Abstract
Objective
To investigate the potential molecular mechanism of traditional Chinese medicine Guizhi Fuling Wan (GZFLW) inhibiting granulosa cells (GCs) autophagy in polycystic ovary syndrome (PCOS).
Methods
Control GCs and model GCs were cultured and treated with blank serum or GZFLW-containing serum. The levels of H19 and miR-29b-3p in GCs were detected using qRT-PCR, target genes of miR-29b-3p were identified using luciferase assay. The protein expressions of Phosphatase and tensin homolog (PTEN), Matrix Metalloproteinase (MMP)-2, and Bax were measured using western blot. The level of autophagy was detected via MDC staining, the degree of autophagosomes and autophagic polymers was observed using dual fluorescence-tagged mRFP-eGFP-LC3.
Results
GZFLW intervention reduced the expression of autophagy-related proteins PTEN, MMP-2 and Bax, by upregulating the expression of miR-29b-3p and downregulated the expression of H19 (p < .05 or p < .01). The number of autophagosomes and autophagy polymers was significantly decreased by GZFLW treatment. However, the inhibition of miR-29b-3p and overexpression of H19 induced a significant increase in the number of autophagosomes and autophagic polymers, which attenuated the inhibitory effect of GZFLW on autophagy (p < .05 or p < .01). In addition, inhibition of miR-29b-3p or overexpression of H19 can attenuate the effect of GZFLW on the expression of PTEN, MMP-2 and Bax proteins (p < .05 or p < .01).
Conclusion
Our study found that GZFLW inhibits autophagy in PCOS GCs via H19/miR-29b-3p pathway.
Introduction
Polycystic ovary syndrome (PCOS) is the common endocrine and metabolic disease that severely affects the physical and mental health of 8–20% of women of childbearing age worldwide [Citation1,Citation2]. The etiology and pathogenesis of PCOS have not been elucidated, but ongoing extensive research indicates toward insulin resistance, hyperandrogenism and abnormal ovulation being the chief causes and the prominent endocrine characteristics of PCOS [Citation3,Citation4]. Alterations in oocyte competence (OC) are considered potential causative factors for subfertility in women with PCOS [Citation5]. Altered oocyte development and decreased endometrial competence, such as a potentially impaired endometrial–embryo cross-talk, are present in women with PCOS [Citation6]. Metformin and hormone therapy have been used to tackle the secondary symptoms of PCOS, but are reported to produce certain side effects, including hepatotoxicity, nausea, vomiting and blurred vision, which increase the risk of breast and endometrial cancer [Citation7,Citation8]. Therefore, novel intervention strategies are required to prevent or mitigate PCOS.
Autophagy is closely correlated to insulin resistance and plays an important role in the PCOS pathogenesis [Citation9]. Autophagy is abnormally activated in ovarian granulosa cells (GCs) of patients with PCOS, and its inhibition of autophagy can significantly improve PCOS symptoms [Citation10]. For instance, metformin improves PCOS symptoms in rats by reducing excessive autophagy of ovarian GCs through the Phosphatidylinositol 3-kinase,/protein kinase B (PKB/AKT) and mechanistic target of rapamycin (PI3K/AKT/mTOR) pathway [Citation11]. Similarly, acupuncture techniques also regulate the autophagy of ovarian GCs in PCOS rats with LncMEG3 as the PI3K/AKT/mTOR pathway inhibitor [Citation12]. In addition to classical signaling pathways, non-coding RNAs play a key role in regulating autophagy in various ways [Citation13,Citation14]. lncRNA H19 is a positive regulator of autophagy; overexpression of H19 activates autophagy. In contrast, miR-29b-3p overexpression inhibits Phosphatase and tensin homolog (PTEN) and reduces Matrix Metalloproteinase (MMP)-2 and MMP-9 levels, thereby alleviating PCOS symptoms [Citation15,Citation16]. Therefore, it is reasonable to deduce that autophagy mediated by the H19/miR-29b-3p signaling pathway is closely related to PCOS and its pathogenesis.
In China, Guizhi Fuling Wan (GZFLW) is a classical prescription originating from the Synopsis of Prescriptions of the Golden Chamber, and composed by Gui Zhi, Fu Ling, Tao Ren, Bai Shao, and Dan Pi, and is commonly used for the treatment of various gynecological diseases. Furthermore, GZFLW has been included in the Chinese Pharmacopeia for the treatment of endometritis, irregular menstruation, uterine fibroids, ovarian tumors, infertility and other gynecological disorders [Citation17]. Similar to metformin, GZFLW is efficacious in improving insulin resistance and promoting mature follicular development and ovulation in patients with PCOS, as well as in improving insulin sensitivity in insulin-resistant PCOS model rats by remodeling intestinal homeostasis [Citation18–20]. Importantly, a recent study indicated toward the therapeutic properties of GZFLW and its efficacy in the treatment of PCOS. Our study confirmed these findings and proved that GZFLW can ameliorate granulosa autophagy in rats with PCOS [Citation19]. However, the molecular mechanism underlying GZFLW in the regulation of autophagy is yet to be understood.
In this study, we used the drug-containing serum of GZFLW to culture ovarian GCs to further investigate the effect of GZFLW on autophagy and the molecular mechanism based on the H19/miR-29b-3p gene target.
Materials and methods
Study protocol
To verify the targeting relationship between H19, PTEN, MMP-2, Bax and miR-29b-3p, we co-transfected WT or MT cells of H19, PTEN, MMP-2, Bax with miR-29-3p mimics or mimics NC, and performed Luciferase report analysis. Meanwhile, to clarify the expression of H19, miR-29b-3p, PTEN, MMP-2 and Bax in ovarian GCs of the PCOS model group and the changes after intervention with GZFLW containing serum therapy, qRT-PCR was used to evaluate the expression of H19 and miR-29b-3p in ovarian GCs with or without GZFLW treatment. We co-transfected GCs with miR-29b-3p-inhibitor, miR-29b-3p-inhibitor + H19 overexpression. MDC and EGFP-LC3 were used to detect autophagy levels in GCs treated with or without GZFLW, as well as the effects of miR-29b-3p blockers and overexpression of H19 on GZFLW regulated autophagy levels. Finally, miR-29b-3p was inhibited and H19 was overexpressed, respectively. The expression levels of PTEN, MMP-2 and Bax proteins in GCs treated with or without GZFLW were detected using WB. The effects of miR-29b-3p inhibitors and overexpression of H19 on GZFLW regulation of PTEN, MMP-2 and Bax were also observed.
Drugs and reagents
GZFLW is a product of Chengdu Jiuzhitang Jinding pharmaceutical Co. Ltd (No. 190202, China). Fetal bovine serum was purchased from HyClone (USA). DMEM/F12 medium was purchased from Gibco Laboratories (USA). QuickMutationTM Plus site-directed mutagenesis kit and luciferase assay kit were purchased from Beyotime Biotechnology (China). Q-PCR master kit and plasmid extraction kit were purchased from Takara (Japan). The iScript cDNA Synthesis Kit was purchased from Bio-Rad (USA). The pLVX-EGFP-C1 was purchased from Creative Biogene (USA). The pMIR-REPORTE plasmid was purchased from Ambion. Antibodies anti-PTEN, MMP2, Bax, GAPDH were purchased from Abcam (UK).
Determination of cinnamic acid and paeonol in GZFLW using HPLC
Extraction of cinnamic acid: GZFLW was weighed (1 g) and ground, added 50% methanol ultrasonicated for 30 min, centrifuged and filtered, obtained 40 mg/mL of cinnamic acid solution. Extraction of paeonol: The same extraction method as described above was used and obtained 4 mg/mL of paeonol solution. Then, cinnamic acid and paeonol reference solution 200 µg/mL were prepared. The cinnamic acid and paeonol in GZFLW were determinated by SHIMADZU LC-2030C high performance liquid chromatograph with PDA detector. The column temperature was 30 °C, with flow rate 1.0 mL/min. Acetonitrile-0.1% phosphoric acid water (30:70) was used as mobile phase, and the detection wavelength was 285 nm to determination of cinnamic acid. Methanol water (60:40) was used as mobile phase, and the detection wavelength was 274 nm to determination of paeonol.
Animal grouping and PCOS model
The BALB/c mice (6 week) were obtained from Sichuan University Experimental Animal Center (Chengdu, China), and housed in environment with 22 °C ± 2 °C and 12h light/dark cycle [Citation21]. Twenty mice were randomly divided into control group and PCOS model group. The PCOS model group was gavaged with letrozole dissolved in carboxymethyl cellulose (CMC) and high-fat emulsion for 21 days. The control group was administered CMC solution for 21 days. All animal experiments were approved by the Ethics Committee of Chengdu University of Traditional Chinese Medicine and the animal procedure was based on the National Institutes of Health’s Guide to the Care and Use of Laboratory Animals.
Preparation of drug-containing serum
Additional 20 female BALB/c mice were randomly divided into two groups, treated with GZFLW (70.2 mg/kg) and an equal volume of sterile saline separately [Citation19,Citation20]. All groups were treated twice a day for 3 days. One hour after the last administration, mice were anesthetized and blood samples were collected from the abdominal aorta. Serum was heat inactivated (56 °C for 30 min) and filtered (0.2 μm). The separated GZFLW drug-containing serum and the blank serum were frozen and stored in –20 °C refrigerator.
Cell transfection
GCs of ovarian tissue in the control group and PCOS model group mice were collected, and cultured in 10% FBS. When the cells reached 85% confluence, the cells were washed with PBS and digested with 0.25% trypsin. The cells were seeded into six-well plates and divided into the following groups: control group (control cells + blank serum), model group (PCOS cells + blank serum), GZFLW group (PCOS cells + GZFLW serum), GZFLW + miR-29b-3p-inhibitor group (PCOS cells + GZFLW serum + miR-29b-3p-inhibitor), and GZFLW + miR-29b-3p-inhibitor group + H19 group (PCOS cells + GZFLW serum + miR-29b-3p-inhibitor + H19 overexpression). At 48-h posttreatment, the cells from different groups were harvested for subsequent assays.
Vector construction, mutagenesis and luciferase assay
The H19 and PTEN, MMP-2, Bax 3′-UTR regions, containing potential miR-29b-3p binding sites, were predicted using online databases. The full length of H19 and 3′-UTRs of PTEN, MMP-2, Bax mRNAs containing the miR-29b-3p binding site was amplified by PCR. Site-directed mutagenesis of miR-29b-3p was carried out using the QuikChange Site-directed Mutagenesis Kit. Therefore, wild-type or mutant-type H19-MT, PTEN-MT, Bax-MT, MMP-2-MT luciferase reporter plasmids were constructed. Cells were co-transfected with wild-type or mutated-type luciferase reporter vector and miR-29b-3p mimics or mimics-NC for 48-h transfection.
RT-PCR
Total RNA was extracted using TRIzol. RNA was reverse transcribed into cDNA using the iScript cDNA Synthesis Kit. qRT-PCR was conducted using the 7500 Real-Time PCR System. The relative expression of H19 and miR-29b-3p was calculated by 2–ΔΔCt method, with GAPDH or U6 as the reference gene.
Western blot
Cell protein was extracted, quantified by BCA method. The sample protein was boiled for 5 min, followed by SDS-PAGE electrophoresis and transferred to PVDF membrane. The membrane was incubated with the primary antibody overnight. The membrane was washed with TBST and incubated in HRP-conjugated secondary antibody for 1 h. Protein bands were detected using Tanon 6600 Luminescent Imaging Workstation. Images were analyzed using Image Pro-Plus 6.0 software.
GC autophagy assay
MDC: cell suspension was mixed with MDC staining solution and incubated at room temperature for 30 min. Then cells were washed twice with wash buffer and resuspended. Slides were prepared by dropping the cell suspension on wet slides. Finally, cells were examined by fluorescence microscopy and photographed. mRFP-EGFP-LC3: Cells were seeded in six-well plates, and 50 μL mRFP-GFP-LC3 adenoviral particles were added into each well, infected for 24 h. The number of autophagosomes and autophagic polymers were evaluated by detecting the intensity of green fluorescence and red fluorescence, respectively.
Statistical analysis
All data were analyzed using GraphPad Prism software (Version 9.3). Student’s t test and one-way ANOVA were applied, and changes were considered statistically significant for p < .05.
Results
Content of cinnamic acid and paeonol in GZFLW
According to the calculation of external standard one point method, the contents of cinnamic acid and paeonol in GZFLW are 0.021 mg/g and 1.689 mg/g (), respectively. The Chinese Pharmacopeia stipulates that cinnamic acid and paeonol in GZFLW shall not be less than 0.012 mg/g and 1 mg/g, respectively. Therefore, the content of GZFLW accusatory ingredients used in this study conforms to the requirements of Chinese Pharmacopeia.
GZFLW downregulates H19 expression and upregulates miR-29b-3p expression
We used luciferase reporter analysis of H19-WT or H19-MT cells co-transfected with either miR-29-3p mimics or mimics NC. Results showed that H19 was the direct target of miR-29b-3p, and the co-transfection of miR-29b-3p analogue and H19-WT significantly inhibited luciferase activity () (p < .01). qRT-PCR was used to assess the expression of H19 and miR-29b-3p in GCs with or without GZFLW treatment. As shown in , the expression of H19 was significantly higher and that of miR-29b-3p was lower in the GCs of the PCOS model group than in the control group. In contrast, the expression of H19 was significantly downregulated, and that of miR-29b-3p was upregulated after GZFLW administration (p < .01).
GZFLW downregulates PTEN, MMP-2 and Bax expression
We examined the miR-29b-3p binding sites in the 3′-UTR of PTEN, MMP-2, and Bax. Compared to co-transfection with MT-type, co-transfection of the PTEN-WT, MMP-2-WT and Bax-WT binding site reporter and miR-29b-3p mimics strongly reduced the relative luciferase activity (p < .05 or p < .01; ). Furthermore, we explored the effect of GZFLW on the expression of PTEN, MMP-2 and Bax in GCs. Compared to control group, the expression levels of PTEN, MMP-2 and Bax in the PCOS model group were significantly increased (p < .01; ). In contrast, the expression levels of PTEN, MMP-2 and Bax were significantly decreased after GZFLW treatment.
Figure 3. GZFLW downregulates PTEN, MMP-2 and Bax protein expression. (A–D) Luciferase assay identified the correlation between miR-29b-3p and PTEN, MMP-2 and Bax. (E–H) Western blot analysis of PTEN, MMP-2 and Bax. ##p < .01 in comparison with controls, *p < .05, **p < .01 in comparison with model.
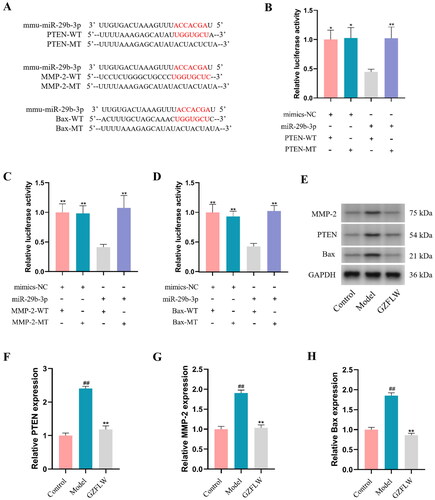
GZFLW regulates H19/miR-29-3p to inhibit autophagy
After MDC staining, the number of autophagic vesicles in GCs was detected using a fluorescence microscope. Compared to control group, the number of autophagic vacuoles (green) in the model group was significantly increased, whereas GZFLW treatment markedly reduced the number of autophagic vacuoles (p < .01; ). Moreover, the inhibition of miR-29b-3p or overexpression of H19 attenuated the inhibition of autophagy by GZFLW (p < .05).
Figure 4. GZFLW regulates H19/miR-29-3p to inhibit autophagy. (A) Fluorescence image of autophagy vesicles after MDC staining. (B) The number of autophagy vesicles. (C and D) The number of autophagosomes and autophagy polymers. (E) Fluorescent images of autophagosomes (green) and autophagy polymers (red). #p < .05, ##p < .01 in comparison with controls, **p < .01 in comparison with model, &p < .05 in comparison with GZFLW.
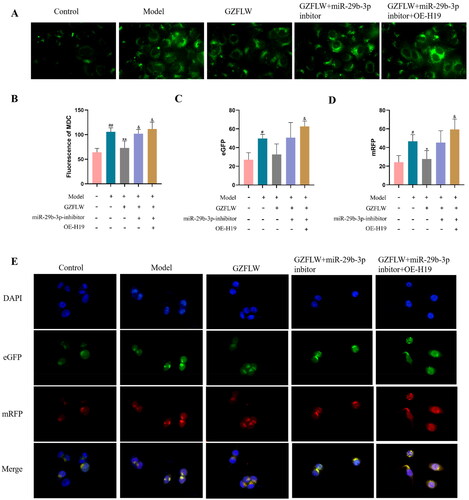
We measured autophagosomes (green) and autophagic polymers (red) using dual fluorescence-tagged mRFP-eGFP-LC3. Compared with control group, the number of autophagosomes and autophagy polymers induced in the model group was significantly increased, whereas the number of autophagosomes and autophagy polymers was significantly decreased by GZFLW treatment (p < .01; ).
GZFLW can inhibit H19 and upregulate miR-29b-3p, thereby reducing the protein expression of PTEN, MMP-2 and Bax
We also explored the effect of GZFLW on the activity of the H19/miR-29b-3p signaling pathway. As shown in , the protein expression levels of PTEN, MMP-2 and Bax were significantly decreased after GZFLW treatment (p < .01), while inhibition of miR-29b-3p reduced the effect of GZFLW. In addition, the expression of miR-29b-3p was inhibited when H19 was overexpressed, and the expression levels of PTEN, MMP-2 and Bax proteins were significantly increased (p < .01). These results suggested that inhibition of miR-29b-3p or overexpression of H19 can attenuate the effect of GZFLW on the expression of PTEN, MMP-2 and Bax proteins.
Figure 5. GZFLW affected the activity of the H19/miR-29b-3p signaling pathways. (A–D) Western blot analysis of PTEN, MMP-2 and Bax proteins transfected with miR-29b-3p or expression of H19. ##p < .01 in comparison with controls, *p < .05 and **p < .01 in comparison with model, &p < .05 and &&p < .01 in comparison with GZFLW.
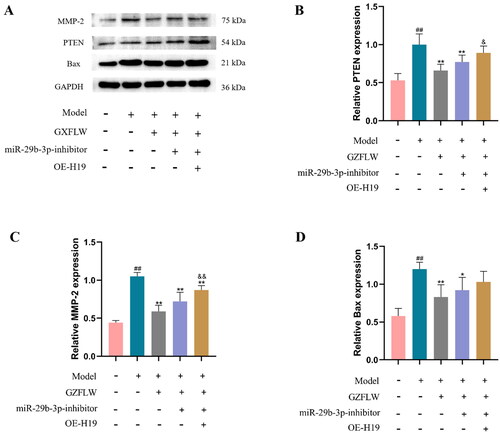
Discussion
Prior studies have shown that 30% of infertility cases are caused by anovulation, whereas 90% of anovulations are caused by PCOS [Citation22,Citation23], and women with PCOS show an increased risk of pregnancy complications [Citation24]. A large amount of experimental data is available supporting an ‘endometrial factor’ in infertility/subfertility related to PCOS and the influence of features/characteristics of the syndrome on the endometrium [Citation6]. GCs are essential for the composition of follicles as well as functional cells, which are closely related to the maturation and ovulation of egg cells. Abnormal autophagy of GCs in PCOS may lead to ovarian dysfunction, and inhibiting excessive autophagy has a protective effect against PCOS [Citation10]. GZFLW, a well-known prescription in gynecology, is effective in treating PCOS by regulating endocrine function and ovulation. Furthermore, GZFLW can effectively inhibit excessive autophagy in PCOS GCs; however, its specific mechanism has not been clarified [Citation19]. lncRNAs H19 and miR-29b-3p are involved in the regulation of ovarian function and the occurrence of related diseases [Citation15]. lncRNA H19 expression was significantly upregulated in PCOS ovarian tissue and GCs, whereas miR-29b-3p, an endogenous competing RNA of H19, was confirmed to regulate the expression of H19, MMP-9 and MMP-2 and reduce PCOS-related autophagy [Citation15].
We first screened the H19-paired 3′-UTR miRNA, and the luciferase assay results showed miR-29b-3p as a potential target of H19. Second, we observed that GZFLW treatment significantly downregulated H19 expression and upregulated miR-29b-3p expression in PCOS GCs, suggesting a potential mechanism by which GZFLW inhibits autophagy by regulating the H19/miR-29b-3p pathway. Therefore, in this study, the regulatory mechanism of GZFLW in autophagy was further investigated through the H19/miR-29b-3p pathway.miR-29b-3p affects cell proliferation by regulating autophagy-related proteins [Citation15]. Clinical studies have found that PTEN levels are significantly increased in patients with PCOS, and PTEN overexpression can induce LC3II (marker protein for autophagosomes) production and increase autophagy, while miR-29b-3p can inhibit PTEN expression [Citation25,Citation26]. An imbalance exists between MMP-2 induction and autophagy, which can directly lead to PCOS [Citation15]. Moreover, miR-29b-3p directly targets MMP-2 and inhibits its expression. Bax is a key regulator of autophagy and apoptosis, and can promote autophagy [Citation27]. In this study, the luciferase assay results showed that PTEN, MMP-2 and Bax are directly bound to miR-29b-3p, whereas western blot analysis showed how GZFLW negatively regulated the protein expression of PTEN, MMP-2 and Bax in PCOS GCs.
Autophagosome formation is the gold standard for determining the occurrence and degree of autophagy. We further examined the formation of autophagic vesicles, number of autophagosomes and autophagic polymers, and expression of autophagy-related molecules. Our results confirmed the presence of a large number of autophagy vesicles in PCOS GCs, which then significantly reduced in number due to the effect of GZFLW treatment. However, after inhibition of miR-29b-3p and overexpression of H19, GZFLW-induced inhibition of autophagy was reversed. Consistently, the number of autophagosomes and autophagic polymers in PCOS GCs increased significantly, while GZFLW treatment reduced the number of autophagosomes and autophagic polymers. Similarly, inhibition of miR-29b-3p and overexpression of H19 attenuated the inhibition effect of GZFLW on autophagy. These results indicated that GZFLW inhibits autophagy by regulating the H19/miR-29b-3p pathway.
Furthermore, we studied the effect of GZFLW on the activity of the H19/miR-29b-3p signaling pathway, and the results showed that inhibiting the expression of miR-29b-3p can reduce the negative regulatory effect of GZFLW on the expression of PTEN, MMP-2 and Bax proteins in PCOS GCs. In addition, the negative regulatory effect of GZFLW on the expression of PTEN, MMP-2 and Bax in PCOS GCs was further weakened by inhibiting miR-29b-3p expression while overexpressing H19. Our results confirmed that GZFLW reduced the expression of PTEN, MMP-2 and Bax, and inhibited autophagy by regulating H19/miR-29b-3p.
Conclusion
In conclusion, the results of this study indicate that GZFLW inhibits autophagy in PCOS mouse GCs. This inhibition was carried out in PCOS GCs by regulating the H19/miR-29b-3p PCOS pathway to reduce the expression of PTEN, MMP-2 and Bax in the mouse cells. Our study may provide a new perspective on the mechanism and development of PCOS whilst also providing a novel strategy for PCOS treatment.
Author contributions
Hongqiu Zhu designed the experiments. Peijuan Wu, Ying Zhu, Xiaodan Hu, Haiyan Chen were performed experiments and collected data. Peijuan Wu discussed the results and strategy. Junjie Li, and Hanxue Wu supervised, directed and managed the study.
Disclosure statement
The authors report there is no competing interests to declare.
Additional information
Funding
References
- Teede HJ, Misso ML, Costello MF, et al. Recommendations from the international evidence-based guideline for the assessment and management of polycystic ovary syndrome. Fertil Steril. 2018;110(3):1–8. PubMed PMID: 30033227; PubMed Central PMCID: PMCPMC6939856.
- Witchel SF, Oberfield SE, Pena AS. Polycystic ovary syndrome: Pathophysiology, presentation, and treatment with emphasis on adolescent girls. J Endocr Soc. 2019;3(8):1545–1573. PubMed PMID: 31384717; PubMed Central PMCID: PMCPMC6676075.
- Aversa A, La Vignera S, Rago R, et al. Fundamental concepts and novel aspects of polycystic ovarian syndrome: expert consensus resolutions. Front Endocrinol (Lausanne). 2020;11:516. PubMed PMID: 32849300; PubMed Central PMCID: PMCPMC7431619.
- Wang J, Wu D, Guo H, et al. Hyperandrogenemia and insulin resistance: the chief culprit of polycystic ovary syndrome. Life Sci. 2019;236:116940.
- Palomba S, Daolio J, La Sala GB. Oocyte competence in women with polycystic ovary syndrome. Trends Endocrinol Metab. 2017;28(3):186–198.
- Palomba S. Is fertility reduced in ovulatory women with polycystic ovary syndrome? An opinion paper. Hum Reprod. 2021;36(9):2421–2428. PubMed PMID: 34333641; eng.
- Brabaharan S, Veettil SK, Kaiser JE, et al. Association of hormonal contraceptive use with adverse health outcomes: an umbrella review of meta-analyses of randomized clinical trials and cohort studies. JAMA Netw Open. 2022;5(1):e2143730. PubMed PMID: 35029663; PubMed Central PMCID: PMCPMC8760614.
- Ge JJ, Wang DJ, Song W, et al. The effectiveness and safety of liraglutide in treating overweight/obese patients with polycystic ovary syndrome: a meta-analysis. J Endocrinol Invest. 2022;45(2):261–273.
- Liu J, Wu DC, Qu LH, et al. The role of mTOR in ovarian neoplasms, polycystic ovary syndrome, and ovarian aging. Clin Anat. 2018;31(6):891–898.
- Li X, Qi J, Zhu Q, et al. The role of androgen in autophagy of granulosa cells from PCOS. Gynecol Endocrinol. 2019;35(8):669–672.
- Xu B, Dai W, Liu L, et al. Metformin ameliorates polycystic ovary syndrome in a rat model by decreasing excessive autophagy in ovarian granulosa cells via the PI3K/AKT/mTOR pathway. Endocr J. 2022;69(7):863–875.
- Chen X, Tang H, Liang Y, et al. Acupuncture regulates the autophagy of ovarian granulosa cells in polycystic ovarian syndrome ovulation disorder by inhibiting the PI3K/AKT/mTOR pathway through LncMEG3. Biomed Pharmacother. 2021;144:112288.
- Di Fazio P, Rusche FD, Roth S, et al. Long non-coding RNA H19 expression correlates with autophagy process in adrenocortical carcinoma. Cancer Invest. 2022;40(3):254–267.
- Xu X, Cui L, Zhong W, et al. Autophagy-Associated lncRNAs: promising targets for neurological disease diagnosis and therapy. Neural Plast. 2020;2020:8881687. PubMed PMID: 33029125; PubMed Central PMCID: PMCPMC7528122.
- Chen Z, Wei H, Zhao X, et al. Metformin treatment alleviates polycystic ovary syndrome by decreasing the expression of MMP-2 and MMP-9 via H19/miR-29b-3p and AKT/mTOR/autophagy signaling pathways. J Cell Physiol. 2019;234(11):19964–19976.
- Song G, Zhou J, Song R, et al. Long noncoding RNA H19 regulates the therapeutic efficacy of mesenchymal stem cells in rats with severe acute pancreatitis by sponging miR-138-5p and miR-141-3p. Stem Cell Res Ther. 2020;11(1):420. PubMed PMID: 32977843; PubMed Central PMCID: PMCPMC7519546.
- Chinese Pharmacopoeia Commission. Guizhi Fuling Wan. In: Chinese pharmacopoeia commission. Chinese Pharmacopoeia; Beijing, China: China Medical Science Press. 2020. p. 1439.
- Liu M, Zhu H, Hu X, et al. Efficacy of different forms of Guizhi Fuling Wan on reproduction and metabolism in women with polycystic ovary syndrome: a protocol for a meta-analysis of randomized controlled trials. Medicine (Baltimore). 2020;99(44):e22954. PubMed PMID: 33126363; PubMed Central PMCID: PMCPMC7598780.
- Liu M, Zhu H, Zhu Y, et al. Guizhi fuling wan reduces autophagy of granulosa cell in rats with polycystic ovary syndrome via restoring the PI3K/AKT/mTOR signaling pathway. J Ethnopharmacol. 2021;270:113821.
- Zhu Y, Li Y, Liu M, et al. Guizhi Fuling Wan, Chinese herbal medicine, ameliorates insulin sensitivity in PCOS model rats with insulin resistance via remodeling intestinal homeostasis. Front Endocrinol (Lausanne). 2020;11:575. PubMed PMID: 32973686; PubMed Central PMCID: PMCPMC7482315.
- Panossian A, Wikman G. Pharmacology of Schisandra chinensis Bail.: an overview of Russian research and uses in medicine. J Ethnopharmacol. 2008;118(2):183–212.
- Balen AH, Rutherford AJ. Managing anovulatory infertility and polycystic ovary syndrome. BMJ. 2007;335(7621):663–666. PubMed PMID: 17901517; PubMed Central PMCID: PMCPMC1995495.
- Barthelmess EK, Naz RK. Polycystic ovary syndrome: current status and future perspective. Front Biosci (Elite Ed). 2014;6(1):104–119. PubMed PMID: 24389146; PubMed Central PMCID: PMCPMC4341818.
- Palomba S, de Wilde MA, Falbo A, et al. Pregnancy complications in women with polycystic ovary syndrome. Hum Reprod Update. 2015;21(5):575–592.
- Fan L, Wang C, Zhan P, et al. miR-141-3p is poorly expressed in polycystic ovary syndrome and correlates with glucose and lipid metabolism. Int J Endocrinol. 2021;2021:2022938. PubMed PMID: 34659401; PubMed Central PMCID: PMCPMC8519708.
- Sang Z, Dong S, Zhang P, et al. miR-214 ameliorates sepsis-induced acute kidney injury via PTEN/AKT/mTOR-regulated autophagy. Mol Med Rep. 2021;24(4):683. PubMed PMID: 34328194; PubMed Central PMCID: PMCPMC8365606.
- Devis-Jauregui L, Eritja N, Davis ML, et al. Autophagy in the physiological endometrium and cancer. Autophagy. 2021;17(5):1077–1095. PubMed PMID: 32401642; PubMed Central PMCID: PMCPMC8143243.