Abstract
Aims: To explore whether blastocyst morphology [blastocyst stage, inner cell mass (ICM), and trophectoderm (TE) grading] impacts the occurrence of monozygotic twinning (MZT) after single blastocyst transfer (SBT).Materials and methods: A single-center retrospective cohort study was conducted including all clinical pregnancies after single blastocyst transfer between January 2015 and September 2021 (n = 9229). Blastocyst morphology was assessed using Gardner grading system. MZT was defined as more than one gestational sac (GS), or two or more fetal heartbeats in a single GS via ultrasound at 5–6 gestational weeks.Results: The overall MZT rate was 2.46% (227 of 9229 cases), of which was the highest in blastocysts of grade A TE and lowest in those with grade C TE (grade A: B:C = 3.40%:2.67%:1.58%, p = .002). Higher risk of MZT pregnancy was associated with higher trophectoderm grading [A vs. C: aOR, 1.883, 95% CI 1.069–3.315, p = .028; B vs C: aOR, 1.559, 95% CI 1.066–2.279, p = .022], but not extended culture in vitro (day 5 vs. day 6), vitrification (fresh vs. frozen-thawed ET), assisted hatching (AH), blastocyst stage (stage 1–6) or ICM grading (A vs. B).Conclusions: We conclude that TE grade is an independent risk factor of MZT after single blastocyst transfer. Blastocysts with high-grade trophectoderm are more liable to obtain monozygotic multiple gestation.
Introduction
Multiple pregnancy is considered as a suboptimal outcome associated with infertility treatment, with the increase of adverse maternal and perinatal outcomes, such as preterm birth, low and very low birthweight, stillbirth, and perinatal mortality [Citation1], and mainly resulting from the manipulation of multiple embryo transfer. Monozygotic twinning (MZT) is caused by single embryo splitting, with a much higher risk than singleton and dizygotic pregnancies, such as twin–twin transfusion syndrome, twin anemia–polycythemia, and selective intrauterine growth restriction, particularly in monochorionic twins. MZT accounts for 1.36–2.69% births after in vitro fertilization (IVF) and intracytoplasmic sperm injection (ICSI), which was much higher than that of 0.40–0.45% in natural conception births [Citation2]. Over the past two decades, reproductive physicians have been working to reduce multiple pregnancies associated with assisted reproductive technology treatment [Citation3]. Multiple gestation rates have significantly reduced since elective single embryo transfer (eSET) was proposed and advocated in many IVF centers worldwide [Citation4–7]. Nonetheless, MZT caused by early embryo splitting cannot be prevented by this strategy [Citation8–10], which have aroused considerable clinical attention. Prior studies hypothesized that the increased MZT rate after IVF/ICSI might be related to IVF laboratory procedures themselves [Citation11–15], including in vitro extended culture [Citation16], embryo cryopreservation [Citation10], and zona pellucida manipulations [Citation17,Citation18], such as ICSI, assisted hatching (AH), and embryo biopsy for preimplantation genetic testing (PGT). Although results among these heterogeneous reports were inconsistent and the mechanisms of embryo splitting are still unclear, extended embryo culture (blastocyst culture) was identified as a robust risk factor of embryo splitting [Citation2,Citation19].
Visual morphology-based selection for transfer is a currently common method with evaluation of blastocyst quality and prediction of pregnancy and live birth, but whether blastocyst morphology parameters have an impact on the occurrence of MZT after single blastocyst transfer (SBT) is still unclear.
Otsuki et al. [Citation20] conducted an observation by time-lapse photography and proposed a hypothesis that the splitting susceptibility of inner cell mass (ICM) could be associated with arrangement features of ICM cells. They suggested that ICM with loosely grouped cells might be more susceptive to splitting than tightly packed counterparts. This hypothesis begun to link the inherent morphological characteristics of blastocyst to embryo splitting. The related reports [Citation21,Citation22] showed differences in the role of ICM and trophectoderm (TE) arrangement features in the occurrence of MZT after IVF/ICSI. Therefore, a retrospective cohort study including numerous SBT cycles was conducted to explore the association of inherent blastocyst morphological characteristics (blastocyst stage, grade ICM, and TE) scored by Gardner grading system with the incidence of MZT after IVF/ICSI. This investigation may provide a novel blastocyst transfer guidance for limiting the risk of MZT after SBT.
Materials and methods
Study design and population
All clinical pregnancies (n = 9229) after SBT during January 2015 to September 2021 in the Reproductive Medical Center of The First Affiliated Hospital of Zhengzhou University were retrospectively included in this study. Only the first embryo transfer (ET) cycles with single blastocyst (PGT cycles were excluded) were included. Anonymous data were extracted from Clinical Reproductive Medicine Management System/Electronic Medical Record Cohort Database (CCRM/EMRCD) of the center. Ethical approval of this retrospective study was obtained by the institutional review board of our hospital.
Demographic characteristics of patients and parameters of blastocysts were extracted from our CCRM/EMRCD, including maternal age, body mass index (BMI), basal follicular stimulation hormone (FSH), type of cycles (fresh or frozen-thawed ET), ovarian stimulation procedures and insemination type (conventional IVF or ICSI, only in fresh cycles), assisted hatching (AH: yes or no), extended culture in vitro (day 5 or day 6), blastocyst stage, ICM grading, and TE grading, endometrium preparation protocols [natural cycle (NC) or hormone replacement therapy (HRT), only of frozen-thawed cycles], and number of gestational sac (GS) and fetal heartbeat (FHB) via ultrasound during the first trimester.
Laboratory protocols and blastocyst morphology parameters
The specific details of IVF/ICSI protocol and manipulation including insemination, embryo culture in vitro, vitrification and warming, endometrium preparation protocols of frozen-thawed embryo transfer (FET) have been described as published from our center [Citation23]. Laser-AH was performed in FET, previous implantation failure, and women older than 35 years of fresh cycles.
Blastocyst morphological parameters including blastocyst stage, ICM grade, and TE grade were evaluated using the Gardner scoring system [Citation24]. Briefly, blastocyst stage was classified from stage 1 to 6 according to blastocoel volume, blastocyst expansion degree, and hatching status (stage 1: Blastocoel cavity less than half the volume of the embryo. Stage 2: Blastocoel cavity more than half the volume of the embryo. Stage 3: Full blastocyst, cavity completely filling the embryo. Stage 4: Expanded blastocyst, cavity larger than the embryo, with thinning of the shell. Stage 5: Hatching out of the shell. Stage 6: Hatched out of the shell.). Grade of ICM and TE was scored as ‘A, B, or C’ considering the cell number and the degree of junction. ICM grading is defined as Grade A: Many cells, tightly packed. Grade B: Several cells, loosely grouped. Grade C: Very few cells. TE grading is defined as Grade A: Many cells, forming a cohesive layer. Grade B: Few cells, forming a loose epithelium. Grade C: Very few large cells. With grade C as a reference, ICM and TE grading A and B were regarded as higher grade. Only blastocyst with ICM grade of A or B was selected for transfer in our center.
Study outcomes
Biochemical pregnancy was identified by the increasing level of serum β-human chorionic gonadotropin (hCG) 14 and 18 days after ET. Clinical intrauterine pregnancies were confirmed when at least one intrauterine GS was seen via ultrasound scan 5–6 weeks after SBT, with or without FHB. MZT pregnancy was identified according to the number of GS and FHB seen via ultrasound. Those with the presence of two or more GSs, or more than one FHB in single GS were defined as MZT, and another ultrasound was performed at 7–8 weeks for confirmation, while the remaining were singleton groups.
Statistical analysis
All statistics analyses were performed using SPSS Statistics (IBM, version 26.0). Mean ± standard deviation (SD) was used to express continuous data, and differences were compared by the student’s t test. Categorical variables were presented as numbers with percentage and compared using Chi-squared test or the Fisher exact test. Multivariate logistic regression model was constructed to analyze the association between variables and the occurrence of MZT after SBT. p values (two-tailed) <.05 were considered statistically significant.
Results
Demographics and blastocyst characteristics of study subjects
Out of 9229 clinical pregnancies after SBT included in this study, 227 were defined as MZT pregnancy, including 149 pregnancies with monochorionic twins, and 5 pregnancies with monochorionic triplets, 72 pregnancies with 2 GSs, and 1 pregnancy with 3 GSs. The brief flow chart is shown in . The overall MZT rate was 2.46%, while the remaining 9002 pregnancies with 1 GS and less than 2 FHBs were singleton as controls. Demographic and embryo characteristics are compared in . The incidence of MZT was significantly higher in in vitro culture time of day 5 (day 5 vs. day 6: 2.60% vs. 1.65%; p = .040), grade A of ICM (grade A vs. B: 2.97% vs. 2.24%; p = .038), and grade A of TE (grades A:B:C = 3.40%:2.67%:1.58%; p = .002). Other parameters, including maternal age, BMI, basal FSH, ovarian stimulation protocol (only fresh-ET cycles), endometrium preparation protocol (only FET cycles), AH, the types of ET, and blastocyst expansion stage, showed no significant difference between MZT and singleton groups.
Figure 1. Flowchart of the retrospective cohort study. Note: SBT = single blastocyst transfer; GS = gestational sac; FHB = fetal heartbeat; No. = number of.; IVF = in vitro fertilization; ICSI = intracytoplasmic sperm injection; ET = embryo transfer; PGD = preimplantation genetic diagnosis.
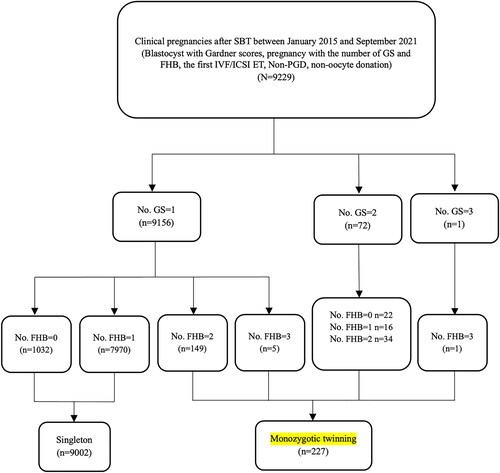
Table 1. Demographic characteristics of patients and embryos in the MZT and singleton cohorts of single blastocyst transfer cycles.
Blastocyst morphological parameters and monozygotic twinning
Multivariate logistics regression analysis model between blastocyst morphological characteristics and the occurrence of MZT after SBT is shown in . In this model, there was no significant association between in vitro extended culture time (day 5 vs. day 6), type of ET (fresh vs. frozen-thawed ET), AH (yes vs. no), blastocyst expansion stage, ICM grading (A vs. B), and MZT. Alternatively, only TE grading was a significant independent factor related to the risk of MZT after SBT. Higher TE grading was associated with higher risk of MZT (A vs. C: aOR 1.883, 95% CI 1.069–3.315, p = .028; B vs. C: aOR 1.559, 95% CI 1.066–2.279, p = .022) (). Grade A TE exhibited the highest occurrence of MZT (3.40%), while the lowest was in grade C TE (1.58%) ().
Figure 2. Key factors affecting the frequency of MZT. ‘aOR’ indicates that the odds ratio (or) and 95% CI were adjusted for potential confounding factors. Note: MZT = monozygotic twinning; ICM = inner cell mass; TE = trophectoderm; AH = assisted hatching.
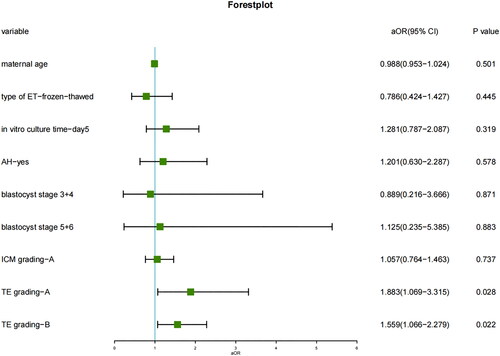
Figure 3. MZT rate of different ICM and TE grading of blastocysts based on the Gardner scoring system. Note: ICM = inner cell mass; TE = trophectoderm; MZT = monozygotic twinning.
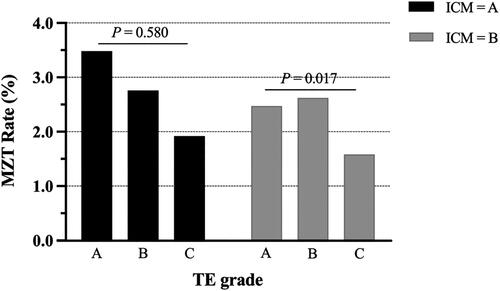
Table 2. Multivariate analysis of the blastocyst morphology parameters on MZT.
Discussion
In this study, the overall MZT rate was 2.46% of 9229 clinical pregnancies after single fresh and frozen-thawed blastocyst transfer in our center, which was generally in line with previous reports [Citation19]. The main finding was the association between the risk of MZT and TE grade. Blastocysts with higher grade TE are more susceptible to resulting in MZT pregnancies. However, we failed to find the relation between MZT and embryo cryopreservation (fresh vs. frozen-thawed ET), AH, and extended culture (day 5 vs. day 6), compared to prior reports [Citation12–14,Citation17]. Differences of these results might be caused by heterogeneity among these IVF centers, including the proportion of fresh and FET cycles, blastocyst quality, and IVF-related laboratory manipulations, such as indications of AH and culture media.
Previous study [Citation25] found that the incidence of MZT did not increase after transfer at blastocyst stage compared with cleavage stage ET when controlling the quality of embryo cohort, suggesting embryo characteristics might contribute to MZT rates. Prior investigations on blastocyst morphology were mainly conducted to evaluate the quality and their predictive value for clinical outcomes. Otsuki et al. [Citation20] observed 71 transferred frozen-thawed blastocysts by time-lapse observations and originally noted the hypothesis that compared with a blastocyst with tightly packed ICM cells, loosely grouped one was more conductive to splitting. Since then, the inner link of inherent blastocyst features and embryo splitting began to be discussed.
In the study examining the effects of hyaluronan-enriched transfer medium on MZT pregnancies after single frozen embryo transfer [Citation26], no association was observed between embryo expansion, TE, ICM grade, and the risk of MZT, which contradicted our conclusions. All MZT embryos underwent PGT and part of them were cultured in a hyaluronan-enriched transfer medium, whereas PGT cycles were excluded in our cohorts. While Xu et al.’s study with 2863 pregnancies indicated that high TE grade was a significant independent factor affecting the occurrence of MZT (A vs. C: aOR 5.46, 95% CI 1.48–20.16, p = .011; B vs C: aOR 3.96, 95% CI 1.17–13.40, p = .027) after frozen-thawed SBT, and no association was found bewteen ICM grade and MZT [Citation22]. Combining the differences in serum hCG after ET between the MZT and singleton, they hypothesized that higher grade TE which was thought to be more well-developed might be associated with monozygotic splitting, potentially by increasing secretion of hCG. Our results agreed with their findings.
Meanwhile, in the study including 26,254 clinical pregnancies after SBT from four centers conducted by Shi et al. [Citation21], 402 pregnancies with sex concordance at birth were identified as MZT. Blastocysts with grade A ICM and grade B or C TE showed the lowest MZT rate, whereas counterparts with grade B or C ICM and grade A TE presented the highest. Blastocysts with lower grade ICM (B or C) and higher-grade TE (A) were with higher risk of MZT (aOR 2.62, 95% CI 1.60–4.43). Based on Otsuki’s theory [Citation20], they proposed an extended hypothesis which was that when loosely packed ICM cells might be more susceptible to splitting, higher grade TE with more number tightly arranged cells would provide further support in the development of splitting ICM resulting in MZT pregnancies.
Influenced by prior studies about the predictive power of blastocyst morphology for clinical outcomes [Citation27–29], blastocysts with ICM score “C” are not routinely selected to transfer in our center for the higher efficiency of fertility treatment. Thus, our cohort included SBT cycles only with A and B grade ICM. Only TE grade was found to be associated with MZT, but not ICM grade, which is inconsistent with previous studies, probably caused by the differences in the definition of MZT pregnancy, laboratory techniques, and the selection of embryo transfer strategies among different IVF centers. Our results were consistent with the conclusions about the role of TE grading in increasing MZT rate after IVF/ICSI of above reports considering blastocyst morphology, and no association bewteen ICM morphology and MZT was observed. Of note, MZT gestation caused by embryo splitting must be a sophisticated and precise process, and many unknown factors might be responsible for the underlying mechanisms of embryo splitting, such as IVF laboratory parameters, embryonic characteristics, and genetic susceptibility [Citation30]. In our data, factors related to the susceptibility of ICM division were not found, and further scientific research is needed to explore the specific mechanism of ICM splitting.
The large sample size including 9229 clinical pregnancies after SBT from a single center is the main strength of this study. Although the assessment of blastocyst morphology based on Gardner scoring system was indeed influenced by subjectivity, our data was collected from a single center and evaluated by experienced embryologists followed by standard operating procedure, which has much reduced information bias. Currently, there is a lack of more objective criteria for choice. However, with the application of Time-lapse and Image Acquisition System, the scoring of embryo morphology will be less subjective in the further studies. Our study also has some limitations. First, the causal relationship between high TE grade and embryo splitting could not be scientifically investigated because of its observational nature, and the possibly underlying mechanism is still enigmatic. And not all possible confounding factors could be fully collected and controlled for its retrospective design. Although no significant associations were observed between MZT and some blastocyst characteristics, such as in vitro culture time and ICM grade, it could not exclude their possible role on pathophysiologic mechanisms behind the increased risk of embryo splitting for their uneven distribution between the singleton and MZT group. Second, data about ovarian stimulation procedures and fertilization methods of frozen-thawed cycles was not recorded in our current CCRM/EMRCD. Therefore, the association between insemination methods and MZT occurrence was not analyzed in this study. Third, MZT pregnancy was mainly defined according to the presence of GSs and FHBs via ultrasound, but not confirmed with sex concordance at birth and the gold criteria of DNA profiling for zygosity, which might overlook the possibility of a concurrent natural conception, although this is a rare event. Although the results could hardly influence clinical conduct to avoid the incidence of MZT, more information might be provided for clinical consults on MZT pregnancy for patients undergoing single blastocyst transfer. In addition, grading of embryos is a subjective, observational assessment made by the embryologist based upon experience and training. The ICM or TE scoring in our center can be different from that from others. Findings from our study should be interpreted with caution.
Conclusion
In this study, we retrospectively investigated the association between inherent blastocyst morphological characteristics and risk of MZT occurrence after single blastocyst transfer. The results indicated that higher risk of MZT is associated with blastocysts with tightly packed trophectoderm cells, which may provide guidance of selection and order of blastocyst transfer to reduce the risk of MZT.
Abbreviations | ||
MZT | = | monozygotic twinning |
SBT | = | single blastocyst transfer |
ICM | = | inner cell mass |
TE | = | trophectoderm |
ET | = | embryo transfer |
GS | = | gestational sac |
FHB | = | fetal heartbeat |
AH | = | assisted hatching |
IVF | = | in vitro fertilization |
ICSI | = | intracytoplasmic sperm injection |
eSET | = | elective single embryo transfer |
PGT | = | preimplantation genetic testing |
BMI | = | body mass index |
FSH | = | follicular stimulation hormone |
GnRH | = | gonadotropin-releasing-hormone |
hCG | = | human chorionic gonadotropin |
Acknowledgments
The authors are sincerely grateful to the staff of Center for Reproductive Medicine, the First Affiliated Hospital of Zhengzhou University, and all patients for their selfless participants. An early version of this work has been posted as a preprint on Research Square platform (https://doi.org/10.21203/rs.3.rs-1512842/v1).
Disclosure statement
The authors report no financial or commercial conflicts of interest.
Additional information
Funding
References
- Hall JG. Twinning. Lancet. 2003;362(9385):1–6. doi: 10.1016/S0140-6736(03)14237-7.
- Hviid KVR, Malchau SS, Pinborg A, et al. Determinants of monozygotic twinning in ART: a systematic review and a meta-analysis. Hum Reprod Update. 2018;24(4):468–483. doi: 10.1093/humupd/dmy006.
- Multiple gestation associated with infertility therapy: a committee opinion. Fertil Steril. 2022;117(3):498–511. doi: 10.1016/j.fertnstert.2021.12.016.
- Farquhar C. Avoiding multiple pregnancies in assisted reproductive technologies: transferring one embryo at a time should be the norm. Fertil Steril. 2020;114(4):671–672. doi: 10.1016/j.fertnstert.2020.08.1404.
- Chambers GM, Keller E, Choi S, et al. Funding and public reporting strategies for reducing multiple pregnancy from fertility treatments. Fertil Steril. 2020;114(4):715–721. doi: 10.1016/j.fertnstert.2020.08.1405.
- Mancuso AC, Boulet SL, Duran E, et al. Elective single embryo transfer in women less than age 38 years reduces multiple birth rates, but not live birth rates, in United States fertility clinics. Fertil Steril. 2016;106(5):1107–1114. doi: 10.1016/j.fertnstert.2016.06.017.
- Kanter JR, Boulet SL, Kawwass JF, et al. Trends and correlates of monozygotic twinning after single embryo transfer. Obstet Gynecol. 2015;125(1):111–117. doi: 10.1097/AOG.0000000000000579.
- Vega M, Zaghi S, Buyuk E, et al. Not all twins are monozygotic after elective single embryo transfer: analysis of 32,600 elective single embryo transfer cycles as reported to the society for assisted reproductive technology. Fertil Steril. 2018;109(1):118–122. doi: 10.1016/j.fertnstert.2017.10.003.
- Noli L, Ogilvie C, Khalaf Y, et al. Potential of human twin embryos generated by embryo splitting in assisted reproduction and research. Hum Reprod Update. 2017;23(2):156–165.
- Osianlis T, Rombauts L, Gabbe M, et al. Incidence and zygosity of twin births following transfers using a single fresh or frozen embryo. Hum Reprod. 2014;29(7):1438–1443. doi: 10.1093/humrep/deu064.
- Mateizel I, Santos-Ribeiro S, Done E, et al. Do ARTs affect the incidence of monozygotic twinning? Hum Reprod. 2016;31(11):2435–2441. doi: 10.1093/humrep/dew216.
- Ikemoto Y, Kuroda K, Ochiai A, et al. Prevalence and risk factors of zygotic splitting after 937 848 single embryo transfer cycles. Hum Reprod. 2018;33(11):1984–1991. doi: 10.1093/humrep/dey294.
- Liu X, Li P, Shi J. Double trouble? Impact of frozen embryo transfer on the monozygotic twinning rate: a retrospective cohort study from 8459 cycles. J Assist Reprod Genet. 2020;37(12):3051–3056. doi: 10.1007/s10815-020-01985-2.
- MacKenna A, Schwarze JE, Crosby J, et al. Factors associated with embryo splitting and clinical outcome of monozygotic twins in pregnancies after IVF and ICSI. Hum Reprod Open. 2020;2020(1):hoaa024.
- Vaughan DA, Seidler EA, Murphy LA, et al. Double trouble? Clinic-specific risk factors for monozygotic twinning. Fertil Steril. 2020;114(3):587–594. doi: 10.1016/j.fertnstert.2020.04.010.
- Kawachiya S, Bodri D, Shimada N, et al. Blastocyst culture is associated with an elevated incidence of monozygotic twinning after single embryo transfer. Fertil Steril. 2011;95(6):2140–2142. doi: 10.1016/j.fertnstert.2010.12.018.
- Liu H, Liu J, Chen S, et al. Elevated incidence of monozygotic twinning is associated with extended embryo culture, but not with zona pellucida manipulation or freeze-thaw procedure. Fertil Steril. 2018;109(6):1044–1050. doi: 10.1016/j.fertnstert.2018.01.040.
- Kamath MS, Antonisamy B, Sunkara SK. Zygotic splitting following embryo biopsy: a cohort study of 207 697 single-embryo transfers following IVF treatment. BJOG. 2020;127(5):562–569. doi: 10.1111/1471-0528.16045.
- Busnelli A, Dallagiovanna C, Reschini M, et al. Risk factors for monozygotic twinning after in vitro fertilization: a systematic review and meta-analysis. Fertil Steril. 2019;111(2):302–317. doi: 10.1016/j.fertnstert.2018.10.025.
- Otsuki J, Iwasaki T, Katada Y, et al. Grade and looseness of the inner cell mass may lead to the development of monochorionic diamniotic twins. Fertil Steril. 2016;106(3):640–644. doi: 10.1016/j.fertnstert.2016.05.007.
- Shi W, Jin L, Liu J, et al. Blastocyst morphology is associated with the incidence of monozygotic twinning in assisted reproductive technology. Am J Obstet Gynecol. 2021;225(6):654.e1–654.e16. doi: 10.1016/j.ajog.2021.06.101.
- Xu S, Zheng Q, Mo M, et al. High grade trophectoderm is associated with monozygotic twinning in frozen-thawed single blastocyst transfer. Arch Gynecol Obstet. 2021;304(1):271–277. doi: 10.1007/s00404-020-05928-1.
- Yang X, Bu Z, Hu L. Live birth rate of Frozen-Thawed single blastocyst transfer after 6 or 7 days of progesterone administration in hormone replacement therapy cycles: a propensity Score-Matched cohort study. Front Endocrinol (Lausanne). 2021;12:706427. doi: 10.3389/fendo.2021.706427.
- Gardner DK, Lane M, Stevens J, et al. Blastocyst score affects implantation and pregnancy outcome: towards a single blastocyst transfer. Fertil Steril. 2000;73(6):1155–1158. doi: 10.1016/s0015-0282(00)00518-5.
- Franasiak JM, Dondik Y, Molinaro TA, et al. Blastocyst transfer is not associated with increased rates of monozygotic twins when controlling for embryo cohort quality. Fertil Steril. 2015;103(1):95–100. doi: 10.1016/j.fertnstert.2014.10.013.
- Kelly AG, Blakemore JK, McCaffrey C, et al. Evaluation of clinical parameters as predictors of monozygotic twins after single frozen embryo transfer. F S Rep. 2021;2(4):428–432. doi: 10.1016/j.xfre.2021.07.001.
- Irani M, Reichman D, Robles A, et al. Morphologic grading of euploid blastocysts influences implantation and ongoing pregnancy rates. Fertil Steril. 2017;107(3):664–670. doi: 10.1016/j.fertnstert.2016.11.012.
- Shi D, Xu J, Zhang M, et al. Association between the quality of inner cell mass and first trimester miscarriage after single blastocyst transfer. Reprod Biol Endocrinol. 2020;18(1):43. doi: 10.1186/s12958-020-00595-y.
- Ross T. P–200 to transfer or to discard: a retrospective analysis of ploidy, implantation and birthweight outcomes of grade “C” blastocysts following preimplantation genetic testing for aneuploidy (PGT-A). Hum Reprod. 2021;36(Suppl_1). doi: 10.1093/humrep/deab130.199.
- Sobek A, Zbořilová B, Procházka M, et al. High incidence of monozygotic twinning after assisted reproduction is related to genetic information, but not to assisted reproduction technology itself. Fertil Steril. 2015;103(3):756–760. doi: 10.1016/j.fertnstert.2014.12.098.