Abstract
Objective
Medroxyprogesterone acetate (MPA) may increase the risk of atherosclerosis during hormone replacement therapy (HRT); therefore, the effect of progestogens other than MPA on atherosclerotic lesions requires evaluation. Adhesion of monocytes to vascular endothelial cells is an important early step in atherosclerosis progression. MCP-1 is a key chemokine that promotes monocyte migration and adhesion to vascular endothelial cells. In this study, we investigated the effects of dienogest (DNG), an alternative progestogen, on monocyte adhesion and cytokine expression in human umbilical vein endothelial cells (HUVECs).
Study Design
HUVECs were treated with DNG, natural progesterone, or MPA, followed by interleukin (IL)-1β stimulation. The mRNA expression of adhesion molecules (E-selectin and ICAM-1) and cytokines (MCP-1 and IL-6) was examined using real-time PCR. A flow chamber system was used to examine the effect of DNG on the adhesion of U937 monocytic cells to monolayer HUVECs.
Results
Unlike MPA, DNG did not alter the mRNA expression of E-selectin, ICAM-1, MCP-1, and IL-6 in HUVECs. Moreover, it did not increase the number of monocytes adhering to HUVECs in the flow chamber system. However, MPA treatment significantly enhanced monocyte adhesion to HUVECs (p < 0.05).
Conclusions
DNG had no effect on the mRNA expression of adhesion molecules and cytokines in HUVECs, as well as the monocyte adhesion to HUVECs, suggesting that DNG can be explored as an alternative to MPA for HRT.
Introduction
Hormone replacement therapy (HRT) is used to alleviate menopausal symptoms caused by estrogen deficiency and reduce the risk of osteoporosis. The incidence of cardiovascular diseases, including atherosclerosis, is higher in postmenopausal women than in men of the same age. The blood estrogen level in postmenopausal women is significantly lower than that in men, suggesting a protective effect of estrogen on atherosclerosis [Citation1–5]. Therefore, HRT in postmenopausal women is believed to reduce the risk of atherosclerosis. However, data from the Women’s Health Initiative (WHI) HRT trials contradicted this hypothesis. These trials demonstrated that HRT with conjugated equine estrogen (CEE) and medroxyprogesterone acetate (MPA) increased the risk of coronary heart disease (CHD), stroke, venous thromboembolism, and breast cancer compared to CEE alone, which led to the termination of these trials [Citation6, Citation7]. Since then, it has become necessary to fundamentally reevaluate conventional notions of HRT regarding its efficacy, indications, and administration routes.
Clinical trials of HRT have used CEE and MPA as substitutes for estrogen and progestogen, respectively; however, our previous in vitro studies suggest that both CEE and MPA may be risk factors for atherosclerosis [Citation8, Citation9]. Subsequent trials after termination of the WHI trials have tested the risk and benefit of estrogen–progestogen therapy with progestogens other than MPA, including norethisterone acetate (NETA), levonorgestrel, dydrogesterone, dienogest (DNG), and natural progesterone (P4) [Citation10, Citation11]. A prospective cohort study of French women showed that estrogen combined with P4 or dydrogesterone was not associated with an increased risk of breast cancer, whereas estrogen alone and estrogen combined with other types of progestogens (e.g. MPA) were associated with a significantly increased risk [Citation11]. Another estrogen–progestogen therapy trial showed that the risk of cardiovascular events was higher with continuous combination therapy than that with cyclic combination therapy, with NETA having the highest risk [Citation10]. However, limited studies have evaluated the effects of individual progestogens on atherosclerotic lesions [Citation12–14]. We had previously established experimental protocols to evaluate the effect of steroid hormones on atherosclerotic lesions and found that MPA and CEE may alter the expression of adhesion molecules in human umbilical vein endothelial cells (HUVECs) and increase the risk of CHD [Citation8, Citation9].
Although many progestogens have been studied, the progestogen that shows optimal effects in HRT remains unknown. The optimal effects include the suppression of uterine cancer risk and the prevention of lifestyle diseases such as CHD. DNG, a progestogen with strong progestational activity and without androgenic, glucocorticoid, or mineralocorticoid activity, inhibits endometrial proliferation and exerts antitumor effects in uterine cancer cell lines [Citation15]. It also has an inhibitory effect on ovarian function and the atrophy of endometriosis lesions. More importantly, the efficacy and safety of DNG have been extensively evaluated including its long-term use. Therefore, it is used in treating dysmenorrhea and endometriosis worldwide. Endometriosis is an estrogen-dependent disease; however, the usual dosage of DNG (2 mg/day) suppresses estrogen secretion only in the early follicular phase, and its suppressive effect is less than that of GnRH agonists. Nevertheless, the non-inferiority of DNG over GnRH agonists in improving dysmenorrhea has been demonstrated. This suggests that DNG may exert other effects that contribute to its therapeutic efficacy, which may include an anti-inflammatory effect [Citation16]. For example, DNG suppresses proinflammatory cytokines, such as IL-6 and MCP-1, in endometrial stromal cells in vitro [Citation17–19]. However, whether DNG has an anti-inflammatory effect on pathologies other than endometriosis is unclear.
Limited studies have examined the effects of DNG on the risk of atherosclerosis in vascular endothelial cells at the cellular and molecular levels. In this study, we aimed to examine the effect of DNG in HUVECs on the mRNA expression of cell adhesion molecules, MCP-1, and IL-6, which are common markers increased in atherosclerotic lesions.
Materials and methods
Steroids
P4, MPA, dihydrotestosterone, mifepristone (RU486), and hydroxyflutamide were purchased from Sigma-Aldrich (St. Louis, MO, USA) and DNG and dexamethasone (DEX) from Nacalai Tesque, Inc. (Kyoto, Japan).
Cell cultures and treatments
Umbilical cord samples from female infants were obtained from patients who underwent cesarean section without complications. This protocol has been approved by the Kyoto Prefectural University of Medicine Institutional Review Board (ERB-C-1519). The samples were stored in DMEM/Ham’s F-12 medium after collection and transported to the laboratory. HUVECs were separated as previously described and cultured at 37 °C under 5% CO2 in 75 cm2 tissue culture flasks with HuMedia containing 10% charcoal-treated FBS, hEGF (10 µg/mL), hydrocortisone (1.34 mg/mL), hFGF-B (5 µg/mL), heparin (10 mg/mL), gentamicin (50 mg/mL), and amphotericin B (50 µg/mL), until cells reached preconfluence. Cells grown to preconfluence between passages 1 and 3 were harvested by trypsinization and seeded in six-well plates (2 × 105 cells/well). When cells reached preconfluence in the wells, P4, MPA, and DNG were added to each well and incubated for 48 h. In some experiments, DEX (GR agonist), dihydrotestosterone (AR agonist), RU486 (GR antagonist), and hydroxyflutamide (AR antagonist) were added simultaneously. Recombinant human IL-1β (40 U/mL; Genzyme, Cambridge, MA, USA) was added 4 h before RNA extraction.
Real-time PCR
Total RNA was extracted from HUVECs using the RNeasy Mini Kit (Qiagen, Venlo, Netherlands) according to the manufacturer’s instructions. RNA concentrations were quantified by ultraviolet absorption (optical density: 260 nm/280 nm) using a NanoDrop instrument (Thermo Fisher Scientific). RNA (1 µg) from each sample was reverse-transcribed to cDNA and amplified using ReverTra Ace® qPCR RT Master Mix (Toyobo, Osaka, Japan) and GeneAmp PCR9700 (Applied Biosystems). Gene expression was analyzed using real-time PCR, which was performed using Thunderbird Master Mix (Toyobo) and CFX Connect Real-Time PCR System (Bio-Rad, Hercules, CA, USA). Cycle time (Ct) values were used to quantify relative gene expression. The cycle time (Ct) values obtained were used to quantify the relative expression of the genes of interest. The Ct values were first normalized to the internal control gene (GAPDH), and then the fold changes of target genes (ΔΔCt) in all the groups were calculated and represented as relative expression values.
Flow chamber system
We assessed the IL-1β-induced adhesion of U937 monocytoid cells to HUVEC monolayers under shear stress using the flow chamber system described by Gerszten et al. [Citation20], with modifications as previously described [Citation21, Citation22]. The chamber comprised a glass slide with a confluent HUVEC monolayer attached to the polycarbonate base. Two flat surfaces were held approximately 270 mm apart using a silicone rubber gasket (Dow Corning, Midland, MI, USA). The flow across the monolayer was controlled using a syringe pump (Harvard Apparatus; South Natick, MA, USA). A U937 monocytoid cell suspension (10,000 cells/mL) in HBSS was perfused into the flow chamber. The experiments were videotaped using a color camera mounted on an inverted microscope. Adherent cells were defined as those that remained stationary for ≥ 30 s. Adherent U937 monocytoid cell number was counted 5 min after cell perfusion. A representative video of the flow chamber system is available in the supplementary file.
Western blot analysis
Cultured cellular protein was extracted using radioimmunoprecipitation buffer (Nacalai Tesque) and then mixed with sodium dodecyl sulfate (SDS) sample buffer [62.5 mM/L Tris-HCl (pH 6.8), 10% glycerol, 1% SDS, 0.1% 2-mercaptoethanol, and 1 mM phenylmethylsulfonyl fluoride] and heated for 5 min at 100 °C. The lysates were loaded onto polyacrylamide gels, subjected to electrophoresis, and transferred to a polyvinylidene difluoride membrane. The blot was incubated with blocking buffer (5% skimmed milk, Tris-buffered saline containing 0.1% Tween-20) for 1 h at room temperature, then incubated overnight at 4 °C with the appropriate primary antibodies to ICAM-1 (1:200), E-selectin (1:200). The blots were incubated with the appropriate secondary antibodies for 1 h at room temperature. Immune complexes were visualized using the Chemi-Lumi One Super (Nacalai Tesque) abd ChemiDoc XRSþ systems (Bio-Rad). Densitometric analysis of blots was performed.
Enzyme-linked immunosorbent assay
The concentrations of IL-6 and MCP-1 in the culture supernatant were measured using commercially available ELISA Kits (Quantikine ELISA Kit R&D Systems, Minneapolis, MN, USA) according to the manufacturer’s instructions.
Statistical analysis
Data are expressed as the mean ± standard error of mean (SEM) of three separate experiments. Differences in progestogen stimulation were analyzed using one-way analysis of variance followed by the Bonferroni–Dunn test for multiple comparisons. p < 0.05 was considered statistically significant.
Results
DNG did not increase the mRNA and protein expression of adhesion molecules in HUVECs
First, the effect of MPA and DNG on the mRNA levels of cell adhesion molecules in HUVECs was investigated using quantitative real-time PCR. Compared with the control cells, MPA-treated cells had a 1.96- and 1.65-fold increase in ICAM-1 and E-selectin mRNA expression, respectively (p < 0.05, ). In contrast, compared to no treatment, DNG treatment did not change ICAM-1 and E-selection mRNA expression (). On protein expression of ICAM-1 and E-selectin, we observed no significant change in DNG-treated cells as compared with the control (Supplementary Figure A and B).
Figure 1. mRNA expression of adhesion molecules, cytokines, and chemokines in human umbilical vein endothelial cells (HUVECs) treated with progestogens. Steroid-deprived and serum-starved HUVECs were treated for 48 h in the presence of various progestogens, namely 100 nM natural progesterone (P4), 100 nM dienogest (DNG), and 100 nM medroxyprogesterone acetate (MPA). The cells were stimulated with interleukin (IL)-1β (40 U/mL) for 4 h. Relative mRNA expression of (A) ICAM-1, (B) E-selectin, (C) IL-6, and (D) MCP-1 was measured by real-time PCR and compared with vehicle-only treatment. Data are presented as the mean ± SEM of three biological replicates. *P < 0.05 vs. vehicle only.
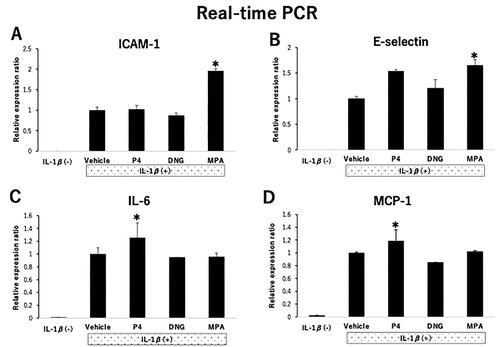
DNG did not change the mRNA and protein expression of cytokines and chemokines in HUVECs
Next, we examined how DNG affected IL-6 and MCP-1 mRNA expression in HUVECs using quantitative real-time PCR. Compared with the control cells, P4-treated cells had a 1.25- and 1.19-fold increase in IL-6 and MCP-1 mRNA expression, respectively (p < 0.05, ). In contrast, compared with the control cells, DNG-treated cells had no changes in IL-6 and MCP-1 mRNA expression (). On protein expression of MCP-1, we observed no significant change in DNG-treated cells as compared with the control (Supplementary Figure D). IL-6 protein expression levels did not differ between P4, DNG, and MPA treated-cells (Supplementary Figure C).
DNG did not change the number of monocytoid cells adhered to HUVECs
To assess the effects of DNG and MPA on cell adherence under flow conditions, we used a flow chamber system to mimic the physiological adherence of monocytes to endothelial cells. MPA significantly increased the adherence of U937 monocytoid cells compared with that of control cells (56.0 ± 1.5 vs. 46.5 ± 3.5 adherent cells/10 fields, p < 0.05, ). Contrarily, DNG did not change the number of adherent monocytoid cells compared with the control cells (44.1 ± 2.0 vs. 46.5 ± 3.5 adherent cells/10 fields, ).
Figure 2. Effects of various progestogens on the adhesion of U937 monocytoid cells to human umbilical vein endothelial cells (HUVECs) under flow conditions.HUVECs were treated with various progestogens as described in . (A) Representative micrographs of HUVECs in a flow chamber system. The indicated small round cells were adherent to the U937 monocytoid cells. Sample fields are at 40× magnification (left), with details of the cells (right) shown at 100× magnification. (B) U937 monocytoid cells at 10,000/mL were perfused over HUVEC monolayers, and adherent cells were counted 5 min after perfusion. The total number of adherent cells in 10 randomly selected microscopic fields of each sample is shown. Data are presented as the mean ± SEM of three biological replicates. *P < 0.05 vs. vehicle only.
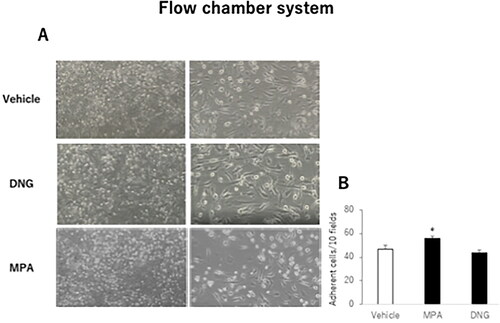
Addition of a GR agonist to DNG increases the mRNA expression of adhesion molecules in HUVECs
We previously found that the expression of adhesion molecules was enhanced by simultaneous addition of DEX, a GR agonist, to MPA. As DNG only acts on PR and has no effects on GR or AR, we expected the presence or absence of GR action to explain the difference in the expression of adhesion molecules between DNG and MPA. Simultaneous addition of DEX to DNG treatment increased E-selectin and ICAM-1 mRNA expression in HUVECs compared to that in DNG treatment alone ().
Figure 3. Effects of the GR agonist on the expression of endothelial adhesion molecules in dienogest (DNG)-treated human umbilical vein endothelial cells (HUVECs).HUVECs were treated with 100 nM DNG in the presence or absence of 100 nM dexamethasone (DEX), as described in . Relative mRNA expression of (A) E-selectin and (B) ICAM-1 in HUVECs. Data are presented as the mean ± SEM of three biological replicates.
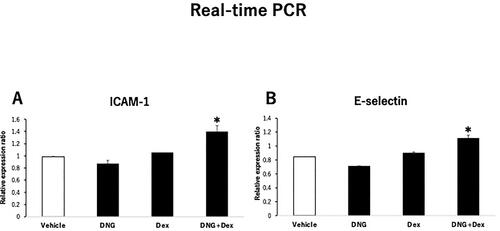
Discussion
As increased risk of CHD and breast cancer was observed in the WHI HRT trials, clinical and mechanistic studies are required to clarify the diverse effects of different progestogen types on CHD risk [Citation6, Citation7]. However, evidence showing the effects of progestogens on vascular endothelial cells regarding atherosclerosis risk is extremely limited [Citation12, Citation13]. This study demonstrates that DNG, a synthetic progestogen, had no effect on the mRNA expression of adhesion molecules and cytokines in HUVECs. Moreover, monocyte adhesion to HUVECs was not affected by DNG. These results suggest that DNG does not increase atherosclerosis risk in vascular endothelial cells.
Atherosclerosis results from an inflammatory response triggered by vascular endothelial cell damage. Endothelial cell damage is stimulated by activated platelets, and the expression of cell adhesion molecules, such as ICAM-1 and E-selectin, increases on the cell surface through the activated NF-κB pathway. Adhesion of blood-migrating monocytes to endothelial cells via specific receptor-adhesion molecule interactions is an important early event in the atherosclerotic lesion formation [Citation23–26]. Here, we examined monocyte adhesion to HUVECs using a flow chamber system. The parallel-plate flow chamber system was initially designed to investigate the interactions between monocytes and the endothelium under physiological conditions. Laminar flow conditions more closely mimic physiological conditions than rotated or static models because the flow imposes shear stress that leads to monocyte rolling on endothelial cells [Citation20, Citation21]. Therefore, the flow assay may be more sensitive than conventional cell adhesion assays under static or agitated conditions. We previously used this flow chamber system to confirm that elevated adhesion molecule expression in vascular endothelium increased adherent monocyte expression [Citation12]. Here, DNG did not change ICAM-1 and E-selectin mRNA levels in HUVECs. Furthermore, HUVEC pretreatment with MPA promoted the U937 monocytic cell adhesion to HUVECs, whereas DNG pretreatment had no effect, suggesting that DNG treatment is not associated with early events of atherosclerotic lesion formation in vascular endothelial cells.
Inflammatory cytokines are known to affect the cell adhesion in atherosclerotic lesions [Citation27–29]. For example, MCP-1 promotes monocyte migration and adhesion to endothelial cells as well as subendothelial infiltration. Activated monocytes increase the expression of various cytokines such as IL-6. In this series of processes, monocytes and endothelial cells continuously produce MCP-1 [Citation30]. Plasma MCP-1 concentrations increase as part of an inflammatory response, which is accompanied by the formation of atherosclerotic lesions. Additionally, elevated MCP-1 concentrations are associated with increased risk of angina pectoris, myocardial infarction, and heart failure, suggesting that it can be used as a diagnostic marker for acute coronary syndromes [Citation31]. Moreover, MCP-1 expression increases in endothelial cells with atherosclerotic lesions [Citation32]. We found that MPA increased MCP-1 mRNA expression in HUVECs, whereas DNG treatment did not significantly change the expression, suggesting that DNG had little effect on MCP-1 and, consequently, had little effect on monocyte-cell adhesion molecules during atherosclerotic lesion formation.
Progestogens are classified into three major subtypes: 17α-hydroxyprogesterone derivatives (e.g. MPA and DG), 19-nortestosterone derivatives (e.g. norethisterone acetate, levonorgestrel, desogestrel, and DNG), and 17α-spironolactone derivatives (e.g. drospirenone). Individual progestins bind to various steroid receptors (e.g. PR, ER, AR, GR, and MR) with different affinities, thereby exerting unique and complex actions on target tissues. DNG is a fourth-generation progestin with high progestational and anti-androgenic activity. Moreover, DNG is used for the treatment of endometriosis because of its ability to promote differentiation and inhibit proliferation by desmoplasia. MPA is a progestogen characterized by its glucocorticoid activity. Our previous study found that the co-treatment of the GR agonist DEX and the AR agonist dihydrotestosterone on HUVECs significantly increased the expression of adhesion molecules compared to controls, but no significant change was observed in the number of monocyte adhesions. Additionally, the co-treatment of the GR antagonist RU486 and MPA partially suppressed the MPA-induced increase in the expression of adhesion molecules, but no changes were observed when cells were co-treated with the AR antagonist and MPA. It indicates that the action of MPA is partly mediated by GR [Citation8]. Therefore, it is possible that DNG, which has no glucocorticoid activity, does not increase the expression of adhesion molecules. In this study, the co-treatment of DEX and DNG increased the expression of adhesion molecules, suggesting that GR action may still have negative effects on atherosclerosis.
Atherosclerosis is not only caused by vascular endothelial cell dysfunction but is also affected by lipid profile, insulin resistance, and changes in the autonomic nervous system. Therefore, in vitro studies possess limited physiological relevance, and in vivo studies are required to thoroughly evaluate the effects of DNG in humans. The results of human clinical trials indicate that the oral administration of DNG has little effect on lipid metabolism [Citation33]. A major adverse effect of DNG administration is irregular genital bleeding, which may be caused by desmoplasia of the endometrium. Moreover, long-term oral administration of DNG may lead to bone loss. However, DNG maintains estradiol secretion within a range equivalent to the estrogen level required to treat endometriosis, which does not stimulate endometrial proliferation and is less likely to cause bone density loss compared to GnRH agonists [Citation34]. Furthermore, the possibility of bone density loss may be further reduced if DNG is administered in combination with estrogen. DNG is known to be safe, without any other reported side effects; consequently, it can be used for a long time. Therefore, DNG can be used as a progestin for HRT. However, its effects on bone metabolism should be examined first to avoid potential side effects.
Conclusions
In conclusion, our in vitro study provides novel evidence that DNG does not change mRNA expression of adhesion molecules and MCP-1 in HUVECs. It also had no effect on the adherence of U937 monocytic cells to HUVECs. Our findings suggest that DNG does not increase the risk of atherosclerosis and may be a better progestogen used in HRT for postmenopausal women. However, further in vivo studies and clinical trials are required to investigate the efficacy and side effects in humans.
Abbreviations | ||
ANOVA | = | analysis of variance |
CEE | = | conjugated equine estrogen |
CHD | = | coronary heart disease |
DEX | = | dexamethasone |
DNG | = | dienogest |
HRT | = | hormone replacement therapy |
HUVEC | = | human umbilical vein endothelial cell |
IL | = | interleukin |
MPA | = | medroxyprogesterone acetate |
NETA | = | norethisterone acetate |
WHI | = | Women’s Health Initiative |
Supplemental Material
Download MS Word (1.9 MB)Disclosure statement
No potential conflict of interest was reported by the author(s).
Data availability statement
All data generated or analyzed during this study are included in this published article and available from the corresponding author on reasonable request.
Additional information
Funding
References
- Kannel WB, Hjortland MC, McNamara PM, et al. Menopause and risk of cardiovascular disease: the Framingham study. Ann Intern Med. 1976;85(4):1–6. doi: 10.7326/0003-4819-85-4-447.
- Colditz GA, Willett WC, Stampfer MJ, et al. Menopause and the risk of coronary heart disease in women. N Engl J Med. 1987;316(18):1105–1110. doi: 10.1056/NEJM198704303161801.
- McGill HC, Jr, Stern MP. Sex and atherosclerosis. Atheroscler Rev. 1979;4:157–242.
- Meng Q, Li Y, Ji T, et al. Estrogen prevent atherosclerosis by attenuating endothelial cell pyroptosis via activation of estrogen receptor α-mediated autophagy. J Adv Res. 2021;28:149–164. doi: 10.1016/j.jare.2020.08.010.
- Lv Y, Zhang S, Weng X, et al. Estrogen deficiency accelerates postmenopausal atherosclerosis by inducing endothelial cell ferroptosis through inhibiting NRF2/GPX4 pathway. Faseb J. 2023;37(6):e22992. doi: 10.1096/fj.202300083R.
- Rossouw JE, Anderson GL, Prentice RL, et al. Risks and benefits of estrogen plus progestin in healthy postmenopausal women: principal results from the women’s health initiative randomized controlled trial. JAMA. 2002;288(3):321–333. doi: 10.1001/jama.288.3.321.
- Anderson GL, Limacher M, Assaf AR, et al. Effects of conjugated equine estrogen in postmenopausal women with hysterectomy: the women’s health initiative randomized controlled trial. JAMA. 2004;291(14):1701–1712. doi: 10.1001/jama.291.14.1701.
- Ito F, Tatsumi H, Mori T, et al. Medroxyprogesterone acetate enhances monocyte endothelial interaction under flow conditions by stimulating the expression of cell adhesion molecules. J Clin Endocrinol Metab. 2014;99(6):2188–2197. doi: 10.1210/jc.2013-2925.
- Ito F, Mori T, Tarumi Y, et al. Equilin in conjugated equine estrogen increases monocyte-endothelial adhesion via NF-κB signaling. PLoS One. 2019;14(1):e0211462. doi: 10.1371/journal.pone.0211462.
- Løkkegaard E, Andreasen AH, Jacobsen RK, et al. Hormone therapy and risk of myocardial infarction: a national register study. Eur Heart J. 2008;29(21):2660–2668. doi: 10.1093/eurheartj/ehn408.
- Fournier A, Berrino F, Clavel-Chapelon F. Unequal risks for breast cancer associated with different hormone replacement therapies: results from the E3N cohort study. Breast Cancer Res Treat. 2008;107(1):103–111. doi: 10.1007/s10549-007-9523-x.
- Tatsumi H, Kitawaki J, Tanaka K, et al. Lack of stimulatory effect of dienogest on the expression of intercellular adhesion molecule-1 and vascular cell adhesion molecule-1 by endothelial cell as compared with other synthetic progestins. Maturitas. 2002;42(4):287–294. doi: 10.1016/s0378-5122(02)00157-3.
- Otsuki M, Saito H, Xu X, et al. Progesterone, but not medroxyprogesterone, inhibits vascular cell adhesion molecule-1 expression in human vascular endothelial cells. Arterioscler Thromb Vasc Biol. 2001;21(2):243–248. doi: 10.1161/01.atv.21.2.243.
- Groh LA, Verel DE, van der Heijden CDCC, et al. Immune modulatory effects of progesterone on oxLDL-induced trained immunity in monocytes. J Leukoc Biol. 2022;112(2):279–288. doi: 10.1002/JLB.3AB1220-846R.
- Banno K, Kisu I, Yanokura M, et al. Progestin therapy for endometrial cancer: the potential of fourth-generation progestin (review). Int J Oncol. 2012;40(6):1755–1762. doi: 10.3892/ijo.2012.1384.
- Grandi G, Mueller M, Bersinger NA, et al. Does dienogest influence the inflammatory response of endometriotic cells? A systematic review. Inflamm Res. 2016;65(3):183–192. doi: 10.1007/s00011-015-0909-7.
- Ichioka M, Mita S, Shimizu Y, et al. Dienogest, a synthetic progestin, down-regulates expression of CYP19A1 and inflammatory and neuroangiogenesis factors through progesterone receptor isoforms A and B in endometriotic cells. J Steroid Biochem Mol Biol. 2015;147:103–110. doi: 10.1016/j.jsbmb.2014.12.008.
- Grandi G, Mueller M, Bersinger N, et al. Progestin suppressed inflammation and cell viability of tumor necrosis factor-α-stimulated endometriotic stromal cells. Am J Reprod Immunol. 2016;76(4):292–298. doi: 10.1111/aji.12552.
- Mita S, Shimizu Y, Notsu T, et al. Dienogest inhibits toll-like receptor 4 expression induced by costimulation of lipopolysaccharide and high-mobility group box 1 in endometrial epithelial cells. Fertil Steril. 2011;96(6):1485–1489.e4. doi: 10.1016/j.fertnstert.2011.09.040.
- Gerszten RE, Luscinskas FW, Ding HT, et al. Adhesion of memory lymphocytes to vascular cell adhesion molecule-1-transduced human vascular endothelial cells under simulated physiological flow conditions in vitro. Circ Res. 1996;79(6):1205–1215. doi: 10.1161/01.res.79.6.1205.
- Yoshida N, Yoshikawa T, Nakamura Y, et al. Methylprednisolone inhibits neutrophil-endothelial cell interactions induced by interleukin-1β under flow conditions. Life Sci. 1997;60(25):2341–2347. doi: 10.1016/s0024-3205(97)00290-7.
- Wang YX, Xiang C, Liu B, et al. A multi-component parallel-plate flow chamber system for studying the effect of exercise-induced wall shear stress on endothelial cells. Biomed Eng Online. 2016;15(Suppl 2):154. doi: 10.1186/s12938-016-0273-z.
- Libby P. Inflammation in atherosclerosis. Nature. 2002;420(6917):868–874. doi: 10.1038/nature01323.
- Burns MP, DePaola N. Flow-conditioned HUVECs support clustered leukocyte adhesion by co-expressing ICAM-1 and E-selectin. Am J Physiol Heart Circ Physiol. 2005;288(1):H194–204. doi: 10.1152/ajpheart.01078.2003.
- Pateras I, Giaginis C, Tsigris C, et al. NF-κB signaling at the crossroads of inflammation and atherogenesis: searching for new therapeutic links. Expert Opin Ther Targets. 2014;18(9):1089–1101. doi: 10.1517/14728222.2014.938051.
- Xu K, Saaoud F, Yu S, et al. Monocyte adhesion assays for detecting endothelial cell activation in vascular inflammation and atherosclerosis. Methods Mol Biol. 2022;2419:169–182. doi: 10.1007/978-1-0716-1924-7_10.
- Luscinskas FW, Gerszten RE, Garcia-Zepeda EA, et al. C-C and C–X-C chemokines trigger firm adhesion of monocytes to vascular endothelium under flow conditions. Ann N Y Acad Sci. 2000;902(1):288–293. doi: 10.1111/j.1749-6632.2000.tb06324.x.
- Haraldsen G, Kvale D, Lien B, et al. Cytokine-regulated expression of E-selectin, intercellular adhesion molecule-1 (ICAM-1), and vascular cell adhesion molecule-1 (VCAM-1) in human microvascular endothelial cells. J Immunol. 1996;156(7):2558–2565. doi: 10.4049/jimmunol.156.7.2558.
- Zielińska KA, Van Moortel L, Opdenakker G, et al. Endothelial response to glucocorticoids in inflammatory diseases. Front Immunol. 2016;7:592. doi: 10.3389/fimmu.2016.00592.
- Deshmane SL, Kremlev S, Amini S, et al. Monocyte chemoattractant protein-1 (MCP-1): an overview. J Interferon Cytokine Res. 2009;29(6):313–326. doi: 10.1089/jir.2008.0027.
- Ikeda U. Inflammation and coronary artery disease. Curr Vasc Pharmacol. 2003;1(1):65–70. doi: 10.2174/1570161033386727.
- Seino Y, Ikeda U, Takahashi M, et al. Expression of monocyte chemoattractant protein-1 in vascular tissue. Cytokine. 1995;7(6):575–579. doi: 10.1006/cyto.1995.0078.
- Köhler G, Göretzlehner G, Brachmann K. Lipid metabolism during treatment of endometriosis with the progestin dienogest. Acta Obstet Gynecol Scand. 1989;68(7):633–635. doi: 10.3109/00016348909013283.
- Liu Y, Gong H, Gou J, et al. Dienogest as a maintenance treatment for endometriosis following surgery: a systematic review and meta-analysis. Front Med (Lausanne). 2021;8:652505. doi: 10.3389/fmed.2021.652505.