Abstract
Context
Substantial evidence suggests that ovarian oxidative stress can result in severe ovarian dysfunction.
Objective
The purpose of this article is to investigate the potential of SIRT6 in alleviating premature ovarian failure (POF) by inhibiting oxidative stress.
Methods
To mimic POF, mice were administered daily subcutaneous injections of d-galactose. The levels of E2, FSH, LH, AMH, and progesterone in serum were measured, along with changes in follicles and SIRT6 levels. Mice were treated with the SIRT6 agonist MDL-800, SIRT6 levels, follicles, and aforementioned hormones were reassessed. The effects of MDL-800 on oxidative stress and apoptosis were subsequently identified. Primary granulosa cells were isolated from mice, and the effects of H2O2 and MDL-800 on cell viability, oxidative stress, SIRT6 level, and apoptosis were evaluated. In addition, the regulation of SIRT6 on H3K9AC/p66SHC was verified by examining changes in protein levels, promoter activity, and the reversal effects of p66SHC overexpression.
Results
MDL-800 mitigated hormone fluctuations, reduced follicle depletion in ovarian tissue, and attenuated oxidative stress and apoptosis in mice. In vitro experiments demonstrated that MDL-800 enhanced the resilience of primary granulosa cells against H2O2, as evidenced by increased cell viability and reduced oxidative stress and apoptosis. Furthermore, SIRT6 was found to decrease H3K9AC and p66SHC levels, as well as attenuate p66SHC promoter activity. The protective effects of MDL-800 on cells were reversed upon p66SHC overexpression.
Conclusion
In summary, this study highlights that activation of SIRT6 can alleviate POF and reduce oxidative stress by degrading H3K9AC and suppressing p66Shc levels in granulosa cells.
Introduction
Premature ovarian failure (POF) is the most common condition influencing female fertility, with an increasing incidence rate among women of reproductive age [Citation1]. It is characterized by a decline in follicles and loss of ovarian function [Citation2, Citation3]. Substantial evidence suggests that oxidative injury to the ovaries can result in severe dysfunction, accompanied by a significant increase in reactive oxygen species (ROS) [Citation4]. ROS-mediated oxidative stress in the ovaries affects follicle development, ovum maturation, ovulation, as well as the quality of oocytes and the potential for embryo development. This not only diminishes the natural pregnancy rate [Citation5–7] but also negatively impacts oocyte fertilization, blastocyst formation, and pregnancy rates in assisted reproduction [Citation8, Citation9], all of which have significant clinical implications. Therefore, addressing the limited fertility in women with POF has become a challenging issue in clinical reproductive medicine, necessitating in-depth research on its pathological etiology and assisted pregnancy strategies [Citation10]. Importantly, the accumulation of ROS leads to increased synthesis of toxic metabolites, primarily inducing apoptosis in ovarian granulosa cells [Citation11, Citation12]. This highlights the critical importance of effectively controlling oxidative stress and apoptosis in ovarian granulosa cells for restoring ovarian function.
SIRT6 belongs to the Sirtuin family of NAD+-dependent enzymes and functions as a chromatin regulatory protein. Members of the Sirtuin family have diverse roles in aging, metabolism, and illness [Citation13, Citation14]. SIRT6, for example, protects smooth muscle cells from aging and reduces atherosclerosis-related damage [Citation15]. In a d-galactose (D-gal)-induced aging mouse model, supplementation with nicotinamide mononucleotide, an NAD + precursor, increased the expression of SIRT6 in intestinal epithelial cells and alleviated intestinal functional decline [Citation16]. The Chinese patent medicine BaZiBuShen formula has been shown to elevate SIRT6 levels and improve sperm quality and testicular shape in aged rats [Citation17]. Additionally, SIRT6 expression is reduced in POF tissues [Citation18], indicating its potential involvement in POF pathology. Hence, the purpose of this article is to investigate whether SIRT6 can mitigate POF by inhibiting oxidative stress.
Given that SIRT6 also functions as a histone deacetylase, targeting lysines K9, K56, and K18 on histone H3 (H3K9AC, H3K56AC, and H3K18AC) [Citation19, Citation20], this study was conducted from this perspective. After confirming the impact of SIRT6 on POF symptoms in mice, the putative regulatory pathways of SIRT6 were investigated. This study identified the regulatory axis of SIRT6 in POF, particularly in granulosa cells, laying the foundation for future research.
Methods and materials
Mouse grouping and modeling
A total of 32 C57BL/6 mice (female, 7-8 weeks old, Aniphe BioLab) were acclimatized for 1 week. Food and water were provided ad libitum throughout the study. The estrous cycle was confirmed by vaginal exfoliated cell smear method. They were randomly divided into four groups of 8 mice each. Mice in the d-gal group received daily subcutaneous injections of d-gal (200 mg/kg/day). The success of modeling was judged by the occurrence of estrous cycle disorder or prolongation of estrous interval. Mice in the control group received an equal volume of saline for 42 days [Citation21]. The MDL-800 group received additional intraperitoneal injection of SIRT6 agonist MDL-800 solution (5% DMSO, 30% PEG-400, and 65% PBS; 10 mg/kg), and the Veh group received the solution without MDL-800 [Citation22]. The vaginal exfoliated cells of the mice were collected after dosing on day 42, smeared, and the estrous cycle was examined under the microscope. Then all mice were euthanized by carbon dioxide, blood was collected by cardiac puncture along with the collection of ovaries.
Granulosa cell isolation and culture
The ovaries were placed in pre-cooled PBS, excess tissue was removed and then placed in pre-cooled DMEM-F12 medium. The follicles were punctured with a 1 ml syringe needle to release ovarian granulosa cells into the DMEM-F12 medium. The granulosa cells were transferred into T25 culture flasks and cultured in an incubator at 37 °C with 5% CO2. When the cell confluence reached 90%, the cells were collected after trypsinization and plated into a 6-well plate at a density of 106 cells/well.
Granulosa cell transfection and treatment
For subsequent mechanistic studies, granulosa cells underwent transfection to overexpress SIRT6 or p66SHC. Plasmids (Genepharma, Shanghai) were mixed with Lipofectamine 2000 (Invitrogen) and added to granulosa cells, and cells were harvested 24 h later. In specific groups, granulosa cells were also treated with MDL-800 (0, 2.5, 5, or 10 μM) for 30 min or stimulated with H2O2 (0, 1.5, 2, or 2.5 μM) for 2 h.
ELISA
Serum biochemical parameters were measured with commercial ELISA kits, including estradiol (E2), follicle-stimulating hormone (FSH), luteinizing hormone (LH), anti-Müllerian hormone (AMH), and progesterone. The values were calculated according to the absorbance obtained from the microplate reader and the equation provided in the kit instruction manual.
H&E staining
Ovarian tissues were fixed overnight in 4% paraformaldehyde, dehydrated with gradient alcohol, embedded in paraffin, and cut into 4 μm thick sections. Deparaffinized tissue sections were stained with hematoxylin solution for 5 min and then stained with eosin for 2 min at room temperature. Sections were dehydrated with gradient alcohol and became transparent using xylene. Specimens were observed under a microscope. Eight slices were randomly selected, and the number of follicles in the slices was manually counted.
RT-qPCR
Ovarian tissues and granulosa cells were lysed with TRIzol® Reagent (Invitrogen), followed by the addition of chloroform, and the lysate was centrifuged at 12,000 × g for 5 min. The RNA in the aqueous phase was precipitated using isopropanol and reverse transcription of RNA to cDNA was conducted using a cDNA Synthesis Kit (EnzyArtisan, Shanghai, China). According to the SYBR Green RT-qPCR kit (Yeasen, Shanghai) instructions, the qPCR reaction system was established and the reaction ran on a 7500 Real-time system. Relative mRNA levels were measured using the ΔΔCt method after normalization to actin.
Western blotting
Ovarian tissues and granulosa cells were lysed in RIPA buffer, lysates were then separated on SDS-polyacrylamide gels for electrophoresis. The separated proteins were transferred to PVDF membranes, followed by the blocking with 5% skim milk. The membranes were incubated with primary antibodies overnight at 4 °C and an HRP-conjugated goat anti-rabbit IgG secondary antibody for 1 h at room temperature. Blots were visualized using an ECL chemiluminescence reagent (Hanbio Biotechnology) and semi-quantified using ImageJ software.
ROS
Fresh ovarian tissue was prepared into a single-cell suspension by enzymatic digestion and transferred to a 96-well plate. Diluted DCFH-DA (Elabscience, Wuhan) was added to the wells and incubated in the dark for 30 min. For granulosa cell samples, after the cells had been treated with MDL-800 or H2O2, diluted DCFH-DA was added. Afterward, cells were washed twice with PBS. The results were photographed under a fluorescence microscope.
MDA and SOD determination
Ovarian tissue and granulosa cells were homogenized or lysed with the lysate and then centrifuged at 10,000g for 10 min to obtain the supernatant. The protein concentration was determined with a BCA protein concentration assay kit. The resulting supernatant was used to measure SOD and MDA levels using commercial kits (Beyotime, Shanghai). The values were calculated according to the absorbance obtained from the microplate reader.
TUNEL
Deparaffinized ovarian tissue sections were incubated with proteinase K for 15 min in a humidified chamber, and endogenous peroxidase was eliminated by 3% hydrogen peroxide. The sections were incubated with TdT labeling buffer (Elabscience) for 1 h at 37 °C and then counterstained with DAPI. TUNEL-positive cells were observed at five random fields of view under a fluorescence microscope.
Immunohistochemistry
Paraffin-embedded ovarian sections were deparaffinized and microwaved in 10 mM sodium citrate, pH 6.0, for antigen retrieval. 3% Hydrogen peroxide was used to eliminate endogenous peroxidase. Sections were incubated in 5% goat serum for 30 min, the primary antibody against cleaved caspase 3 at 4 °C overnight, and biotinylated secondary antibody. Specimens were counterstained with hematoxylin and positive immunostaining was examined under a microscope.
CCK8
Granulosa cells were seeded in 96-well plates and cultured overnight. Following treatment with MDL-800 or H2O2, cells were cultured for 24 h, and then CCK-8 solution was added to each well. After further incubation for 2 h at 37 °C away from light, absorbance was obtained with a microplate reader.
Flow cytometry
Following treatment with MDL-800 or H2O2, granulosa cells were washed twice with PBS, suspended in 1x binding buffer, and incubated with Annexin V-FITC for 10 min in the dark. Thereafter, propidium iodide in 1x binding buffer was added to the cells. Cell apoptosis was then analyzed using flow cytometry (BD Biosciences).
Luciferase reporter assay
Granulosa cells were seeded into cell dishes and cultured overnight. A mixture of pGL3 luciferase reporter plasmids carrying the promoter region of p66Shc and SIRT6 overexpression plasmids were co-transfected into granulosa cells using Lipofectamine 2000. Cells were harvested 24 h after transfection, and luciferase activity was measured by a dual-luciferase reporter assay system (Promega).
Statistical analysis
All data are expressed as means ± SD. In comparisons between two groups, a two-sided, unpaired t-test was used. Comparisons between multiple groups were analyzed with one-way ANOVA followed by Tukey’s test using SPSS software. P <0.05 was considered to be statistically significant.
Results
SIRT6 in POF pathology and hormone
The levels of E2, FSH, LH, AMH, and progesterone in the serum of mice were detected using ELISA. Compared with the control group, the levels of FSH and LH in the D-gal group increased by 2-3fold, and the levels of E2, AMH, and progesterone decreased by >1fold (). Ovarian tissues were subjected to H&E staining, and the follicles in the model group almost disappeared, and the oocytes were significantly reduced (). The results of RT-qPCR and western blotting demonstrated that SIRT6 in the ovarian tissue of the model group decreased significantly (). To investigate the potential role of SIRT6 in POF, mice were administrated the SIRT6 agonist MDL-800. MDL-800 induced an increase in SIRT6 level in ovarian tissue compared with the Veh group (), and H&E staining revealed that MDL-800 significantly alleviated the reduction of follicles and ovum cells (). In addition, the levels of the afore-mentioned hormones also tended to the levels in the control group ().
Figure 1. SIRT6 in POF pathology and hormone (A) The levels of E2, FSH, LH, AMH, and progesterone in the serum of mice were detected using ELISA. (B) The pathological changes of ovarian tissue were evaluated by H&E staining and the number of follicles was counted. (C) RT-qPCR and (D) Western blotting were used to detect the enrichment of SIRT6 in ovarian tissue. (E) Mice were administrated the SIRT6 agonist MDL-800, RT-qPCR and (F) Western blotting was used to detect the enrichment of SIRT6 in ovarian tissue. (G) The effect of MDL-800 on the pathological changes of ovarian tissue was evaluated by H&E staining, and the number of follicles was counted. (H) The effect of MDL-800 on the levels of E2, FSH, LH, AMH, and progesterone in the serum of mice was detected using ELISA. Scale bar = 100 μm. ***P < 0.001.
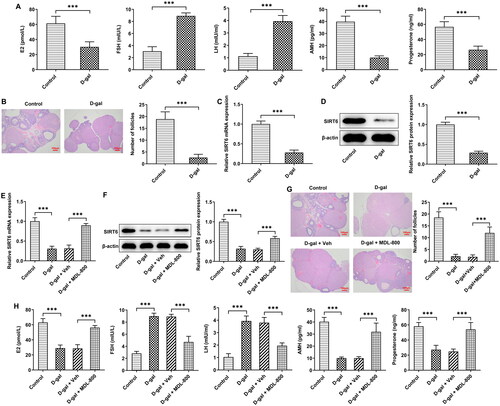
SIRT6 in POF oxidative stress and apoptosis
Oxidative stress in ovarian tissue was assessed by ROS staining () and SOD and MDA levels (). D-gal induced the increase of ROS and MDA and the decrease of SOD in the tissue; MDL-800 weakened the alterations caused by D-gal and relieved the oxidative stress. Apoptosis in ovarian tissue was identified by TUNEL staining () and levels of apoptosis-related proteins (). The number of apoptotic cells in the D-gal group increased significantly, along with decreased Bcl2 and increased Bax and cleaved caspase 3. MDL-800 treatment reduced apoptotic cells and the levels of apoptosis-related proteins were also somewhat restored. Alterations in cleaved caspase 3 upon D-gal and MDL-800 treatment could also be witnessed in immunohistochemistry ().
Figure 2. SIRT6 in POF oxidative stress and apoptosis (A) Oxidative stress in ovarian tissue was assessed by ROS staining and (B) SOD and MDA levels. (C) Apoptosis in ovarian tissue was identified by TUNEL staining. (D) The levels of apoptosis-related proteins in ovarian tissue were evaluated by western blotting. (E) The level of cleaved caspase 3 in ovarian tissue was evaluated by immunohistochemistry. Scale bar = 50 μm. **P < 0.01, ***P < 0.001.
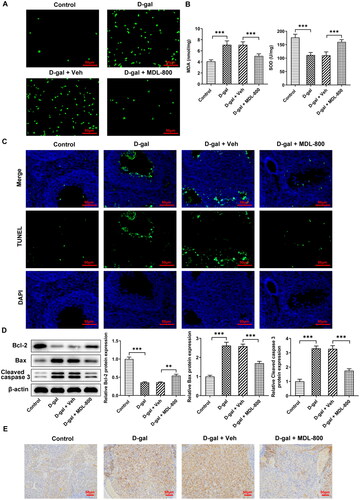
Oxidative stress and apoptosis in granulosa cells
Granulosa cells were stimulated by H2O2 to mimic oxidative stress, and as the concentration increased, cell viability decreased (), whereas ROS levels increased in a concentration-dependent manner (). H2O2 triggered a decrease in SIRT6 levels in cells, also in a concentration-dependent manner (). MDL-800 at a concentration of 0-10 μM had no significant effect on granulosa cell viability (), while MDL-800 pretreatment could reduce the generation of cellular ROS induced by H2O2 (). In addition, MDA and SOD in cells showed a concentration-dependent decrease and increase in response to MDL-800 pretreatment, respectively (). The effect of MDL-800 pretreatment on apoptosis was also evaluated. The results of flow cytometry and western blotting demonstrated that MDL-800 significantly reduced the rate of apoptosis (), accompanied by a gradient increase of Bcl-2 and a gradient decrease of Bax and cleaved caspase 3 ().
Figure 3. Oxidative stress and apoptosis in granulosa cells (A) Granulosa cells were stimulated by H2O2, the viability was assessed using a CCK8 assay. (B) The effect of H2O2 on the levels of ROS was assessed using a DCFH-DA probe. (C) The effect of H2O2 on the levels of SIRT6 was assessed using western blotting. (D) The effect of MDL-800 on viability was assessed using a CCK8 assay. (E) The effect of H2O2 and MDL-800 on the generation of ROS was assessed using a DCFH-DA probe. (F) The effect of H2O2 and MDL-800 on the levels of SOD and MDA was assessed using commercial kits. (G) The effect of H2O2 and MDL-800 on apoptosis was assessed using flow cytometry and (H) western blotting. Scale bar = 50 μm. *P < 0.05, **P < 0.01, ***P < 0.001.
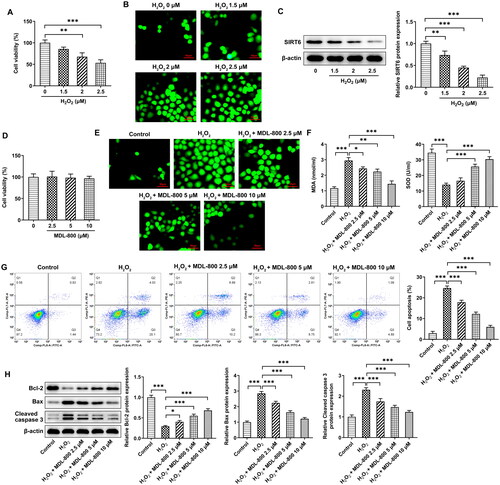
H3K9AC/p66Shc in granulosa cells
To discover the mechanism of SIRT6, the levels of H3K9AC and p66Shc in ovarian tissues were determined using western blotting (). They both increased significantly in the model group, and MDL-800 reduced the alterations in their levels. Based on granulosa cells, RT-qPCR () and western blot () results indicated that p66Shc mRNA and protein, H3K9AC protein levels increased in H2O2-induced granulosa cells, and MDL-800 pretreatment could also reduce p66Shc mRNA and protein, H3K9AC protein levels in vitro. In order to study the probable regulation of the p66Shc promoter region, following the transfection efficiency of SIRT6 overexpression was verified (), it was co-transfected with pGL3. The results of the luciferase assay indicated that SIRT6 overexpression reduced the activity of the p66Shc promoter region, which was the same trend as that after H2O2 treatment (). When granulosa cells overexpressed p66SHC (), the inhibition of ROS () and MDA generation and the promotion of SOD in cells () by MDL-800 were broken. Apoptosis was also induced upon p66SHC overexpression (), as shown by decreased Bcl2 and increased Bax and cleaved caspase 3 ().
Figure 4. H3K9AC/p66Shc in granulosa cells (A) The levels of H3K9AC and p66Shc in ovarian tissues were determined using western blotting. (B) p66Shc mRNA levels in granulosa cells were determined using RT-qPCR. (C) H3K9AC and p66Shc protein levels in granulosa cells were determined using western blotting. (D) The transfection efficiency of SIRT6 overexpression was verified using RT-qPCR and (E) Western blotting. (F) Luciferase assay was used to evaluate the activity of the p66Shc promoter region. (G) The transfection efficiency of p66Shc overexpression was verified using RT-qPCR and (H) western blotting. (I) The effect of p66Shc overexpression on ROS generation was assessed using a DCFH-DA probe. (J) The effect of p66Shc overexpression on MDA and SOD levels was assessed using commercial kits. (K) The effect of p66Shc overexpression on apoptosis was assessed using flow cytometry and (L) western blotting. Scale bar = 50 μm. **P < 0.01, ***P < 0.001.
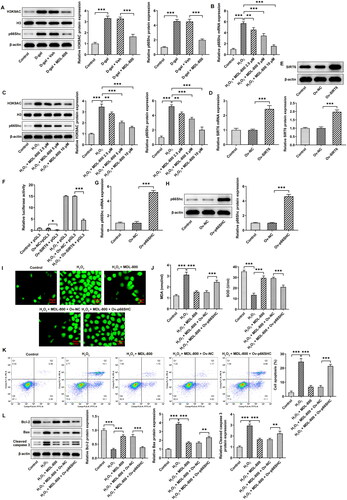
Discussion
Due to the increasing trend of delayed childbirth, ovarian infertility, and subfertility, characterized by preterm ovarian insufficiency and diminished ovarian reserve, have become significant issues in developed countries [Citation23]. The pathogenesis of POF is not entirely understood, but genetic factors and autoimmune disorders are considered major contributors [Citation24, Citation25]. Early identification and intervention are crucial when encountering young women with menstrual irregularities [Citation26], particularly when accompanied by immune system dysfunction. Patients with POF are strongly advised to undergo menopausal hormone or hormone replacement therapy, as it has a positive effect on cardiovascular disease risk factors. Without treatment, these women face higher risk of cardiovascular disease, osteoporosis, dementia, depression, and premature death [Citation27]. While stem cell therapy is an emerging therapeutic approach for POF [Citation28], it is still in its early stages and holds promise as a competitive treatment option.
Female mice possess an estrous cycle similar to humans, albeit shorter, and the accelerated aging observed in the D-gal-induced mouse model of POF [Citation29] closely resembles the human condition. Hence, mouse models are widely utilized to explore the mechanism of ovarian aging. By administering SIRT6 agonists to mice, this study discovered a reduction in ovarian tissue lesions and oxidative stress levels, as well as normalization of related hormone levels in the serum. These findings indicate that activating SIRT6 can slow down the course of POF. While stem cell therapy is currently a major focus of research, studies involving genetic determinants in POF, as evidenced by research on 200,000 European women, demonstrate the importance of intervention in the canonical DNA damage response pathway to improve fertility and extend reproductive lifespan in mice [Citation30]. Thus, developing therapeutic methods with a genetic focus is equally significant.
Among the various forms of cell death induced by oxidative stress in ovarian granulosa cells, apoptosis is considered to be the primary mechanism. Apoptosis is a tightly regulated process that plays a crucial role in ovarian follicle development and atresia [Citation31]. Besides oxidative stress, apoptosis is also controlled by multiple factors, including hormonal signaling and growth factors [Citation32]. Activation of apoptotic pathways in response to oxidative stress leads to the controlled elimination of damaged or non-functional granulosa cells, which is essential for maintaining the overall health and function of ovarian follicles [Citation33, Citation34]. While apoptosis is the predominant form of cell death, other types such as autophagy, necrosis, and ferroptosis can also contribute to the overall response of ovarian granulosa cells to oxidative stress [Citation7, Citation35, Citation36].
Previous studies have demonstrated that SIRT6 degrades the expression of Notch signaling proteins by deacetylating histone H3K9 [Citation37]. Histone H3K9 binds to the promoter of the target gene, stabilizing gene expression. When SIRT6 degrades H3K9, it inhibits the promoter region level of the target gene, thereby inhibiting the expression of mRNA and protein. Under high glucose-induced conditions, the level of H3K9 acetylation (H3K9AC) increases in the promoter region of p66Shc[Citation38], suggesting that H3K9AC is enriched in the p66Shc promoter region and regulates its stable expression. Does such a regulatory axis exist in POF? This study verified that SIRT6 degrades p66SHC through H3K9AC in granulosa cells. p66Shc was originally reported to mediate growth signals in normal and cancer cells and is also considered a pro-apoptotic protein involved in cellular responses to oxidative stress. It has been studied extensively in vascular diseases, where it controls lesions by generating and intensifying pro-apoptotic ROS signals [Citation39, Citation40]. Knockout of p66Shc can reduce the resting and maximum oxidative capacity of cells, promoting a switch from oxidative metabolism to the glycolytic pathway to prolong lifespan [Citation41]. Additionally, it has been found to induce ovarian fibrosis in rats with polycystic ovary syndrome [Citation42], demonstrating its involvement in the progression of various illnesses. Given that p66SHC has been shown to influence B cell autophagy [Citation43], and considering the relationship between POF and immunity, it is a limitation of this study that the role of this regulatory axis in immune regulation was not further investigated.
In summary, this study highlights that activating SIRT6 can alleviate POF and reduce oxidative stress through the degradation of p66Shc via H3K9AC in granulosa cells. In the future, our focus will continue to be on the potential of targeting SIRT6 in the treatment of POF, and we strive to promote the development of additional therapies for this condition.
Author contributions
CS and YJ contributed to the concept, experiments, and draft. JL, YH, YL, and DF contributed to the experiments and data analysis. All authors approved the final version.
Ethics approval
The animal experiment was approved by West China Second University Hospital, Sichuan University, number 2022-040. Operations were strictly performed according to the National Institute of Health Guide for the Care and Use of Laboratory Animals.
Disclosure statement
No potential conflict of interest was reported by the authors.
Data availability statement
All data generated or analyzed during this study are included in this article. Further inquiries can be directed to the corresponding author.
Additional information
Funding
References
- Wang J, Liu W, Yu D, et al. Research progress on the treatment of premature ovarian failure using mesenchymal stem cells: a literature review. Front Cell Dev Biol. 2021;9:1. doi: 10.3389/fcell.2021.749822.
- Liu L, Wang H, Xu GL, et al. Tet1 deficiency leads to premature ovarian failure. Front Cell Dev Biol. 2021;9:644135. doi: 10.3389/fcell.2021.644135.
- Ghahremani-Nasab M, Ghanbari E, Jahanbani Y, et al. Premature ovarian failure and tissue engineering. J Cell Physiol. 2020;235(5):4217–9. doi: 10.1002/jcp.29376.
- Pandey AK, Gupta A, Tiwari M, et al. Impact of stress on female reproductive health disorders: Possible beneficial effects of shatavari (Asparagus racemosus). Biomed Pharmacother. 2018;103:46–49. doi: 10.1016/j.biopha.2018.04.003.
- Lin L, Gao W, Chen Y, et al. Reactive oxygen species-induced SIAH1 promotes granulosa cells’ senescence in premature ovarian failure. J Cell Mol Med. 2022;26(8):2417–2427. doi: 10.1111/jcmm.17264.
- Prasad S, Tiwari M, Pandey AN, et al. Impact of stress on oocyte quality and reproductive outcome. J Biomed Sci. 2016;23:36. doi: 10.1186/s12929-016-0253-4.
- Yadav AK, Yadav PK, Chaudhary GR, et al. Autophagy in hypoxic ovary. Cell Mol Life Sci CMLS. 2019;76(17):3311–3322. doi: 10.1007/s00018-019-03122-4.
- Tamura H, Jozaki M, Tanabe M, et al. Importance of melatonin in assisted reproductive technology and ovarian aging. Int J Mol Sci. 2020;21(3). doi: 10.3390/ijms21031135.
- Yong W, Ma H, Na M, et al. Roles of melatonin in the field of reproductive medicine. Biomed Pharmacother. 2021;144:112001. doi: 10.1016/j.biopha.2021.112001.
- Tsiligiannis S, Panay N, Stevenson JC. Premature ovarian insufficiency and long-term health consequences. Curr Vasc Pharmacol. 2019;17(6):604–609. doi: 10.2174/1570161117666190122101611.
- An R, Wang X, Yang L, et al. Polystyrene microplastics cause granulosa cells apoptosis and fibrosis in ovary through oxidative stress in rats. Toxicology. 2021;449:152665. doi: 10.1016/j.tox.2020.152665.
- Yang H, Xie Y, Yang D, et al. Oxidative stress-induced apoptosis in granulosa cells involves JNK, p53 and Puma. Oncotarget. 2017;8(15):25310–25322. doi: 10.18632/oncotarget.15813.
- Kuang J, Chen L, Tang Q, et al. The role of Sirt6 in obesity and diabetes. Front Physiol. 2018;9:135. doi: 10.3389/fphys.2018.00135.
- Liu G, Chen H, Liu H, et al. Emerging roles of SIRT6 in human diseases and its modulators. Med Res Rev. 2021;41(2):1089–1137. doi: 10.1002/med.21753.
- Grootaert MOJ, Finigan A, Figg NL, et al. SIRT6 protects smooth muscle cells from senescence and reduces atherosclerosis. Circ Res. 2021;128(4):474–491. doi: 10.1161/CIRCRESAHA.120.318353.
- Ru M, Wang W, Zhai Z, et al. Nicotinamide mononucleotide supplementation protects the intestinal function in aging mice and D-galactose induced senescent cells. Food Funct. 2022;13(14):7507–7519. doi: 10.1039/d2fo00525e.
- Li L, Chen B, An T, et al. BaZiBuShen alleviates altered testicular morphology and spermatogenesis and modulates Sirt6/P53 and Sirt6/NF-κB pathways in aging mice induced by D-galactose and NaNO(2). J Ethnopharmacol. 2021;271:113810. doi: 10.1016/j.jep.2021.113810.
- Zhang J, Fang L, Lu Z, et al. Are sirtuins markers of ovarian aging? Gene. 2016;575(2 Pt 3):680–686. doi: 10.1016/j.gene.2015.09.043.
- Wei H, Khawar MB, Tang W, et al. Sirt6 is required for spermatogenesis in mice. Aging. 2020;12(17):17099–17113. doi: 10.18632/aging.103641.
- Huang Z, Zhao J, Deng W, et al. Identification of a cellularly active SIRT6 allosteric activator. Nat Chem Biol. 2018;14(12):1118–1126. doi: 10.1038/s41589-018-0150-0.
- Yan Z, Dai Y, Fu H, et al. Curcumin exerts a protective effect against premature ovarian failure in mice. J Mol Endocrinol. 2018;60(3):261–271. doi: 10.1530/JME-17-0214.
- Jin J, Li W, Wang T, et al. Loss of proximal tubular sirtuin 6 aggravates unilateral ureteral obstruction-induced tubulointerstitial inflammation and fibrosis by regulation of β-catenin acetylation. Cells. 2022;11(9). doi: 10.3390/cells11091477.
- Kasapoğlu I, Seli E. Mitochondrial dysfunction and ovarian aging. Endocrinology. 2020;161(2):1–11. doi: 10.1210/endocr/bqaa001.
- Yang Q, Mumusoglu S, Qin Y, et al. A kaleidoscopic view of ovarian genes associated with premature ovarian insufficiency and senescence. FASEB J Off Publ Federation Am Soc Exp Biol. 2021;35(8):e21753. doi: 10.1096/fj.202100756R.
- Szeliga A, Calik-Ksepka A, Maciejewska-Jeske M, et al. Autoimmune diseases in patients with premature ovarian insufficiency-our current state of knowledge. Int J Mol Sci. 2021;22(5):2594. doi: 10.3390/ijms22052594.
- Ding T, Yan W, Zhou T, et al. Endocrine disrupting chemicals impact on ovarian aging: evidence from epidemiological and experimental evidence. Environ Pollut. 2022;305:119269. doi: 10.1016/j.envpol.2022.119269.
- Anagnostis P, Paschou SA, Katsiki N, et al. Menopausal hormone therapy and cardiovascular risk: where are we now? Curr Vasc Pharmacol. 2019;17(6):564–572. doi: 10.2174/1570161116666180709095348.
- Saha S, Roy P, Corbitt C, et al. Application of stem cell therapy for infertility. Cells. 2021;10(7):1613. doi: 10.3390/cells10071613.
- Liang X, Yan Z, Ma W, et al. Peroxiredoxin 4 protects against ovarian ageing by ameliorating D-galactose-induced oxidative damage in mice. Cell Death Dis. 2020;11(12):1053. doi: 10.1038/s41419-020-03253-8.
- Ruth KS, Day FR, Hussain J, et al. Genetic insights into biological mechanisms governing human ovarian ageing. Nature. 2021;596(7872):393–397. doi: 10.1038/s41586-021-03779-7.
- Jiang JY, Cheung CK, Wang Y, et al. Regulation of cell death and cell survival gene expression during ovarian follicular development and atresia. Front Biosci J Virtual Library. 2003;8:d222–d237.
- Matsuda F, Inoue N, Manabe N, et al. Follicular growth and atresia in mammalian ovaries: regulation by survival and death of granulosa cells. J Reprod Dev. 2012;58(1):44–50. doi: 10.1262/jrd.2011-012.
- Guan Y, Zhang W, Wang X, et al. Cell-free DNA induced apoptosis of granulosa cells by oxidative stress. Clin Chim Acta Int J Clin Chem. 2017;473:213–217. doi: 10.1016/j.cca.2016.11.023.
- Hoque SAM, Umehara T, Kawai T, et al. Adverse effect of superoxide-induced mitochondrial damage in granulosa cells on follicular development in mouse ovaries. Free Rad Biol Med. 2021;163:344–355. doi: 10.1016/j.freeradbiomed.2020.12.434.
- Shen M, Jiang Y, Guan Z, et al. Protective mechanism of FSH against oxidative damage in mouse ovarian granulosa cells by repressing autophagy. Autophagy. 2017;13(8):1364–1385. doi: 10.1080/15548627.2017.1327941.
- Zhang S, Liu Q, Chang M, et al. Chemotherapy impairs ovarian function through excessive ROS-induced ferroptosis. Cell Death Dis. 2023;14(5):340. doi: 10.1038/s41419-023-05859-0.
- Liu M, Liang K, Zhen J, et al. Sirt6 deficiency exacerbates podocyte injury and proteinuria through targeting Notch signaling. Nat Commun. 2017;8(1):413. doi: 10.1038/s41467-017-00498-4.
- Mishra M, Duraisamy AJ, Bhattacharjee S, et al. Adaptor protein p66Shc: a link between cytosolic and mitochondrial dysfunction in the development of diabetic retinopathy. Antioxid Redox Signal. 2019;30(13):1621–1634. doi: 10.1089/ars.2018.7542.
- Haslem L, Hays JM, Hays FA. p66Shc in cardiovascular pathology. Cells. 2022;11(11):1855. doi: 10.3390/cells11111855.
- Boengler K, Bornbaum J, Schlüter KD, et al. P66shc and its role in ischemic cardiovascular diseases. Basic Res Cardiol. 2019;114(4):29. doi: 10.1007/s00395-019-0738-x.
- Pinton P, Rizzuto R. p66Shc, oxidative stress and aging: importing a lifespan determinant into mitochondria. Cell Cycle (Georgetown, Tex). 2008;7(3):304–308. doi: 10.4161/cc.7.3.5360.
- Wang D, Wang T, Wang R, et al. Suppression of p66Shc prevents hyperandrogenism-induced ovarian oxidative stress and fibrosis. J Transl Med. 2020;18(1):84. doi: 10.1186/s12967-020-02249-4.
- Onnis A, Cassioli C, Finetti F, et al. Regulation of selective B CELL autophagy by the pro-oxidant adaptor p66SHC. Front Cell Dev Biol. 2020;8:193. doi: 10.3389/fcell.2020.00193.