Abstract
Objective
Endometriosis (EMS) is an estrogen-dependent condition with unclear pathogenesis. Recent findings suggest implicate autophagy and ferroptosis in EMS development.
Methods
We assessed autophagy and ferroptosis proteins in EMS patients using immunohistochemistry and western blot and established an EMS rat model through allograft endometrial transplantation, confirmed via hematoxylin and eosin staining and epithelial–mesenchymal transition -related proteins. Primary EMS cells were isolated from the model rats and cultured under five conditions: control, EMS, EMS with Rapamycin (autophagy inducer), EMS with si-Atg5 (autophagy inhibitor), and EMS with si-Atg5 plus Erastin (ferroptosis inducer). We evaluated cell viability, iron content, oxidative stress, and mitochondrial morphologyin EMS cells, and detected autophagy and ferroptosis proteins through immunofluorescence, western blot, and monodansylcadaverine staining.
Results
Autophagy proteins Beclin1 and LC3 were highly expressed, whereas p62, glutathione peroxidase 4, and p53 were lowly expressed in EMS patients. Rapamycin decreased cell viability but increased iron content, reactive oxygen species, lipid peroxide production, the number of ferroptotic mitochondria, and the expression of autophagy proteins in EMS cells, while si-Atg5 showed opposite effects. Additionally, Erastin reversed the impact of si-Atg5 on EMS cells.
Conclusion
Our findings suggest that autophagy-dependent ferroptosis plays a role in EMS progression.
Keywords:
Introduction
Endometriosis (EMS) is a long-lasting inflammatory condition influenced by estrogen, characterized by the occurrence of endometrial tissue beyond the uterine cavity [Citation1]. Despite its nonmalignant nature, EMS exhibits the capacity to infiltrate and invade remote tissues, mirroring the metastatic behavior of malignant tumors. The etiology, as well as pathogenesis of EMS, is not well defined, but various hypotheses have been put forward [Citation2–4]. One hypothesis posits that retrograde menstruation leads to the attachment and invasion of endometrial fragments in the peritoneal cavity, a process requiring a complex interplay between the ectopic endometrial cells and the peritoneal microenvironment. This theory is supported by the fact that retrograde menstruation occurs in most menstruating women, but only a fraction of these women develop EMS, suggesting that other factors are involved [Citation5]. Emerging evidence suggests a role of cellular and molecular mechanisms related to autophagy in the pathogenesis of EMS [Citation1,Citation3,Citation6].
Autophagy is a cellular degradation process that removes and recycles damaged proteins and organelles through the lysosomal machinery. Initiated by the formation of a phagophore, a double-membrane structure, autophagy is mediated by various Atg proteins that control the expansion of the phagophore, resulting in an autophagosome. Several Atgs are associated with autophagy, among which LC3 and Beclin1 are significant factors [Citation7]. LC3, a gene homologous to the Atg8 autophagy-related gene in yeast, is a unique marker of autophagosomes, and its expression level directly signifies the degree of autophagy [Citation8]. Beclin1 also referred to as BECN 1, is an autophagy-specific gene, a tumor suppressor gene related to autophagy, and a direct autophagy regulator in mammals [Citation9]. The autophagosome then merges with a lysosome, leading to the degradation and recycling of its content [Citation10,Citation11]. This process plays a pivotal role in maintaining cellular homeostasis. Dysregulation of autophagy, as has been suggested in the context of EMS, may promote the survival and proliferation of ectopic endometrial cells [Citation3]. EMS exhibits numerous tumor disease traits, including metastasis, implantation, and recurrence [Citation12,Citation13]. Various studies have reported a link between autophagy and several malignant tumors. For instance, a decrease in autophagy activity has been associated with the onset and progression of various cancers, including pancreatic, breast, cervical squamous cell, colon, and ovarian cancer, indicating a strong correlation between the abnormal expression of autophagy-related genes and tumor development [Citation14,Citation15].
Ferroptosis is a type of regulated cell death characterized by the iron-dependent accumulation of lethal lipid peroxides (LPOs) [Citation16]. It is controlled by the enzyme glutathione peroxidase 4 (GPX4), which reduces LPOs to prevent their accumulation [Citation17]. Ferritinophagy, a specialized form of autophagy, degrades ferritin, the primary cellular iron storage protein. The cargo receptor known as nuclear receptor coactivator 4 (NCOA4) targets ferritin for degradation in the lysosome , consequently releasing iron [Citation18]. This process enables cells to access stored iron during iron deficiency. Iron, released through ferritinophagy, contributes to ferroptosis by participating in the Fenton reaction, leading to the production of reactive oxygen species (ROS) and lipid peroxidation [Citation19]. Dysregulation of ferroptosis, which has been linked to diseases including cancer and neurodegeneration [Citation20], may also contribute to the inflammatory and oxidative stress environment observed in EMS [Citation21–23]. The peritoneal fluid of EMS patients and the local microenvironment of ectopic lesions had been described as abundant in free iron and high oxidative level; these may owe to retrograde menstruation producing iron release and ectopic lesions bleeding themselves [Citation24]. More evidence supported that the imbalance of iron homeostasis in EMS could promote ectopic lesions implant and development [Citation25].
Therefore, given these observations, in this study, in clinical, the expression of the autophagy and ferroptosis proteins in EMS patients was tested. In vivo, the EMS rat model was established by allograft endometrial transplantation. In vitro, primary EMS cells were isolated and cultured with Rapamycin (autophagy inducer), si-Atg5 (autophagy inhibitor), and Erastin (ferroptosis inducer) to observe the autophagy and ferroptosis proteins’ change in EMS cells.
Material and method
Ethics authorization
The local Ethics Committee of the first affiliated hospital of zhejiang Chinese medical university provided the ethical clearance for this research (2023-KS-486-01). Prior to any surgical procedures, a written and informed consent was obtained from every participant.
Participant and tissue sample selection
The participants were nonpregnant women (27–46 years) who attended the first affiliated hospital of zhejiang Chinese medical university. These women had regular menstrual cycles that varied between 28 and 35 days, and none had taken any form of steroid medication in the six months preceding the surgery. Experts confirmed that all endometrial tissue samples were collected during the proliferative stage, based on hematoxylin and eosin (HE) sections. The control group comprised of 10 cases, where normal endometrium tissue samples were collected. In addition, 10 cases of eutopic endometrium of EMS were gathered from patients undergoing laparoscopy for pelvic EMS. The tissue was fixed in 10% buffered formalin and made into paraffin slices for immunohistochemistry (IHC) analysis.
IHC staining
Remove paraffin by immersing slides in xylene followed by a graded alcohol series for rehydration. Heat slides in an appropriate buffer solution to unmask antigens. Incubate slides with a blocking solution to reduce nonspecific background staining. Apply the primary antibody listed in and incubate for 2 h. Rinse slides with a buffer solution to remove unbound primary antibodies. Apply a secondary antibody conjugated to a detection system and incubate. Rinse slides again to remove unbound secondary antibodies. Add a substrate or fluorophore to visualize the antigen–antibody complex. Apply a counterstain, hematoxylin, to provide a contrasting background for the stained antigen. Dehydrate slides through a graded alcohol series, clear with xylene.
Table 1. Primary antibody information.
Establishment of EMS rats and grouping
We procured 30 female nonpregnant Sprague Dawley rats, aged 8 weeks, weighing 200 g, from Shanghai SLAC Laboratory Animal Co., Ltd., under the animal production license number SCXK (Hu) 2017-0005. The rats were housed in a controlled environment with a temperature of 24 °C, 55% humidity, and a 12-h light/dark cycle at Zhejiang Eyong Pharmaceutical Research and Development Co., Ltd., under the experimental license number SYXK (Zhe) 2021-0003.
Adaptive feeding was carried out for 1 week. All rats were randomly divided into a control group (6 rats) and an EMS group (donor group: 4 rats; recipient group: 20 rats). Rats with normal estrous cycles were selected, and a rat model of EMS was established using an allogeneic heterotopic endometrial transplantation method [Citation6,Citation26]. Before modeling, valerate estradiol (1 mg/kg) was administered via gavage for 2 days to synchronize the estrous cycle. Anesthesia was induced with isoflurane inhalation, followed by iodophor disinfection. Under aseptic conditions, donor uteri were excised and cut into 3 mm × 4 mm fragments, which were sutured onto the recipient rats’ bilateral abdominal walls and around the ovarian mesentery with rich blood vessels, one fragment on each side, with the endometrial surface facing the abdominal cavity (donor:recipient = 1:5). After surgery, 10,000 IU of penicillin sodium injection was administered intraperitoneally, followed by a layer-by-layer abdominal closure and incision disinfection. The blank group was left untreated. Postoperative recipient rats received 10,000 IU of penicillin sodium injection intraperitoneally for three consecutive days to prevent infection, iodophor disinfection of the abdominal incision for 5 days, and valerate estradiol (1 mg/kg) gavage for 5 days to promote ectopic lesion growth. One week after modeling, two recipient rats were randomly selected for HE staining to examine the success of the EMS model. If the transplanted tissue volume increased, ectopic lesion fluid accumulated, transparent vesicles were observed, and adhesions of varying degrees were seen with surrounding tissues. HE staining under a light microscope revealed distinct endometrial or stromal cells and glands, which indicated successful modeling. And the graphical representation of study flowchart can be seen as Figure S1.
Sample collection
Upon successful modeling, anesthesia was induced in rats with isoflurane inhalation. The in situ endometrium of control rats and EMS group rats were separated, partly for the isolation and culture of primary cells of non-EMS in situ endometrial cells and EMS ectopic endometrial cells, and partly for the staining.
HE staining
Initiate the process by immersing the tissue sections in xylene. Subsequently, place sections in anhydrous ethanol I and II, then in 75% alcohol, before rinsing with tap water. Next, immerse the sections in HE (Sigma, H3136) for 3–5 min, rinse them with tap water, differentiate, rinse again with tap water, and restore the blue color. Afterward, rinse with running water. Continue by placing the sections sequentially in 85% and 95% gradient alcohol for dehydration, for 5 min each. Stain the section with eosin stain (Sigma, E4009) for another 5 min. Then, place the sections in anhydrous ethanol I, II, and III, each for 5 min. Follow this by placing the sections in xylene to achieve transparency. Finally, mount the sections with neutral resin. Examine the sections under a Nikon Eclipse Ci-L microscope.
Primary EMS culture
Isolate EMS according to the method described previously [Citation27,Citation28]. The EMS produced by this method was more than 95% pure, which was confirmed by staining using vimentin and E-cadherin. In summary, endometrial tissue was finely chopped (2–3 mm) and subjected to digestion in DMEM/F-1 with 12.0% type IV collagenase (Sigma). The resulting dispersed cells were then sieved through a 400-mesh wire screen to remove undigested tissue fragments. Post a gentle centrifugation, the supernatant was removed while the cells were re-suspended in DMEM/F-10 supplemented with 12% fetal bovine serum, penicillin, streptomycin, and amphotericin B. Freshly isolated EMS was allowed to culture overnight in 25 cm2 flasks. The following day, any unattached cells were rinsed away, leaving predominantly stromal cells (2–3 × 106/flask) adhered to the flask. After digestion with trypsin, the EMS was seeded into six well plates at a density of 4 × 105 cells per well.
Immunofluorescence
The EMS cells are first fixed using paraformaldehyde. The cell membrane is then permeabilized with a detergent Triton X-100 to allow antibodies to enter the cell and access intracellular antigens. The cells are incubated with bovine serum albumin to prevent nonspecific antibody binding. The primary antibody (Anti-Vimentin, Abcam, ab8978; E-cadherin, Affinity, AF0131; LC3, Affinity, AF5402), is added respectively and the cells are incubated to allow the antibody to bind to its target. Unbound primary antibodies are washed away with a buffer solution. A fluorescently labeled secondary antibody (Goat-Anti-Rabbit lgG H&L (Alexa Fluor 594), Abcam, ab150080; Goat-Anti-Mouse lgG H&L (Alexa FLIOR 594), Abcam, ab150116), which recognizes the primary antibody, is added and incubated with the cells. Unbound secondary antibodies are washed away. Imaging: fluorescent signals are then visualized and captured using a fluorescence microscope (Nikon, Ts2-FC).
Cell grouping
The cells were divided into control, EMS, EMS + Rapamycin (autophagy inducer), EMS + si-Atg5, and EMS + si-Atg5 + Erastin (ferroptosis inducer) groups. The plasmid (si-Atg5) was transfected 24 h in advance. Added 100 nM Rapamycin (S1039) purchased from Selleckchem (USA) dissolved in dimethyl sulfoxide (DMSO, Sigma–Aldrich) for 24 h according to the previous study to the EMS cells [Citation29]. And EMS cells were treated Erastin (10 μM; Sigma, 571203-78-6) for 24 h according to the study [Citation30]. After the drug treatment, cells were collected for follow-up experiments.
Cell counting kit-8
Prepare the EMS cell suspension and adjust the cell density to 25,000/mL. Inoculate cells into 96-well plates with six replicate wells in a 37 °C incubator. Remove half of the culture medium from the experimental wells and add 2× pre-prepared test drugs at different concentrations to each well. Incubate in a 37 °C incubator and assess the inhibition rate after 4 days. Add 10 μL of cell counting kit-8 (CCK8) solution (Beyotime, C0037) to each well. Incubate for 2 h in the incubator. Use a microplate reader (MD, CMaxPlus) to measure the absorbance at 450 nm and calculate cell viability. Cell survival rate (%) = (each treatment group − blank group)/(control group − blank group) × 100%.
Monodansylcadaverine staining
Digest the logarithmic growth phase cells using standard methods and seed them in six-well plates, inoculating 1 × 106 cells per well. When the EMS cell density reaches 60–70%, discard the supernatant, wash twice with PBS, and add culture medium containing monodansylcadaverine (MDC; final concentration 50 pM; Beyotime, C3018S) to each well. Incubate the plates in a 37 °C, 5% CO2 incubator, protected from light, for 20 min. Remove the six-well plate and place it under a fluorescence microscope (Nikon, Ts2-FC). Observe and photograph the occurrence of autophagy in cells within 1 h.
Enzyme-linked immunosorbent assay measurement
The level of LPO and ROS of the EMS cells were tested by the Rat (LPO) enzyme-linked immunosorbent assay (ELISA) kit (Meimian, Jiangsu, MM-0890R2) and Rat (ROS) ELISA kit (Meimian, Jiangsu, MM-21264R2) respectively under the instructions.
Iron content assay
The iron content of the EMS cells was tested by the kit (BC5310) that was provided by the Beijing Solaibao Technology Co., LTD. Use a ratio of cell number (1 × 104 cells) to extraction solution volume (mL) of 1000:1 and break the cells with ice-bath ultrasonication (power 200 W, sonication for 3 s, interval 7 s, repeat 30 times). Centrifuge and collect the supernatant for testing. Preheat the visible spectrophotometer for 30 min, adjust the wavelength to 510 nm, and zero with distilled water. Add samples to 1.5 mL Eppendorf tubes. Mix well, let the samples stand at 25 °C for 10 min for color development, and measure the absorbance at 510 nm in a 1 mL glass cuvette, recording the values.
Western blot
Isolate proteins from EMS cells by employing a lysis buffer. Measure the protein concentration using a protein assay kit (Solarbio, pc0020). Separate proteins utilizing SDS-PAGE. Transfer proteins from the gel to a PVDF membrane (GE Healthcare Life, 10600023). Block the membrane with a 5% milk solution. Incubate the membrane with a primary antibody, followed by a secondary antibody (). Identify the enzyme-linked secondary antibody with a chemiluminescent substrate. Evaluate the signal intensity to determine the protein quantity.
Table 2. Secondary antibody information.
Electron microscope imaging
Collect EMS cells and fix them in 2.5% glutaraldehyde solution for 4 h. Wash four times with 0.1 M pH 7.0 PBS, 15 min each time. Fix with 1% osmium tetroxide solution for 1–2 h, then wash three times. Dehydrate the cells using a gradient concentration of ethanol solutions (50%, 70%, 80%, 90%, and 95%), treating each concentration for 15 min, followed by 100% ethanol for 20 min, and finally treating with pure acetone for 20 min. Infiltration with gradient embedding agent. Place cells in a 0.5 mL dry centrifuge tube and heat overnight at 70 °C in a heating polymerization instrument; trim and cut sections (50–70 nm) using a Leica EM UC 7 ultramicrotome; use a plastic embedding mold, stain with 100 μL of 50% uranyl acetate saturated solution in ethanol (15 min-1 h), rinse with double distilled water, and stain with 100 μL of lead citrate for 15 min. Then use transmission biological electron microscope (TEM; Hitachi H-7650) to capture the figure.
Statistical analysis
The data analysis was performed using SPSS software (version 16.0, IBM, USA). Comparisons between the two groups were conducted using the Student’s t-test. One-way ANOVA was utilized for analyzing data with a normal distribution in this study. If the distribution was normal but the variance was not uniform, Dunnett’s T3 test or independent sample t-test was employed. A p-value of less than .05 was considered statistically significant.
Results
Expression of autophagy and ferroptosis proteins, pathology, and EMT proteins in EMS models
In , using IHC, we observed that the average of density (AOD) of the Beclin 1 and LC3 in the tissue of the endometrium was enhanced in the EMS patients than the control group (p < .05 or p < .01). On the contrary, the AOD of the GPX4 and p53 in the tissue of the endometrium was reduced in the EMS patients than the control group (p < .01). As shown in , the expression of p62 that tested by western blot was decreased in the EMS group than in the control group (p < .01). In , with HE staining, the results demonstrated that in the randomly selected recipient rats’ uterine tissues, the transplanted tissue volume increases, fluid accumulates in the ectopic lesions, transparent small vesicles can be observed, and different degrees of adhesion with surrounding tissues are present. In , the highly expressed vimentin protein and low expressed E-cadherin protein were found in EMS cells than in control cells (p < .01).
Figure 1. Expression of autophagy and ferroptosis proteins in clinical EMS patients. (A,B) Positive expression of the Beclin1, LC3, GPX4, p53, and autophagy associated proteins was tested by the IHC (magnification 200×, scale bar: 100 μm), and the representative image of each group as well as the chart was showed. n = 3 in each group. (C) Expression of p62 was evaluated by western blot assay, and GAPDH was used as the internal control. n = 3 in each group. #p < .05 and ##p < .01 vs. control group. EMS: endometriosis; GPX4: glutathione peroxidase 4; IHC: immunohistochemistry.
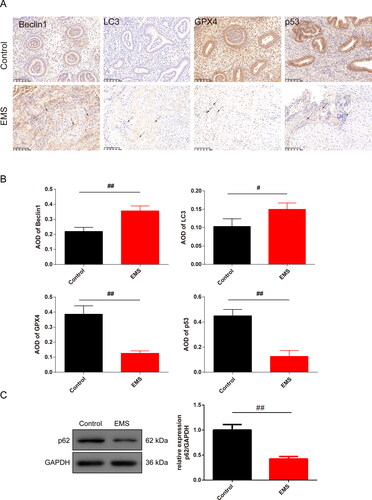
Figure 2. Pathology of EMS in rats and expression of the EMT related proteins in EMS cells. (A) HE staining was used to observe the pathology of the endometrium of the EMS rats (magnification, 40×, scale bar: 400 μm; 100×, scale bar: 200 μm). (B) Expression of the vimentin and E-cadherin was tested by IF to identify the cells that was cultured from the rats (magnification 200×, scale bar: 100 μm), and the chart was calculated. n = 3 in each group, ##p < .01 vs. control group. EMS: endometriosis; EMT: epithelial–mesenchymal transition; HE: hematoxylin and eosin; IF: immunofluorescence.
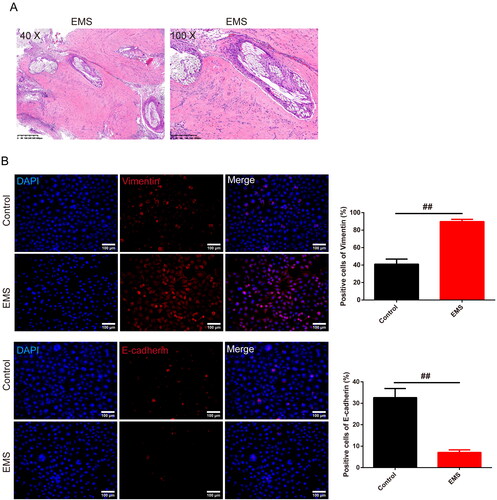
Ferroptosis closely related to autophagy in EMS cells
In , CCK8 assay and kits were used to test cell viability and the level of the iron content, ROS and LPO production of the EMS cells. We discovered that the cell viability, iron content, ROS and LPO production were higher in the EMS group than control group (p < .05 or p < .01). And the addition of Rapamycin reduced the cell viability, but si-Atg5 group reversed the trend in relative to the EMS group (p < .01). With the addition of the Erastin, the cell viability was decreased compared to the EMS + si-Atg5 group (p < .01). Furthermore, the addition of Rapamycin enhanced the iron content, ROS and LPO production while the addition si-Atg5 reduced the expression in relative to the EMS group (p < .05 or p < .01). On the basis of the EMS and si-Atg5, Erastin strengthened the iron content, ROS and LPO production (p < .01). In , TEM results showed that the number of ferroptosis mitochondria with ridge reduction or disappearance and outer membrane rupture in the EMS group was significantly increased than control group (p < .05), mitochondrial was smaller and the feature of the ferroptosis was obvious. Compared with EMS group, the EMS cell ridge decreased, the number of ferroptosis mitochondria with broken outer membrane increased in EMS + Rapamycin, EMS + si-Atg5 + Erastin group (p < .05), and mitochondrial became smaller. In the EMS + si-Atg5 group, the cell ridge was disappeared, the number of ferroptosis mitochondria broken in the outer membrane was significantly decreased (p < .05). Furthermore, the expression of NCOA4, FTH, FTMT, and HO-1 was evaluated by western blot assay (). The expression of the FTH, FTMT, HO-1 was enhanced whereas the NCOA4 was reduced in the EMS group than in the control group (p < .05 or p < .01). Compared to the EMS group, the expression of FTH, FTMT, HO-1 in the EMS + Rapamycin group was increased whereas the expression of NCOA4 was reduced. Nevertheless, the expression was opposite in the EMS + si-Atg5 group. And this effect was reversed by the Erastin addition (p < .01).
Figure 3. Ferroptosis is closely related to autophagy in EMS cells. (A) CCK8 was used to test the cell viability of each group of cells. n = 6 in each group. (B) Iron content of the cells was detected by the kit. n = 3 in each group. (C) ELISA kits were used to test the expression of the ROS and LPO of cells in each group. n = 3 in each group. (D) Transmission electron microscopy was used to observe the morphological changes of mitochondria (magnification 10.0k×, scale bar: 2 μm; magnification 25.0k×, scale bar: 500 nm) and the number of the mitochondria was recorded in the chart. n = 3 in each group. (E) Expression of NCOA4, FTH, FTMT, and HO-1 was evaluated by western blot assay, and GAPDH was used as the internal control. n = 3 in each group. #p < .05 and ##p < .01 vs. control group. @p < .05 and @@p < .01 vs. EMS group. $p < .05 and $$p < .01 vs. EMS + si-Atg5 group. EMS: endometriosis; CCK8: cell counting kit-8; HE: hematoxylin and eosin; ELISA: enzyme-linked immunosorbent assay; ROS: reactive oxygen species; LPO: lipid peroxide; NCOA4: Nuclear Receptor Coactivator 4; FTH: ferritin heavy chain; FTMT: ferritin mitochondrial; HO-1: heme oxygenase-1.
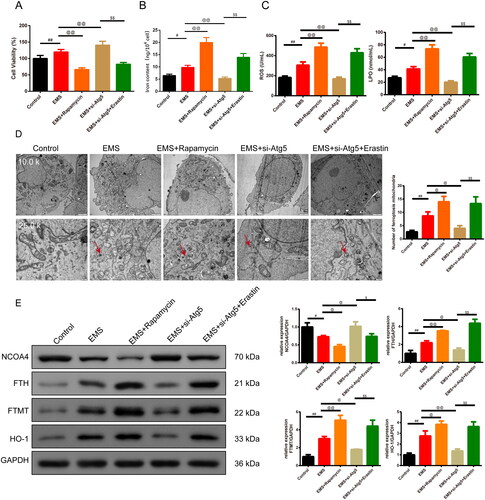
Autophagy-dependent ferroptosis involved in EMS cells
As shown in , under immunofluorescence (IF) and western blot assay, we found that the expression of the LC3, LC3 II/LC3 I ratio, and Beclin 1 was higher in the EMS group than the control group (p < .01). The addition Rapamycin enhanced the expression of the LC3, LC3 II/LC3 I ratio, and Beclin1, while si-Atg5 reduced the expression in relative to the EMS group (p < .05 or p < .01). On the basis of the EMS and si-Atg5, Erastin strengthened the LC3, LC3 II/LC3 I ratio, and Beclin1 expression (p < .01). Nevertheless, the expression of the GPX4 and p53 acted conversely (p < .05 or p < .01). In , MDC staining was used to test the autophagy particle of cells in each group. The fluorescence intensity of cells in the EMS group was extremely enhanced than control group, and aggregated fluorescent particles could be seen in the cytoplasm (p < .01). However, they were reversed in the EMS + Rapamycin and EMS + si-Atg5 + Erastin groups (p < .01). The fluorescence expression intensity of EMS + si-Atg5 group was significantly decreased than EMS group (p < .01).
Figure 4. Autophagy-dependent ferroptosis is involved in EMS cells. (A) Expression of the LC3 in cells was tested by IF (magnification 200×, scale bar: 100 μm), and the chart was calculated. n = 3 in each group. (B) Expression of the Beclin1, LC3 II/LC3 I, GPX4, and p53 was tested by western blot. n = 3 in each group. (C) MDC staining was used to test the autophagy particle of cells in each group. n = 3 in each group. ##p < 0.01 vs. control group. @p < .05 and @@p < .01 vs. EMS group. $$p < .01 vs. EMS + si-Atg5 group. EMS: endometriosis; GPX4: glutathione peroxidase 4; IF: immunofluorescence; MDC staining: monodansylcadaverine staining.
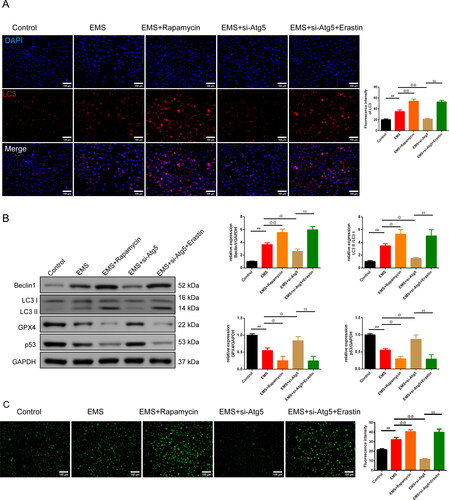
Discussion
EMS is characterized as a progressive fibrotic disorder that manifests in the uterus and the adjacent stroma. Nonetheless, the absence of comprehensive knowledge about EMS’s underlying mechanisms continues to pose significant challenges. Recently, a study reported that CTLA4 is an immune checkpoint molecule that plays a crucial role in the regulation of the immune system. It is involved in the maintenance of chronic inflammation in the peritoneal environment, which is relevant to EMS [Citation31]. From our perspective, we showcased autophagy-dependent ferroptosis is involved in the EMS cells.
To begin with, the HE staining of the EMS tissue results and the highly expressed vimentin whereas the low expressed E-cadherin in EMS culture cells indicated that the successful model of the EMS was established. The abnormal epithelial–mesenchymal transition (EMT) process is involved in the initial formation of EMS [Citation32]. Prior research indicates that hypoxia can instigate the EMT process by enhancing the expression of EMT-associated transcription factors, which leads to a more aggressive tumor phenotype and equips the tumor cells with the capability to invade far-off locations in EMS [Citation33]. Investigations have demonstrated that EMT-like procedures transpire during ovarian EMS, characterized by a decline in E-cadherin expression but an elevation in vimentin expression within epithelial cells affected by EMS lesions [Citation34]. Consistently, we observed the EMT process in EMS rats.
Subsequently, we observed the autophagy proteins change in EMS models. Earlier research has suggested that autophagy is increased in ovarian EMS, potentially aiding the survival of endometriotic cells and the persistence of lesions at ectopic locations. Consistently, in our study, we found the highly expressed Beclin1, LC3, and lowly expressed p62 in the EMS patients as well as in the EMS cells. In addition, the highly positive cells of the MDC staining indicated that the EMS promoted the autophagy. The addition of the Rapamycin and si-Atg5 proved that the EMS is closely related to the autophagy. Nonetheless, there have been conflicting findings regarding autophagy levels in EMS. A study by Choi et al. noted a decrease in autophagy in ectopic endometrium, coinciding with the activation of p70S6K phosphorylation [Citation35]. These divergent outcomes might be due to the intricate signaling networks that govern autophagy. Liu reported in their study that autophagy upregulation in EMS may due to local hypoxia and autophagy play a role in HIF-1α induced human endometrial stromal cells migration and invasion [Citation5]. Hence, we speculated that in our study, the EMS is accompanied with hypoxia and the autophagy is increased in EMS models.
Ferroptosis is a type of cell death that is dependent on autophagy [Citation22]. It can cause oxidative stress, lipid peroxidation, morphological changes of mitochondria, contributing to the pathophysiology of EMS [Citation36]. Within the ovary, hypoxia-driven elevations in ROS levels can provoke autophagy in EMS cells [Citation37]. While autophagy serves to eliminate damaged proteins and subcellular organelles to sustain cell viability, unrecoverable damage can trigger cell death in the intrafollicular microenvironment [Citation38]. Abnormal expression of iron-specific autophagy-related genes and proteins has been detected in granulosa cells of patients experiencing infertility related to EMS [Citation37]. In our study, the addition of Erastin, a ferroptosis inducer, it has been reported that it induces ferroptosis via ferroportin-mediated iron accumulation in EMS [Citation23]. Erastin inhibited the cell viability, GPX4, p53, NCOA4 expression but promoted the iron content, FTH, FTMT, ROS, HO-1, LPO production, autophagy, and the number of the ferroptosis mitochondria compared to the EMS + si-Atg5 group, indicating that the ferroptosis is closely related to the autophagy in EMS. Consistently, iron excess in the peritoneal fluid of EMS interferes with blastocyst development, lowers GPX4 expression, and triggers lipid peroxidation. This implies that iron overload contributes to embryo toxicity and promotes ferroptosis [Citation39].
In conclusion, autophagy-dependent ferroptosis is involved in the EMS cells. However, the deeper mechanism of the ferroptosis in the EMS animal model should be verified in the future study.
Conclusion
To sum up, autophagy proteins Beclin1 and LC3 were highly expressed while ferroptosis proteins GPX4 and p53 were lowly expressed in the EMS patients. The addition of Rapamycin inhibited the cell viability but promoted the iron content, ROS, LPO production, number of the ferroptosis mitochondria, and the autophagy proteins of the EMS cells, while the si-Atg5 reversed the trend. And Erastin had the opposite effect than si-Atg5. This study provided the basis for further clinical and experimental research of the potential drug therapy target to the EMS.
Supplemental Material
Download TIFF Image (498.2 KB)Disclosure statement
No potential conflict of interest was reported by the authors.
Data availability statement
All data will be available upon request.
Correction Statement
This article has been corrected with minor changes. These changes do not impact the academic content of the article.
Additional information
Funding
References
- Xu H, Gao Y, Shu Y, et al. EPHA3 enhances macrophage autophagy and apoptosis by disrupting the mTOR signaling pathway in mice with endometriosis. Biosci Rep. 2019;39(7):1. doi: 10.1042/BSR20182274.
- Kim SI, Yeo SG, Gen Y, et al. Differences in autophagy-associated mRNAs in peritoneal fluid of patients with endometriosis and gynecologic cancers. Eur J Obstet Gynecol Reprod Biol X. 2019;2:100016. doi: 10.1016/j.eurox.2019.100016.
- Yang HL, Mei J, Chang KK, et al. Autophagy in endometriosis. Am J Transl Res. 2017;9(11):4707–11.
- Zhan L, Li J, Wei B. Autophagy in endometriosis: friend or foe? Biochem Biophys Res Commun. 2018;495(1):60–63. doi: 10.1016/j.bbrc.2017.10.145.
- Liu H, Zhang Z, Xiong W, et al. Hypoxia-inducible factor-1α promotes endometrial stromal cells migration and invasion by upregulating autophagy in endometriosis. Reproduction. 2017;153(6):809–820. doi: 10.1530/REP-16-0643.
- Siracusa R, D’Amico R, Impellizzeri D, et al. Autophagy and mitophagy promotion in a rat model of endometriosis. Int J Mol Sci. 2021;22(10):5074. doi: 10.3390/ijms22105074.
- Katagiri H, Nakayama K, Razia S, et al. Loss of autophagy-related protein Beclin 1 may define poor prognosis in ovarian clear cell carcinomas. Int J Oncol. 2015;47(6):2037–2044. doi: 10.3892/ijo.2015.3191.
- Shi J, Shi S, Wu B, et al. Autophagy protein LC3 regulates the fibrosis of hypertrophic scar by controlling Bcl-xL in dermal fibroblasts. Oncotarget. 2017;8(55):93757–93770. doi: 10.18632/oncotarget.20771.
- Zhu ML, Lu JX, Pan GP, et al. Traditional Chinese medicine Ka-Sai-Ping suppresses the growths of gastric cancers via induction of autophagy. Oncotarget. 2017;8(56):95075–95082. doi: 10.18632/oncotarget.18041.
- Li X, He S, Ma B. Autophagy and autophagy-related proteins in cancer. Mol Cancer. 2020;19(1):12. doi: 10.1186/s12943-020-1138-4.
- Lamark T, Johansen T. Mechanisms of selective autophagy. Annu Rev Cell Dev Biol. 2021;37:143–169. doi: 10.1146/annurev-cellbio-120219-035530.
- Machado DE, Rodrigues-Baptista KC, Alessandra-Perini J, et al. Euterpe oleracea extract (Açaí) is a promising novel pharmacological therapeutic treatment for experimental endometriosis. PLoS One. 2016;11(11):e0166059. doi: 10.1371/journal.pone.0166059.
- Ye H, He Y, Wang J, et al. Effect of matrix metalloproteinase promoter polymorphisms on endometriosis and adenomyosis risk: evidence from a meta-analysis. J Genet. 2016;95(3):611–619. doi: 10.1007/s12041-016-0675-5.
- Lin SC, Chu PY, Liao WT, et al. Glycyrrhizic acid induces human MDA-MB-231 breast cancer cell death and autophagy via the ROS-mitochondrial pathway. Oncol Rep. 2018;39(2):703–710. doi: 10.3892/or.2017.6123.
- Peng Q, Qin J, Zhang Y, et al. Autophagy maintains the stemness of ovarian cancer stem cells by FOXA2. J Exp Clin Cancer Res. 2017;36(1):171. doi: 10.1186/s13046-017-0644-8.
- Dixon SJ, Lemberg KM, Lamprecht MR, et al. Ferroptosis: an iron-dependent form of nonapoptotic cell death. Cell. 2012;149(5):1060–1072. doi: 10.1016/j.cell.2012.03.042.
- Song X, Zhu S, Chen P, et al. AMPK-Mediated BECN1 phosphorylation promotes ferroptosis by directly blocking system X(c)(-) activity. Curr Biol. 2018;28(15):2388–2399.e5. doi: 10.1016/j.cub.2018.05.094.
- Mancias JD, Wang X, Gygi SP, et al. Quantitative proteomics identifies NCOA4 as the cargo receptor mediating ferritinophagy. Nature. 2014;509(7498):105–109. doi: 10.1038/nature13148.
- Stockwell BR, Friedmann Angeli JP, Bayir H, et al. Ferroptosis: a regulated cell death nexus linking metabolism, redox biology, and disease. Cell. 2017;171(2):273–285. doi: 10.1016/j.cell.2017.09.021.
- Hassannia B, Vandenabeele P, Vanden Berghe T. Targeting ferroptosis to iron out cancer. Cancer Cell. 2019;35(6):830–849. doi: 10.1016/j.ccell.2019.04.002.
- Sanchez AM, Viganò P, Somigliana E, et al. The distinguishing cellular and molecular features of the endometriotic ovarian cyst: from pathophysiology to the potential endometrioma-mediated damage to the ovary. Hum Reprod Update. 2014;20(2):217–230. doi: 10.1093/humupd/dmt053.
- Zhou B, Liu J, Kang R, et al. Ferroptosis is a type of autophagy-dependent cell death. Semin Cancer Biol. 2020;66:89–100. doi: 10.1016/j.semcancer.2019.03.002.
- Li Y, Zeng X, Lu D, et al. Erastin induces ferroptosis via ferroportin-mediated iron accumulation in endometriosis. Hum Reprod. 2021;36(4):951–964. doi: 10.1093/humrep/deaa363.
- Alvarado-Díaz CP, Núñez MT, Devoto L, et al. Iron overload-modulated nuclear factor kappa-B activation in human endometrial stromal cells as a mechanism postulated in endometriosis pathogenesis. Fertil Steril. 2015;103(2):439–447. doi: 10.1016/j.fertnstert.2014.10.046.
- Pirdel L, Pirdel M. Role of iron overload-induced macrophage apoptosis in the pathogenesis of peritoneal endometriosis. Reproduction. 2014;147(6):R199–207. doi: 10.1530/REP-13-0552.
- Siracusa R, D’Amico R, Cordaro M, et al. The methyl ester of 2-Cyano-3,12-Dioxooleana-1,9-Dien-28-Oic acid reduces endometrial lesions development by modulating the NFkB and Nrf2 pathways. Int J Mol Sci. 2021;22(8):3991. doi: 10.3390/ijms22083991.
- Jin X, Cui L, Zhao W, et al. Decidualization-derived cAMP regulates phenotypic and functional conversion of decidual NK cells from CD56(dim)CD16(-) NK cells. Cell Mol Immunol. 2021;18(6):1596–1598. doi: 10.1038/s41423-021-00675-y.
- Lin YK, Li YY, Li Y, et al. SCM-198 prevents endometriosis by reversing low autophagy of endometrial stromal cell via balancing ERα and PR signals. Front Endocrinol (Lausanne). 2022;13:858176. doi: 10.3389/fendo.2022.858176.
- Pei T, Huang X, Long Y, et al. Increased expression of Yap is associated with decreased cell autophagy in the eutopic endometrial stromal cells of endometriosis. Mol Cell Endocrinol. 2019;491:110432. doi: 10.1016/j.mce.2019.04.012.
- Liang Z, Wu Q, Wang H, et al. Silencing of lncRNA MALAT1 facilitates erastin-induced ferroptosis in endometriosis through miR-145-5p/MUC1 signaling. Cell Death Discov. 2022;8(1):190. doi: 10.1038/s41420-022-00975-w.
- Mikuš M, Goldštajn M, Brlečić I, et al. CTLA4-Linked autoimmunity in the pathogenesis of endometriosis and related infertility: a systematic review. Int J Mol Sci. 2022;23(18):10902. doi: 10.3390/ijms231810902.
- Bilyk O, Coatham M, Jewer M, et al. Epithelial-to-mesenchymal transition in the female reproductive tract: from normal functioning to disease pathology. Front Oncol. 2017;7:145. doi: 10.3389/fonc.2017.00145.
- Duan Y, He Q, Yue K, et al. Hypoxia induced bcl-2/Twist1 complex promotes tumor cell invasion in oral squamous cell carcinoma. Oncotarget. 2017;8(5):7729–7739. doi: 10.18632/oncotarget.13890.
- Liu H, Du Y, Zhang Z, et al. Autophagy contributes to hypoxia-induced epithelial to mesenchymal transition of endometrial epithelial cells in endometriosis. Biol Reprod. 2018;99(5):968–981. doi: 10.1093/biolre/ioy128.
- Choi J, Jo M, Lee E, et al. Differential induction of autophagy by mTOR is associated with abnormal apoptosis in ovarian endometriotic cysts. Mol Hum Reprod. 2014;20(4):309–317. doi: 10.1093/molehr/gat091.
- Ng SW, Norwitz SG, Taylor HS, et al. Endometriosis: the role of iron overload and ferroptosis. Reprod Sci. 2020;27(7):1383–1390. doi: 10.1007/s43032-020-00164-z.
- Ni Z, Li Y, Song D, et al. Iron-overloaded follicular fluid increases the risk of endometriosis-related infertility by triggering granulosa cell ferroptosis and oocyte dysmaturity. Cell Death Dis. 2022;13(7):579. doi: 10.1038/s41419-022-05037-8.
- Yadav AK, Yadav PK, Chaudhary GR, et al. Autophagy in hypoxic ovary. Cell Mol Life Sci. 2019;76(17):3311–3322. doi: 10.1007/s00018-019-03122-4.
- Li S, Zhou Y, Huang Q, et al. Iron overload in endometriosis peritoneal fluid induces early embryo ferroptosis mediated by HMOX1. Cell Death Discov. 2021;7(1):355. doi: 10.1038/s41420-021-00751-2.