Abstract
Objective: This study aimed to find proteomic differences in endometrial receptivity between recurrent implantation failure (RIF) and recurrent implantation success (RIS).
Methods
This study was a basic proteomic analysis of endometrial biopsies from RIF and RIS women. Differentially expressed proteins between RIF and RIS were screened via isobaric tags for relative and absolute quantification (iTRAQ) and liquid chromatography coupled with tandem mass spectrometry (LC-MS/MS). Furthermore, bioinformatic analysis was launched to analyze the difference in the endometrial receptivity between RIF and RIS women. Quantitative P CR and Western blotting were used to validated the study.
Results
This study identified 625 differentially expressed proteins in the RIF women compared with RIS women. Bioinformatics analysis demonstrated that these differentially expressed proteins were enriched in ‘Wnt signaling,’ ‘Vascular smooth muscle contraction,’ ‘Ubiquitin mediated proteolysis,’ ‘Tight junction,’ or ‘TGF-beta signaling’ related processes or pathways. Quantitative PCR and Western blotting validation experiments revealed that Claudin-4, p38, PRKAB, IKB-b were unregulated, myosin-2, PKG2 were downregulated significantly in RIF.
Conclusion
This study indicated that the alternations of key signal pathways play important roles in the failure of embryo implantation, further studies are warranted to verify if targeting these molecules might be useful to improve the embryo implantation or not.
Introduction
Recurrent implantation failure (RIF) after in vitro fertilization-embryo transfer (IVF-ET) is a status that repeated clinical pregnancy failure occurs despite transfers of good quality embryos. The current accepted definition is failure to get clinical pregnancy after at least three fresh embryo or frozen embryo transfer cycles with at least four top-quality embryos in a woman under 40 years old [Citation1]. It is thought that about two-thirds of embryo implantation failures are related to endometrial factors [Citation2,Citation3]. On the contrary, there was a group of infertile women who were very likely to get pregnant. Ghosh et al. [Citation4] and his group firstly described this case and introduced the term ‘recurrent implantation success’ (RIS). Women with RIS appear to have a persistently receptivity window of implantation (WOI) [Citation5]. To understand the influence of endometrial receptivity on the embryo implantation well, the RIS women would be a good control population for studying RIF.
Recently, several studies have compared proteomics of the human endometrium obtained from different menstrual cycle [Citation6–11]. However, most of these previous studies compared the proteome of the human endometrium between the pre-receptive phase and the receptive phase in the same women, and few reports focused on the differentially expressed proteins during WOI between RIF and RIS. Besides, the most widely used methods including 2-DE or 2D-DIGE technology have some limitations. So far, the specific molecular mechanisms associated with RIF remain unclear.
Modern analysis method is important for elucidating disease mechanisms. Proteomics, followed LC-MS/MS is a useful tool for detecting protein changes in RIF and RIS samples. Isobaric tags for relative and absolute quantitation (iTRAQ) is an MS-based proteomics method that makes it easy to compare protein expression among multiple samples, providing accurate changes in protein quantity [Citation12]. Until now, there are few relative studies using iTRAQ to identify the differentially expressed proteins associated with endometrial receptivity.
In this study, iTRAQ combined with LC-MS/MS techniques were used to explore the differential proteins and possible pathways in the endometrium samples already diagnosed as RIF or RIS.
Methods
Subject selection
Women between 25 to 35 years with regular monthly menses were included. Women included in the RIF group were primary infertility, and had at least 3 consecutive IVF attempts with at least 4 good quality embryos transfer. Women included in the RIS group should had at least one live birth, and obtained successful implantation every time after embryo transfer. Women with PCOS, endometriosis, uterine fibroids, intrauterine adhesion, and endometrial polyp were excluded. This prospective study was approved by the Institute Ethics Committee of Xiangya Hospital, Central South University. All methods were carried out in accordance with relevant guidelines and regulations. Written informed consent was obtained from all couples.
Sample collection
Patients included received HRT. During HRT cycle, endometrial biopsy was taken 5 days after progesterone treatment. First, patients were primed with 6 mg estradiol valerate a day for at least 12 days. When the endometrial thickness was more than 7 mm, 600 mg Progesterone (P) was administrated.
Endometrial biopsy was performed under sterile conditions using a pipelle catheters, and sample was rinsed with PBS and immediately frozen at −80 °C for protein extraction.
Protein preparation
Proteomics analysis was performed in four infertile women with RIF and 4 women with RIS. Each sample (50 mg) was fully cracked in lysis buffer containing 1% protease inhibitor mixture, and total proteins was extract by ultra-sonication. Centrifugation was performed at 14,000 g for 45 min at 4 °C. Then the supernatant was collected and the protein concentration was determined by BCA method. Protein (200 μg) was reduced with 100 mM DTT for 5 min at 100 °C, and then alkylated with 50 mM IAA for 30 min in darkness. All samples were digested with trypsin at 37 °C for 16-18h and reconstituted with 40 μl dissolved buffer. The samples were labeled according to iTRAQ reagent kit precedures.
LC-MS/MS analysis
The labeled samples were separated by Easy nLC1000 online reversed-phase chromatography. The peptides were automatically loaded into a C18 trap column and eluted to a C18 analytical column for 120 min at a gradient of 250nL/min. LC-MS/MS was performed by Q-Exactive mass spectrometer. The process was performed in positive ion mode, using MS1 measurement scan (m/z: 300-1800) with a resolution of 70,000, followed by 10 high-energy collision dissociation (HCD) type MS2 scan with a resolution of 17,500.
Protein identification
The MS original files were processed by Proteome Discoverer 1.3 software. Database retrieval was conducted with Mascot 2.2 against the UniProt database. The Mascot search use the following parameters: two missed trypsin cleavage sites, methyl carboamate fixation modification, methionine oxidation modifications, ±20ppm peptide mass tolerance, 0.1 Da fragment mass tolerance and dait database pattern. Polypeptides were identified and screened with a false detection rate (FDR) of 1%.
Bioinformatics analysis
Proteins were considered to be differentially expressed if they revealed more than 1.3-fold change (>1.3 or <0.7). Student’s t-test was used to analysis and the P-value <0.05 was believed to be significant. Heatmap visualizations of differentially expressed proteins was performed using Matrix2png program. Gene Ontology (Go) enrichment and Kyoto Encyclopedia of Genes and Genomes (KEGG) pathways were analyzed using OmicsBean, an online bioinformatics data analysis tool
Quantitative PCR
Total RNA was extracted from endometrium samples using TRIzol Reagent. cDNA was synthesized from 400 ng of total RNA using PrimeScript RT Master Mix. qPCR was performed using SYBR Premix Ex Taq on a CFX96 Real-Time PCR Detection System following the manufacturer’s protocol. 57 genes were selected as target genes, and GAPDH expression was used as internal reference. The specific primer sequences of each gene were showed in Supplemental Table 1.
Western blotting
Total protein (40 μg) was loaded onto an 8-12% sodium dodecyl sulfate-polyacrylamide gel, and then transferred to PVDF membranes. The membranes were sealed in TBST containing 5% BSA for 1h and then incubated with primary antibody against Claudin-4, p38, PRKAB, IKB-b, myosin-2, PGK2, and GAPDH at 4 °C overnight. GAPDH expression was used as internal control. After three washes, the membranes were incubated with secondary antibody for 1.5h. The antigen-antibody complexes were detected using an enhanced chemiluminescence Kit.
Statistical analysis
Continuous variables were analyzed for distribution status. Data with normal distribution were expressed as mean ± SEM. Differences between groups were determined by Student’s t-test. p values < .05 were considered statistically significant. All data analyses were carried outwith GraphPad Prism version 5.0.
Results
The protein software pilot was used to characterize the number of identifies proteins. A total of 35071 peptides, and 6290 proteins were identified via the iTRAQ process in both RIF and RIS women. Based on this result, we can further screen for differentially expressed proteins. Differential protein screening of quantitative proteomics was conducted using combined fold change (FC) with hypothetical testing. Proteins that meet both p < .05 and FC > 1.3/FC < 0.77 are considered differential proteins. According to the definition criteria of differentially expressed proteins (fold change ratio >1.3 or <0.77 and p <0.05), 425 up-regulated proteins and 200 down-regulated proteins were found in the RIF group compared with RIS group ().
Then, using heatmap, the expression levels of these 625 differentially expressed proteins (DEPs) were carefully visualized (). The protein expression patterns were stratified and clustered according to the expression ratio as a log2 scale. Each row in the color heat map represents a protein. The color bar represents the change of protein expression after logarithm analysis; Green means relatively low protein abundance, red means relatively high protein abundance, and black means roughly equal protein abundance. Our results showed that the protein expressions of the same type women are clustered together.
Figure 2. Hierarchical cluster analysis of the two groups. Samples were clustered based on the similarity of their protein expression profiles observed in log10 of the iTRAQ ratios and a dendrogram generated to indicate the relationship between the samples.
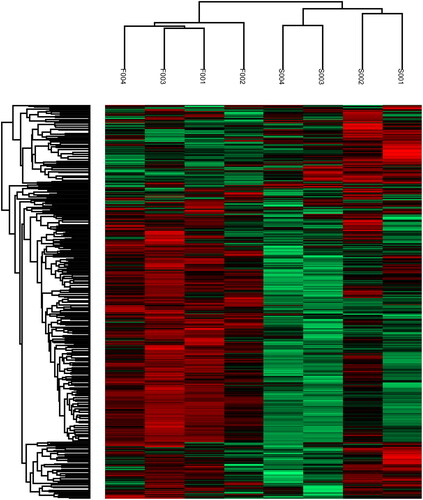
Gene Ontology (GO) enrichment analysis was conducted on the 625 differentially expressed proteins to further explore their biological functions. Based on differential changes, enrich factor and pathway correlation, and the top 30 of GO enrichment terms for up-regulated and down-regulated are showed in and , respectively.
As shown in (for up-regulated terms), biological processes accounted for 16 GO terms. The largest group was protein-DNA complex assembly, followed by nucleosome organization, chromatin assembly, protein-DNA complex assembly, regulation of RNA stability, regulation of mRNA stability, et al.; cellular components accounted for 9 GO terms, and the largest group was spliceosomal complex; molecular functions accounted for 5 GO terms (the largest group was structure-specific DNA binding). The annotated protein species were strongly enriched in the GO categories ‘ribonucleoprotein complex’ (p = 9.924e-10), ‘RNA binding’ (p = 4.339e-08), ‘poly(A) RNA binding’ (p = 4.867e-08), ‘DNA metabolic process’ (p = 7.865e-08).
As shown in (for down-regulated terms), biological processes accounted for 26 GO terms (the largest group were regulation of body fluid leves and cellular homeostasis), cellular components accounted for 2 GO terms (late endosome and endosome membrane), and molecular functions accounted for 2 GO terms (the largest group was phosphatase activity). The annotated protein species were strongly enriched in the GO categories ‘leukocyte mediated cytotoxicity’ (p = 5.771e-05), ‘cellular biogenic amine metabolic process’ (p = 2.670e-03), ‘response to reactive oxygen species’ (p = 2.790e-03), ‘late endosome’ (p = 3.242e-03), ‘leukocyte mediated immunity’ (p = 3.661e-03), and ‘organic anion transport’ (p = 3.511e-03).
In order to further study the biological functions of these proteins, we divided the up-regulated proteins into 75 KEGG classifications, and the top 30 KEGG pathways were showed in . ‘Ribosome’ is the most represented pathway, followed by ‘spliceosome,’ and ‘Base excision repair.’ Accordingly, down-regulated proteins were sub-categorized into 46 KEGG classifications, and the top 30 KEGG pathways were showed in . ‘Nitrogen metabolism’ is the most represented pathway.
Q-PCR and Western blotting validation
58 mRNA of differential expressed proteins was validated by QPCR, and results were consistent with the proteomics results (Supplemental Table 2).
To further verify the proteomics results, we selected six proteins which may have a direct and particularly close connection to embryo implantation or endometrial receptivity for validation after scanning literatures and basing on the QPCR results. Ultimately, six proteins were enrolled for Western blotting analysis. The protein expression levels of Claudin-4, p38, PRKAB, IKB-b were significantly increased, myosin-2, PKG2 were decreased significantly in RIF group than RIS group ().
Discussion
RIF is considered to be the bottleneck affecting the progress of the assisted reproductive technology (ART). Defection of the endometrium receptivity is responsible for two-thirds of RIF. During the past decade, many molecular or proteins have been identified to have associated with endometrial receptivity [Citation13]. But the specific molecular mechanism about embryo implantation is still unclear. To further understand the mechanism of embryo implantation, the differential protein expression was found between the women with RIF and RIS using iTRAQ and LC-MS/MS technology.
Here, a ‘proteomic’ comparative analysis between ‘RIF’ and ‘RIS’ was performed with iTRAQ, allowing 8 samples one time and reducing technical differences. To the best of our knowledge, this is the first study to show differential expressed proteins in endometrium with RIF and RIS.
625 proteins were found to be differentially expressed in women with RIF. GO enrichment and KEGG pathway analysis showed that several molecular mediators or metabolic processes, such as Wnt/TGF-β/Neurotrophin/Insulin signaling pathway, ECM-receptor interaction, nitrogen/pyrimidine/purine/amine/cellular ketone metabolism and glycolysis/gluconeogenesis/pentose phosphate pathway, were disturbed in RIF women.
iTRAQ results are thought to be influenced by variety of factors, such as PCOS, endometriosis, male factor infertility. Strict inclusion/exclusion criteria were used to minimize differences in patient characteristics and infertility-related factors between women in RIF and RIS groups.
Proteomic analyses, primarily using 2D-DiGE and MALDI-TOF MS, have identified a number of protein changes between the proliferative and secretory phases or between pre-receptive and receptive endometrium.
Previous studies of endometrial proteomes at fertile and infertile endometrium, or at different stages of the menstrual cycle, have identified some key proteins involved in development of endometrial receptivity and embryo implantation, such as apolipoprotein A1 [Citation7], antithrombin III [Citation6, Citation14], annexin A4 [Citation15,Citation16], and stathmin 1 [Citation15].
In order to select key proteins, some studies used isotope-coded affinity-tag technology to compare the endometrium between proliferative and secretory-phase [Citation17]. Some studies used 2D DIGE followed by matrix- assisted laser desorption/ionization tandem tome-of –flight MS to compare endometrium between late-proliferative and mid-secretory [Citation18] or between mid-proliferative and mid-secretory phase [Citation19]. To date, several studies have used 2D DIGE MS to determine changes in the protein-expression-profile between pre-receptive and receptive endometrium [Citation15, Citation20].
Dominguez et al. [Citation15] and his co- authors showed 32 proteins differentially expressed during the endometrial transformation, and only two cytoskeleton-related proteins, ANXA2 and STMN 1, were consistently up-regulated in the two experiments they conducted. Li et al. [Citation20]identified a protein- Annexin A4, which was differentially expressed between the pre-receptive and receptive endometrium. while one proteomic analysis [Citation21] showed the endometrial stromal cell proteome with different clinical phenotypes (RIF, recurrent pregnancy loss and normal fertile). In recent years, in order to explore the noninvasive testing for ER, some studies have analyzed the different protein composition of endometrial fluid aspirate [Citation22–24].
Among proteomic techniques used for biomarker identification, iTRAQs have the advantage of relatively high throughput, providing information on peptide quantitation and identification simultaneously [Citation25]. Endometrial biopsy samples from patients with RIF or RIS can be used to identify molecules related to endometrial receptivity and thus better understand human implantation [Citation13, Citation26].
Meng et al. [Citation27] studied the endometrial receptivity in cycles with GnRH antagonist protocol, GnRH agonist protocol and natural cycles during ICSI procedures, using iTRAQ analysis, and 7 proteins were differentially expressed in women with GnRH-ant protocol. Another study [Citation9] firstly used iTRAQ technology to compare the endometrial protein expression between fertile women, IUD carriers and RIF patients. Different from our results, this study found no differences between fertile and RIF patient endometrium, suggesting that other unknown factors may be associated with idiopathic RIF.
Admittedly, this study has some limitations. First, this study did not consider embryonic factor, which was an important factor of embryo implantation failure. Second, we only analysis the possible pathway involved in endometrial receptivity based on the differently expressed proteins. We do not further explore the key proteins or pathway in this study, and do not carry out functional experiments to evaluate the mechanism of key proteins.
Conclusion
The present study showed proteomic characterization on endometrium of RIF firstly. Bioinformatics analysis indicates significant alternation of the molecular mediators or metabolism related processes following RIF. Targeting these differential proteins and signaling pathways, we need to further explore possible novel strategies for RIF.
Authors’ contributions
Jing Zhao designed the study, conducted the experiment and drafted the article. Yanping Li contributed to conception and design. Jie Hao conducted acquisition of data and analysis and interpretation of data. Bin Xu participated in the interpretation of the data and the revision of the article. All authors read and approved the final manuscript.
Abbreviations | ||
ART | = | assisted reproductive technology |
CPR | = | Clinical pregnancy rate |
COS | = | controlled ovarian stimulation |
Supplemental Material
Download Zip (1.5 MB)Disclosure statement
No potential conflict of interest was reported by the authors.
Data availability statement
The datasets generated during the current study are available in the repository.
Additional information
Funding
References
- Coughlan C, Ledger W, Wang Q, et al. Recurrent implantation failure: definition and management. Reprod Biomed Online. 2014;28(1):1–9.
- Haouzi D, Dechaud H, Assou S, et al. Insights into human endometrial receptivity from transcriptomic and proteomic data. Reprod Biomed Online. 2012;24(1):23–34.
- Polanski LT, Baumgarten M. Endometrial receptivity testing and therapy in assisted reproductive treatment. Semin Reprod Med. 2021;39(1-02):27–33.
- Ghosh S, Chattopadhyay R, Goswami S, et al. Recurrent implantation success in consecutive embryo transfer cycles: a unique case report. Syst Biol Reprod Med. 2013;59(5):285–286.
- RoyChoudhury S, Singh A, Gupta NJ, et al. Repeated implantation failure versus repeated implantation success discrimination at a metabolomic level. Hum Reprod. 2016;31(6):1265–1274.
- Wang C, Feng Y, Zhou W-J, et al. Screening and identification of endometrial proteins as novel potential biomarkers for repeated implantation failure. Peer J. 2021;9:e11009.
- Brosens JJ, Hodgetts A, Feroze-Zaidi F, et al. Proteomic analysis of endometrium from fertile and infertile patients suggests a role for apolipoprotein A-I in embryo implantation failure and endometriosis. Mol Hum Reprod. 2010;16(4):273–285.
- Evans J, Hutchison J, Salamonsen LA, et al. Proteomic insights into endometrial receptivity and embryo-endometrial epithelium interaction for implantation; critical determinants of fertility. Proteomics. 2020;20(1):e1900250.
- Pérez-Debén S, Bellver J, Alamá P, et al. iTRAQ comparison of proteomic profiles of endometrial receptivity. J Proteomics. 2019;203:103381.
- Garrido-Gómez T, Quiñonero A, Antúnez O, et al. Deciphering the proteomic signature of human endometrial receptivity. Hum Reprod. 2014;29(9):1957–1967.
- Guo X, Li TC, Chen X. X: the endometrial proteomic profile around the time of embryo implantation. Biol Reprod. 2021;104(1):11–26.
- Christoforou AL, Lilley KS. Isobaric tagging approaches in quantitative proteomics: the ups and downs. Anal Bioanal Chem. 2012;404(4):1029–1037.
- Hernández-Vargas P, Muñoz M, Domínguez F. Identifying biomarkers for predicting successful embryo implantation: applying single to multi-OMICs to improve reproductive outcomes. Hum Reprod Update. 2020;26(2):264–301.
- Hannan NJ, Stephens AN, Rainczuk A, et al. 2D-DiGE analysis of the human endometrial secretome reveals differences between receptive and nonreceptive states in fertile and infertile women. J Proteome Res. 2010;9(12):6256–6264.
- Domínguez F, Garrido-Gómez T, López JA, et al. Simón. C: proteomic analysis of the human receptive versus non-receptive endometrium using differential in-gel electrophoresis and MALDI-MS unveils stathmin I and annexin A2 as differentially regulated. Hum Reprod. 2009;24(10):2607–2617.
- Li J, Tan Z, Li M, et al. Proteomic analysis of endometrium in fertile women during the prereceptive and receptive phases after luteinizing hormone surge. Fertil Steril. 2011;95(3):1161–1163.
- DeSouza L, Diehl G, Yang ECC, et al. Proteomic analysis of the proliferative and secretory phase of the human endometrium: protein identification and differential protein expression. Proteomics. 2005;5(1):270–281.
- Parmar T, Gadkar-Sable S, Savardekar L, et al. Protein profiling of human endometrial tissues in the midsecretory and proliferative phases of the menstrual cycle. Fertil Steril. 2009;92(3):1091–1103.
- Chen JIC, Hannan NJ, Mak Y, et al. Proteomic characterization of midproliferative and midsecretory human endometrium. J Proteome Res. 2009;8(4):2032–2044.
- Li J, Tan Z, Li MT, et al. Z: study of altered expression of annexin IV and human endometrial receptivity. Zhonghua Fu Chan Ke Za Zhi. 2006;41:803–805.
- Dhaenens L, Lierman S, De Clerck L, et al. Endometrial stromal cell proteome mapping in repeated implantation failure and recurrent pregnancy loss cases and fertile women. Reprod Biomed Online. 2019;38(3):442–454.
- Azkargorta M, Escobes I, Iloro I, et al. Matorras. R: differential proteomic analysis of endometrial fluid suggests increased inflammation and impaired glucose metabolism in non-implantative IVF cycles and pinpoints PYGB as a putative implantationn marker. Hum Reprod. 2018;33(10):1898–1906.
- Kasvandik S, Saarma M, Kaart T, et al. Uterine fluid proteins for minimally invasive assessment of endometrial receptivity. J Clin Endocrinol Metab. 2019;105(1):gz019.
- Giacomini E, Scotti GM, Vanni VS, et al. Global transcriptomic changes occur in uterine fluid-derived extracellular vesicles during the endometrial window for embryo implantation. Hum Reprod. 2021;36(8):2249–2274.
- Rehman I, Evans CA, Glen A, et al. iTRAQ identification of candidate serun biomarkers associated with metastatic progression of human prostate cancer. PLoS One. 2012;7(2):e30885.
- Achache H, Revel A. Endometrial receptivity markers, the journal to successful embryo implantation. Hum Reprod Update. 2006;12(6):731–746.
- Meng Y, Guo Y, Qian Y, et al. Effect of GnRH antagonist on endometrial protein profiles in the window of implantation. Proteomics. 2014;14(20):2350–2359.