary Reasoning and Communication framework (Shen et al., Citation2015) is then used to analyse the complexity of the tasks asked of students when applying physics knowledge to the earth system in the form of (quasi-)equilibrium large scale isostasy where buoyancy is the predominant support mechanism.
Throughout, face validity and the continual testing of the codes developed across the different data sets were the main forms of ensuring trustworthiness (Robson & McCartan, Citation2016). Where relevant, the consistency of the results both within the data sets (single level) and between data sets (different levels) provided an additional confirmation of relevance. The relatively large number of textbooks and the temporal distribution of their publication dates provides an indication of the generalisability and representativeness of the reported results. The result of the workshops were corroborated in further discussions with lecturers of geosciences and geophysics courses at the University of Oslo and other Norwegian universities, and attendees at seminars given to both physics and geoscience education researchers.
Results
Why do students struggle with isostasy? The teacher perspective
A workshop with five geoscience teachers identified that several topics involving isostasy cause problems for students, e.g. Relative Sea Level and Marine Limit (). The problems can reflect students’ lack of understanding or misunderstandings. They can also reflect a reliance on intuitive ideas of physics, i.e. a feeling of what the correct answer is without evidence or proof. Since some aspects of buoyancy can be perceived as quite counter-intuitive (e.g. the 5-blocks problem, Loverude et al., Citation2003; and the hydrostatic paradox, Noxaïc & Fadel, Citation2022), a reliance on intuition may give rise to new misunderstandings and misconceptions.
Figure 2. Isostasy as a troublesome physics-dependent topic that is also an element of other topics staff identified as troublesome for students. Examples of troublesome topics are in square boxes, proposed causes in hexagonal boxes and one outcome in the oval box. For example, Relative Sea Level reflects the combined effects of isostasy (uplift/subsidence of the landsurface) and eustacy (global sea level change), and the Marine Limit reflects the interplay of Relative Sea level and the time of deglaciation at a site.
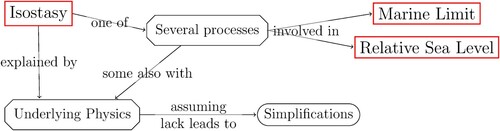
The staff-proposed explanation of student difficulties with isostasy was that the students had insufficient knowledge of the underlying physics. With limited time in geoscience courses to cover the relevant material, teachers employ simplifications, which in turn can introduce or strengthen misunderstandings and misconceptions. This is often compounded by the complexity of having several processes contributing to real-world features (cf. ). This is in keeping with other studies exploring student difficulties with material from cognate disciplines (Anderson & Libarkin, Citation2016; Francek, Citation2013).
From buoyancy to isostasy: disciplinary lenses
In a workshop, eight geoscience teachers discussed what happens as buoyancy (articulated as ‘Archimedes’ principle’, as in some introductory textbooks) moves discipline from physics to geoscience. The discussion focussed on what is lost and gained when the topic is taken from its original discipline and introduced to explain some aspect of the new discipline. This is summarised in , and has been corroborated in research seminars with students, teachers and educational researchers in geoscience.
Table 1. Crossing disciplines: gains and losses from physics (Archimedes’ principle) to geoscience (isostasy) from a workshop with geoscientists (backwards transfer perspective; Shen et al., Citation2015).
On the physics side of simplicity is the result of being able to consider a highly idealised, isolated system that one can (in theory) control precisely, and that is amenable to an exact, generalised mathematical description. In contrast, we find that, in the geoscience context, the concept from physics provides a reasonable explanation of the underlying process, but one is generally considering a specific example that is complicated by connectivity and interactions over a wide range of space and time scales. Moreover, explicit, deliberate human control is rarely possible, so one it reliant on ‘snapshot’ observations, sometimes indirect (cf. Ault, Citation1998).
To identify potential issues with a direct mapping from the physics concept of buoyancy and floatation to isostasy, KD applied a physics perspective to the most common presentation of isostasy in the textbooks: a row of blocks of wood of different heights as an analogue to the ‘roots’ of a mountain chain (specifically Fig. 10.26 of Tarbuck et al. (Citation2012)). This was done in the initial stage of the research project, and the contents of have not been subsequently updated beyond explanatory comments in square brackets because it (a) reflects a novice position within geoscience, and (b) foregrounds physics knowledge. The approach involved deliberately being rather literal in the interpretation and understanding of the diagram while drawing explicitly on physics knowledge. Many of the questions that arose have subsequently been corroborated in discussions with physics lecturers and education researchers.
Table 2. Crossing disciplines: initial questions from a physics perspective (forwards transfer; Shen et al., Citation2015) when faced with a presentation of isostasy related to floating blocks of wood and Archimedes principle.
The names of the phenomena are included in to call attention to the translation required by the students. The table also highlights the difference between the clarity of the parts and their properties in the physics (blocks of wood in water) case and the uncertainty about the parts and properties in the mountain range and mantle (a range of materials under physical conditions outside of human experience). As a novice in geoscience but drawing on a physics background, the nature of the asthenosphere as a ‘partial melt’ that is essentially solid led to several related questions about the location and properties of the liquid layer that corresponds to the water in which the blocks of wood float. These reflected knowledge of the assumptions made in basic hydrostatics, and concepts from thermal physics that arise with temperature and pressure variations with depth. A related concern appears in considering the behaviour of lithospheric rocks, when compared to the implied properties of blocks of wood. The list highlights the gaps that students may find themselves negotiating if they deliberately try to apply physics knowledge directly from the blocks of wood example to a mountain range.
Connecting buoyancy and isostasy in introductory textbooks
Having established some disciplinary perspectives on buoyancy and isostasy, we now consider how the topic is presented in textbooks, especially introductory textbooks, which are likely to represent the first time students encounter isostasy in geoscience, and have the broadest circulation. There was considerable variation in the structure of the presentations of isostasy in the introductory textbooks. Some textbooks started with the definition of isostasy, others explain at least one situation (a consequence of isostasy), with or without analogy, before giving the definition. Other textbooks used an analogy to everyday experience of floating objects, some of which were discussed in some detail, either as a digression or as a separate segment. One textbook moves back and forth between a single analogy and a range of Earth features that are then explained by isostasy.
Some of the ways in which the physics concept of buoyancy is related to isostasy in introductory textbooks are outlined in . Relatively intuitive ideas are shown on the upper half of the figure (above the dashed central line), whereas the lower part contains more technical expressions, often associated with documented student difficulties (Bierman & Kincanon, Citation2003; Gette et al., Citation2018; Lan, Citation2000; Loverude et al., Citation2003, Citation2010; Psillos, Citation1999; Suarez et al., Citation2017; Yadav, Citation2014). Some introductory geoscience textbooks switch implicitly between explanations of floating based on density differences (e.g. the higher average elevation of continental compared to oceanic lithosphere is due to density differences) and a more formal buoyancy-based explanatory model of floating and sinking phenomena in which the forces involved are explicitly considered (most often found in the glossary entries). While both models can be taught successfully, the formal buoyancy-based model is more complicated (Schwichow & Zoupidis, Citation2023; Shen et al., Citation2017). The presence of both models indicates another potential complication for students.
Figure 3. Concept map relating buoyancy in physics and the presentation of isostasy as hydrostatic equilibrium in introductory and intermediate geoscience textbooks, with some of the approaches taken. The shaded boxes are how Buoyancy is often formalised in Physics, and were predominantly found in definitions of isostasy, including in glossary entries. Textbooks that use isostasy to 'explain' regular floating phenomena (e.g. of blocks of wood) misrepresent the contextualisation of the concept.
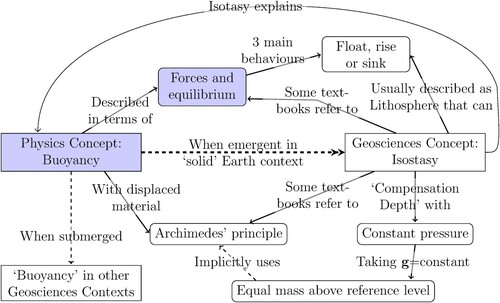
The two shaded boxes in are the Physics discipline formulation: 11 of the 14 glossary entries for isostasy mentioned force balance or equilibrium, but the pages in which isostasy was introduced more substantially did not usually expand on these concepts significantly beyond the definitions. Given the lack of explanations in the textbooks, it appears that a prior understanding of the concepts of force balance and equilibrium is assumed. The ‘Constant pressure’ node is formally equivalent to the ‘Forces and equilibrium’ node but has an explicit framing within hydrostatics, an area of known student difficulties (e.g. Loverude et al., Citation2010).
Isostasy in intermediate level textbooks
Sections of six intermediate textbooks (introductions to geophysics as an area of geoscience) and one advanced textbook specifically on isostasy were examined to explore whether there is a consistent approach to introducing isostasy at more advanced levels. The relevant pages were identified through the index and summarised guided by a set of eight codes developed from introductory textbooks. The resulting summaries were in their turn coded to extract the organisation of the presentations.
The eight codes were:
Buoyancy/floatation: mention of floatation or any aspect of buoyancy, including hydrostatic pressure, Archimedes’ principle and equilibrium.
Elastic lithosphere: reference to the strength and the bending or flexing of the lithosphere.
Asthenosphere flow: mention of the viscous nature of the asthenosphere or mantle.
Airy model and Pratt model: explicit mention of the name or recognisable reference to the relevant model.
Examples: features or behaviours of the Earth presented as explained by, or manifestations of isostasy.
Analogy: comparison with or use of a more tangible, often everyday example to illustrate the discussion.
Historical context: reference to particular developments, either explicitly (year or century) or implicitly (relative to another development).
In five of the six intermediate textbooks, the introduction was confined to a single chapter or section (sometimes with interleaving sections on e.g. the geoid or gravitational anomalies). In the sixth textbook, the introduction to isostasy was spread across sections within five chapters. Despite this, the order in which the elements are introduced is broadly similar. All textbooks devote some time to developing the idea of an elastic lithosphere, although this is usually after both the buoyancy (Airy and Pratt) models have been firmly established. Historical references were also common. Most intermediate textbooks also introduce the geoid and gravitational anomalies (either immediately before or as subsections in the presentation of isostasy). These two topics are not typically included in the introductory textbook presentations of isostasy, and they were therefore not considered in the analysis.
A flowchart of how isostasy was introduced in each textbook was made on the basis of the coded summary and checked by reviewing it against the original pages. It was found that isostasy is presented in a fairly consistent manner in intermediate textbooks, and the flowcharts could be combined to outline a common approach to introducing isostasy, as shown in . Although flexure of the elastic lithosphere is always introduced, the buoyancy-based models are typically introduced first and discussed in more detail.
Figure 4. Typical structure of presentation of isostasy in intermediate textbooks. Brackets indicate features that were not always present or could have limited detail.
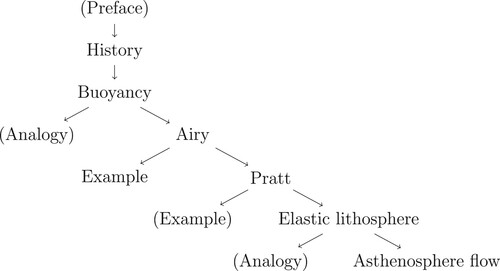
The same structure was present in the advanced textbook (Watts, Citation2001). Here, the Pratt and Airy buoyancy-based models are part of the detailed historical introduction that also covers their use and weaknesses and the subsequent development of flexural models. The bulk of the book and its technical content is dedicated to (visco)elastic (flexural) models of isostasy with examples illustrating the models. The flow of the asthenosphere was not discussed as an independent chapter or section.
Discussion
In the following, we apply the Interdisciplinary Reasoning and Communication framework (Shen et al., Citation2015) to our results to better understand what is being asked of geoscience students in applying knowledge of the Physics concept buoyancy to the Earth system as isostasy.
Translation
Translation focusses on the linguistic aspect of interdisciplinarity, and the need for translation is perhaps the most obvious of the interdisciplinary challenges. The translation of physics terminology (e.g. buoyancy, Archimedes’ principle, reference level of constant pressure) to geoscience-specific terminology (e.g. isostasy, partial melt, compensation depth) goes beyond applying established terms from physics to the Earth system, and introduces students to new, specialised vocabulary. The meanings of these new terms do not always correspond precisely to the original terms in physics. This means that a degree of relational translation, i.e. explicit exploration of the relations between physics and geoscience ideas, is required if students are to apply understanding from physics in geoscience. However, in introductory textbooks, the ideas are typically only described from the perspective of geoscience (intradisciplinary explanations; Shen et al., Citation2015). One notable failure of translation in several textbooks is the misuse of the term 'isostasy' to explain buoyancy phenomena not related to the lithosphere, as seen in .
Instead, the textbooks make use of concrete translation, i.e. contextualising the translations through examples (Shen et al., Citation2015). Especially in introductory textbooks, the concrete translation is commonly approached through the use of metaphors and analogies. In our sample of 14 introductory textbooks, 13 used at least one analogy in connection with introducing isostasy, such as ice or blocks of wood floating in water as an analogue of lithosphere floating on asthenosphere. This is no doubt intended to make the translation from physics to geoscience easier by starting with a simple system (idealised, isolated, within human experience; cf. ) and moving to the complex Earth system (many interrelated processes and time scales, materials and physical conditions outside of human direct experience). Analogies can be powerful educational tools, but may also obscure important differences between the analogical relations (translated terms), and mislead students (Duit et al., Citation2001).
For example, mapping the fluid in the wood-floating-in-water analogy to the asthenosphere can easily introduce misconceptions about the nature of the mantle (see questions in ; cf. King, Citation2008; Kirby, Citation2011). This underlines the importance of explicitly exploring the relevant features of the analogy, and where it breaks down (Glynn, Citation2008). Such explicit exploration of the analogies is not undertaken in the introductory textbooks.
Transfer
The transfer of knowledge between two disciplines can take the form of forwards transfer (in this case, applying physics already familiar to the students to their new field of study, geoscience) or backwards transfer (here, introducing physics to geoscience students in the context of geoscience). In the previous sections, we have explicitly identified some of the aspects of the transfer, from both the backwards () and the forwards () perspectives. Depending on their prior knowledge of hydrostatics, students may attempt to perform either type of transfer in connecting buoyancy to isostasy.
However, teachers in this study specifically identify insufficient knowledge of the underlying physics as a challenge in teaching isostasy. Also, hydrostatics is known to cause students problems (Bierman & Kincanon, Citation2003; Gao et al., Citation2020; Gette et al., Citation2018; Loverude et al., Citation2010; Radovanović & Sliško, Citation2013; Valiyov & Yegorenkov, Citation2000; Wagner et al., Citation2009), and is one of the topics most likely to be omitted from introductory physics courses (Drazin, Citation1987; Erceg et al., Citation2019; Loverude et al., Citation2010; University of Oslo courses). This suggests that forwards transfer may not be a realistic option for many students.
This reality is not reflected in the buoyancy to isostasy descriptions in most of the introductory textbooks examined. In 12 of 14 introductory textbooks, the brevity of introductions to physics ideas and the paucity of relational translation between physics and geoscience terms, coupled with little exploration of the analogies presented (the concrete translations), indicate that the books to a large degree rely on forwards transfer, i.e. assumes prior knowledge of physics. If students lack the relevant background in hydrostatics, they may be forced to rely on intuitive ideas of physics, which in turn may both reinforce old, and give rise to new misconceptions in students’ understanding of isostasy.
As seen in in which a textbook presentation was examined on the basis of prior physics knowledge, even forwards transfer based on an extensive knowledge of physics is not without its potential difficulties and confusions in the absence of explicit definitions and explanations (i.e. translation sensu Shen et al., Citation2015). In contrast, the two remaining introductory textbooks and all the intermediate/advanced textbooks provide an expanded introduction to the relevant physics concepts. In these instances, the necessary physics is introduced in the geoscience context, which may be seen as backwards transfer.
Integration
An underlying assumption of teaching is that students will integrate new knowledge into their understanding of the world. In the Interdisciplinary Reasoning and Communication framework, integration results from comparing and contrasting ideas from different disciplines (physics and geoscience) to organise them into a connected whole (differential integration), and to identify a common set of knowledge (commonality integration). This requires successful translation, and in turn allows successful transfer (application of ideas from physics in geoscience) to take place (Shen et al., Citation2015). However, we have already noted that introductory geoscience textbooks sampled in this study, in contrast to intermediate and advanced textbooks, show a strong tendency to rely on concrete translation through metaphors and analogies, without providing support for detailed comparing and contrasting of ideas. This suggests that students using the introductory textbooks may struggle to achieve meaningful integration in the sense of the Interdisciplinary Reasoning and Communication framework.
These observations may help explain an apparent paradox noted by Anderson and Libarkin (Citation2016). Geoscience students do not show significant improvement on basic physics and chemistry questions in introductory geoscience courses as measured by pre- and post-course Geoscience Concept Inventory scores, yet these scores are high when upper- and graduate-level students are tested. Where, how and why does the learning occur? Our analysis of the disciplinary crossover of buoyancy from physics to isostasy in geoscience using the Interdisciplinary Reasoning and Communication framework suggests that this may simply (and perhaps to no great surprise) reflect the different ways in which the students are taught at introductory and intermediate levels.
The complexity of introducing isostasy: what do we ask of students?
The use of the Interdisciplinary Reasoning and Communication framework highlights how translation, transfer and integration are approached differently in introductory and intermediate/advanced textbooks. In one sense, the common complaint from teachers (including in this study) can be said to be correct: in many cases the students simply may not possess the physics background required to make proper use of the introductory textbooks, leaving them with a largely intuitive understanding of isostasy. However, the framework moves the focus from perceived shortcomings of students to the way in which the crossover from physics to geoscience is facilitated (or not) in introductory textbooks, and how textbooks and teachers better can support students undertaking disciplinary crossings. The reliance on concrete translation in the form of metaphor and analogy with little in the way of relational translation (explanation of the relations between the ideas), coupled with an assumption of forwards transfer (students taking introductory geoscience courses already possess the relevant physics knowledge) combines to make successful integration and transfer of knowledge from physics to geoscience seem unlikely.
In addition, the discussions with teachers/researchers in geoscience and physics underline the importance of the differences between the nature and practices of geoscience and physics (cf. Ault, Citation1998), for example, the use of quantitative (equation-based) or qualitative (explanation-based) descriptions, and the challenges of moving from an ideal system (buoyancy) to a real, contextualised one (isostasy).
Conclusions
The goal of this exploratory study was to investigate why moving topics between disciplines may be challenging to students, using the example of buoyancy (physics) and isostasy (geoscience). To do so, we have systematically considered the interdisciplinary nature of introductions to isostasy, building our analysis around the Interdisciplinary Reasoning and Communication framework (Shen et al., Citation2015). This is a novel use of the framework that helps instructors articulate exactly what is being asked of students, and uncover tacit assumptions about the learners in inherently interdisciplinary courses.
We find that, somewhat unexpectedly, the majority of introductory textbooks appear to assume forwards transfer of a prior understanding of hydrostatics, whereas intermediate textbooks develop the necessary physics explicitly, permitting backwards transfer, i.e. students learn the relevant physics in the context of geoscience. Introductory textbooks rely heavily on concrete translation (contextualised examples) in the form of metaphor and analogy, with little space devoted to relational translation (explanations of the relations between the ideas). This approach places high demands on students’ prior understanding of hydrostatics to successfully integrate and transfer knowledge from physics to geoscience. In the absence of the relevant physics background, students may be forced to rely on intuitive ideas of physics, which may both reinforce old and give rise to new misconceptions in students’ understanding of isostasy. Since many students lack a background in hydrostatics, it seems to us that asking students to integrate and transfer knowledge from physics to geoscience based on typical introductory textbooks is unreasonable.
By focussing on the example of buoyancy (physics) and isostasy (geoscience) we have been able to explore one example of a disciplinary crossing in some detail from both physics and geoscience disciplinary perspectives, highlighting the complexity of the task and some of the potential challenges students may face. We propose that this approach may be helpful when examining the many disciplinary crossings students are asked to engage with in geosciences, as well as in other subjects.
Ethics approval
The data reported in this paper were collected in line with the relevant institutional and national ethical guidelines, and required no ethical approval (pre-approval for the study to this effect was given by the Norwegian Centre for Research Data in March, 2022).
Supplemental Material
Download PDF (65 KB)Acknowledgments
The workshop participants, and Jason P. Davies of University College London, are warmly acknowledged for their input to what has become and . We would also like to thank the anonymous reviewer for their support and suggestions.
Disclosure statement
No potential conflict of interest was reported by the authors.
Additional information
Funding
References
- Airy, G. B. (1855). On the computation of the effect of the attraction of mountain-masses, as disturbing the apparent astronomical latitude of stations in geodetic surveys. Philosophical Transactions of the Royal Society of London, 145, 101–104. https://doi.org/10.1098/rstl.1855.0003
- Anderson, S. W., & Libarkin, J. C. (2016). Conceptual mobility and entrenchment in introductory geoscience courses: New questions regarding physics’ and chemistry’s role in learning earth science concepts. Journal of Geoscience Education, 64(1), 74–86. https://doi.org/10.5408/14-017.1
- Ault, C. R., Jr. (1998). Criteria of excellence for geological inquiry: The necessity of ambiguity. Journal of Research in Science Teaching, 35(2), 189–212. https://doi.org/10.1002/(SICI)1098-2736(199802)35:2<189::AID-TEA8>3.0.CO;2-O
- Bierman, J., & Kincanon, E. (2003). Reconsidering Archimedes’ principle. The Physics Teacher, 41(6), 340–344. https://doi.org/10.1119/1.1607804
- Braun, V., & Clarke, V. (2006). Using thematic analysis in psychology. Qualitative Research in Psychology, 3(2), 77–101. https://doi.org/10.1191/1478088706qp063oa
- Dolphin, G., & Benoit, W. (2016). Students’ mental model development during historically contextualized inquiry: How the “Tectonic Plate” metaphor impeded the process. International Journal of Science Education, 38(2), 276–297. https://doi.org/10.1080/09500693.2016.1140247
- Drazin, P. (1987). Fluid mechanics. Physics Education, 22(6), 350. https://doi.org/10.1088/0031-9120/22/6/004
- Duit, R., Roth, W. M., Komorek, M., & Wilbers, J. (2001). Fostering conceptual change by analogies—Between Scylla and Charybdis. Learning and Instruction, 11(4-5), 283–303. https://doi.org/10.1016/S0959-4752(00)00034-7
- Erceg, N., Aviani, I., Grlaš, K., Karuza, M., & Mešić, V. (2019). Development of the kinetic molecular theory of liquids concept inventory: Preliminary results on university students’ misconceptions. European Journal of Physics, 40(2), 0025704. https://doi.org/10.1088/1361-6404/aaff36
- Francek, M. (2013). A compilation and review of over 500 geoscience misconceptions. International Journal of Science Education, 35(1), 31–64. https://doi.org/10.1080/09500693.2012.736644
- Gao, Y., Zhai, X., Andersson, B., Zeng, P., & Xin, T. (2020). Developing a learning progression of buoyancy to model conceptual change: A latent class and rule space model analysis. Research in Science Education, 50(4), 1369–1388. https://doi.org/10.1007/s11165-018-9736-5
- Gette, C. R., Kryjevskaia, M., Stetzer, M. R., & Heron, P. R. L. (2018). Probing student reasoning approaches through the lens of dual-process theories: A case study in buoyancy. Physical Review Physics Education Research, 14(1), 010113. https://doi.org/10.1103/PhysRevPhysEducRes.14.010113
- Glynn, S. M. (2008). 5.1 Making science concepts meaningful to students: teaching with analogies. Four Decades of Research in Science Education-from Curriculum Development to Quality Improvement: From Curriculum Development to Quality Improvement, 113.
- Hubenthal, M. (2018). Exploring undergraduates’ conceptions of elasticity, within a plate tectonics context, before and after experience with rock’s elastic behavior. Journal of Geoscience Education, 66(4), 261–277. https://doi.org/10.1080/10899995.2018.1493964
- King, C. (2008). The earth science misconceptions of some science writers: How wrong can they be? Teaching Earth Sciences, 33(2), 9–11.
- King, H. (2012). Student difficulties in learning geoscience. Planet, 25(1), 40–47. https://doi.org/10.11120/plan.2012.00250040
- Kirby, K. (2011). ‘Easier to address’ earth science misconceptions. http://serc.carleton.edu/NAGTWorkshops/intro/misconception_list.html.
- Lan, B. L. (2000). Don’t run naked and shout “Eureka!” yet. The Physics Teacher, 38(2), 125–126. https://doi.org/10.1119/1.880449
- Loverude, M. E., Heron, P. R. L., & Kautz, C. H. (2010). Identifying and addressing student difficulties with hydrostatic pressure. American Journal of Physics, 78(1), 75–85. https://doi.org/10.1119/1.3192767
- Loverude, M. E., Kautz, C. H., & Heron, P. R. L. (2003). Helping students develop an understanding of Archimedes’ principle. American Journal of Physics, 71(11), 1178–1187. https://doi.org/10.1119/1.1607335
- Noxaïc, A. L., & Fadel, K. (2022). How to use the Archimedes Paradox for educational purposes. The Physics Teacher, 60(2), 137–139. https://doi.org/10.1119/10.0009424
- Psillos, D. (1999). Teaching fluids: Intended knowledge and students’ actual conceptual evolution. International Journal of Science Education, 21(1), 17–38. https://doi.org/10.1080/095006999290813
- Pyle, E. J., Darling, A., Kreager, Z., & Conrad, S. H. (2018). Research on students’ conceptual understanding of geology/solid earth science content. In K. St. John (Ed.), Community framework for geoscience education research (pp. 9–16). National Association of Geoscience Teachers.
- Radovanović, J., & Sliško, J. (2013). Applying a predict–observe–explain sequence in teaching of buoyant force. Physics Education, 48(1), 28–34. https://doi.org/10.1088/0031-9120/48/1/28
- Robson, C., & McCartan, K. (2016). Real world research (4th ed.). Wiley.
- Schwichow, M., & Zoupidis, A. (2023). Teaching and learning floating and sinking: A meta-analysis. Journal of Research in Science Teaching, 1–30. https://doi.org/10.1002/tea.21909
- Shen, J., Liu, O. L., & Chang, H. Y. (2017). Assessing students’ deep conceptual understanding in physical sciences: An example on sinking and floating. International Journal of Science and Mathematics Education, 15(1), 57–70. https://doi.org/10.1007/s10763-015-9680-z
- Shen, J., Sung, S., & Zhang, D. (2015). Toward an analytic framework of interdisciplinary reasoning and communication (IRC) processes in science. International Journal of Science Education, 37(17), 2809–2835. https://doi.org/10.1080/09500693.2015.1106026
- Singer, S. R., Nielsen, N. R., & Schweingruber, H. A. (eds.). (2012). Discipline-based education research: Understanding and improving learning in undergraduate science and engineering. The National Academies Press.
- Spada, G. (2003). The theory behind TABOO. Samizdat Press.
- Spada, G., Antonioli, A., Boschi, L., Brandi, V., Cianetti, S., Galvani, G., Giunchi, C., Perniola, B., Piana Agostinetti, N., Piersanti, A., & Stocchi, P. (2003). TABOO, user guide. Samizdat Press.
- Suarez, A., Kahan, S., Zavala, G., & Marti, A. C. (2017). Students’ conceptual difficulties in hydrodynamics. Physical Review Physics Education Research, 13(2), 020132. https://doi.org/10.1103/PhysRevPhysEducRes.13.020132
- Tarbuck, E. J., Lutgens, F. K., & Tasa, D. (2012). Earth science (13th ed.). Pearson Prentice Hall.
- Valiyov, B. M., & Yegorenkov, V. D. (2000). Do fluids always push up objects immersed in them? Physics Education, 35(4), 284. https://doi.org/10.1088/0031-9120/35/4/12
- Wagner, D. J., Cohen, S., & Moyer, A. (2009). Addressing student difficulties with buoyancy. AIP Conference Proceedings, 1179(1), 289–292. https://doi.org/10.1063/1.3266739
- Watts, A. B. (2001). Isostasy and flexure of the lithosphere. Cambridge University Press.
- Yadav, M. K. (2014). Clarifying the misconception about the principle of floatation. Physics Education, 49(5), 523–525. https://doi.org/10.1088/0031-9120/49/5/523