Abstract
Objective
To investigate whether the Anti-Müllerian Hormone (AMH), an ovarian hormone belonging to the Transforming Growth Factor β superfamily, may represent a possible candidate for use as a bone anabolic factor.
Methods
We performed in vitro studies on Human Osteoblasts (HOb) to evaluate the expression and the functionality of AMHRII, the AMH receptor type-2, and investigate the effects of exogenous AMH exposure on osteogenic gene expression and osteoblast functions.
Results
We reported the first evidence for the expression and functionality of AMHRII in HOb cells, thus suggesting that osteoblasts may represent a specific target for exogenous AMH treatment. Furthermore, the exposure to AMH exerted a stimulatory effect on HOb cells leading to the activation of osteogenic genes, including the upregulation of osteoblastic transcription factors such as RUNX and OSX, along with increased deposition of mineralized nodules.
Conclusion
Our findings proved interesting clues on the stimulatory effects of AMH on mature osteoblasts expressing its specific receptor, AMHRII. This study may therefore have translation value in opening the perspective that AMH may be an effective candidate to counteract the bone loss in osteoporotic patients by selectively targeting osteoblast with minimal off-target effect.
Introduction
Bone is a highly dynamic tissue undergoing constant remodeling. Its turnover is tightly regulated by a cross-talk between bone-forming osteoblasts and bone-resorbing osteoclasts [Citation1,Citation2].
The strict balance between bone resorption and formation is critical for the maintenance of bone mass and involves several cytokines, hormones, and signaling pathways [Citation3].
An imbalance in the bone remodeling process due to excessive activity of osteoclasts or impaired osteoblast activity/differentiation may lead to pathological conditions characterized by low bone mass or density [Citation4].
Osteoporosis is the most common metabolic bone disease currently affecting more than 200 million people worldwide [Citation5]. It results in a progressive loss of bone mineral density with deterioration of the bone structure, increased bone fragility, and risk of fractures [Citation6]. Osteoporotic fractures, the most devastating consequence of this disease, severely impact the quality of life of the patients, thereby representing an important public health problem [Citation7,Citation8]. In particular, women are more likely to develop osteoporosis than men because of hormonal changes occuring during menopause. It has been widely observed that estrogen deficiency after menopause leads to an imbalance in bone turnover favoring an increase in osteoclastic bone resorption, with negative effects on bone density [Citation9].
Antiresorptive agents (e.g. bisphosphonates, denosumab, estrogens, and raloxifene) able to suppress osteoclastic-mediated bone breakdown represented for a long time the standard therapeutic drugs for postmenopausal osteoporosis [Citation10]. However, these molecules have a poor ability to stimulate new bone synthesis, which is essential for the treatment of patients with advanced bone loss. More recently, new therapies based on anabolic agents stimulating bone remodeling through the activation of osteoblasts are therefore under investigation [Citation11].
Despite the great advances in drug discovery and the wide availability of treatments, some restrictions in the use of pharmacological agents to treat osteoporosis still exist and further studies are needed to develop target-specific drugs for osteoporosis to overcome the side effects of long-term treatments mainly due to their pleiotropic effects in both skeletal and non-skeletal tissues [Citation12].
Among the molecular factors involved in normal and pathological osteogenesis, particular attention has been addressed to the growth factors belonging to the Transforming Growth Factor β (TGF-β) superfamily. Many members of this family are involved in a vast majority of cellular processes and thereby exhibit versatile roles in the body, including the function to mediate the balance between bone formation and resorption through directly or indirectly regulating osteogenesis and osteoclastogenesis [Citation13]. Among the TGB-β proteins, the Bone Morphogenetic Proteins (BMPs) have been well-defined and recognized as essential for the differentiation of mesenchymal stem cells into osteoblasts, especially via SMAD 1/5/8 signaling pathway which induces the expression of key transcription factors such as RUNX2, and Osterix [Citation14–17].
Among the TGF-β family members, the Anti-Müllerian Hormone (AMH) is an important regulator of reproductive organ differentiation and ovarian follicle development which shares type I receptors and downstream SMAD proteins (1/5/8) the with BMP pathway [Citation18,Citation19]. Interestingly, a recent analysis of genes up-regulated in males during Müllerian duct (MD) regression identified the BMP-induced gene Osterix as a downstream effector of AMH signaling during MD regression [Citation20], leading us to focus our attention on the potential role of AMH in the modulation of factors regulating bone homeostasis.
To date, the function of AMH as well as the presence of its receptor (AMHRII) remains restricted to the reproductive organs [Citation21] and its involvement in bone formation is unknown.
Recent evidence also showed that osteoblast and osteoclast do not express the AMH gene, however, AMH exogenous treatment was found to inhibit osteoclast differentiation by suppressing expression levels of osteoclast differentiation markers such as c-Fos, NFATc1 and TRAP [Citation22], leading us to deepen the role of AMH and explore the presence of AMHRII in bone tissue to evaluate whether bone-forming osteoblast may represent a specific target for exogenous AMH treatment.
Materials and methods
Cell culture and treatments
The human fetal osteoblast cell line (hFOB1.19) was purchased from ATCC and maintained at 37 °C with 5% carbon dioxide in a 1:1 mixture of DMEM/Ham’s F-12 medium supplemented with 10% fetal bovine serum (FBS), 1% glutamine, 100 U/mL penicillin, 100 μg/mL streptomycin and 0.25 μg/ml amphotericin B (basal medium).
Human granulosa cells (hGCs), used as a positive control of AMH type II receptor protein expression, were isolated from ovarian follicles of women undergoing oocyte retrieval for IVF protocol at the Department of Obstetrics and Gynecology of Modena University Hospital. All patients consented to the donation of GCs. Granulosa cells were maintained in DMEM medium supplemented with 5 % FBS, 1% glutamine, 100 U/mL penicillin, 100 μg/mL streptomycin and 0.25 μg/ml amphotericin B. Before performing experiments, hGCs were cultured for 6 days to eliminate the hormonal effects suffered by the patient during the pre-IVF stimulation protocol.
Gibco (Thermo Fisher Scientific, Waltham, MA, USA) supplied all reagents for cell cultures.
All the experiments were performed by seeding cells in basal medium two days before the experiment. On the day of the assay, growth medium was replaced with a fresh medium to expose cells to the appropriate experimental conditions. For examination of osteogenic gene and SMADs expression, cells were incubated with the recombinant human AMH (rhAMH, R&D Systems, Minneapolis, MN, USA) at 100 ng/ml in a serum-free basal medium. Cells treated with recombinant human 100 ng/ml BMP2 and human 10 ng/ml TGF-β1 (Gibco, Thermo Fisher Scientific, Waltham, MA, USA) were used as a positive and negative control of SMADs activation, respectively. To induce osteoblast-to-osteocyte differentiation and matrix mineralization, cells were cultured in an osteogenic medium (basal medium supplemented with 50 μg/ml L-ascorbic acid, 10−7 M dexamethasone and 10 mM β-glycerophosphate), both in the presence and absence of 100 ng/ml AMH. The medium was changed every three days for 14 days.
SDS-PAGE and western blot analysis
Cells at 70–80% of confluence were detached from the plate, washed with PBS 1X and resuspended in lysis buffer 1X (50 mM TrisEDTA, 150 mM NaCl, 0,1% SDS, 0,5% DOC, 0.1% TritonX-100) supplemented with the Protease and Phosphatase Inhibitor Cocktail (Thermo Fisher Scientific, Waltham, MA, USA). Protein concentration was measured by BCA Protein Assay Kit (Thermo Fisher Scientific, Waltham, MA, USA), according to the manufacturer’s protocol. Samples containing equal amounts of proteins (30 μg) were run on 4-12% SDS-polyacrylamide gel at 140 V and transferred onto a nitrocellulose membrane at 100 V for 75 min. Membranes were blocked for 2 h in 2% of BSA in 1X Tris-buffered Saline, 0.05% Tween® 20 Detergent (TBST), and then incubated with the antibodies overnight at 4 °C. Primary antibodies used were: mouse anti-AMHRII antibody (1:800, Novus Biologicals, USA, NBP1-05491), rabbit anti-pSMAD 1/5 (1:500, Cell Signaling Technology, Danvers, MA, USA, BK9516S), rabbit anti-pSMAD 3 (1:500, Cell Signaling Technology, Danvers, MA, USA, BK9520T) and rabbit anti-vinculin (1:1000, Thermo Fisher Scientific, Waltham, MA, USA, #700062). After overnight incubation, membranes were washed with TBST and then incubated for 2 h at room temperature with the secondary HRP antibodies (goat anti-mouse and goat anti-rabbit, 1:10000, Thermo Fisher Scientific, Waltham, MA, USA, G21040 and G21234 respectively). Signals were detected by an ECL immunodetection system (Amersham Corp, Arlington Heights, IL, USA) following the manufacturer’s instruction. The relative band intensity was captured by ChemiDocTM XRS 2015 (Bio-Rad Laboratories, CA, USA), and densitometric analysis was performed using Image Lab software and normalized to housekeeping.
Cell growth and viability
Cellular growth was assessed using the Trypan Blue exclusion test [Citation23] by seeding 2x105 cells in triplicate in basal medium. Cell culture media change was performed two days after seeding, which is the needed time to let cells properly adhere to the plate before exposing them to 100 AMH for up to 72 h. Adherent cells were trypsinized, collected and counted with Trypan Blue every 24 h without changing the medium. The cytotoxicity of the prolonged AMH exposure was also assessed using the CCK-8 assay (Sigma-Aldrich). Cells were seeded into 96-well plates (2000 cells/well), incubated overnight and then treated with or without AMH for 24, 48 and 72 h. 10 μl CCK-8 solution was added to each well followed by a 4-h incubation at 37 ̊C. Next, the OD value foreach well was read at a wavelength of 450 nm to determine cell viability using a microplate reader.
Total RNA preparation, RT-PCR and Real-time PCR
Total RNA was extracted using TRI-Reagent (Sigma-Aldrich, St. Louis, MO, USA). RNA concentration and quality were detected using the Nanodrop ND-1000 (Thermo Fisher Scientific, Waltham, MA, USA). Total RNA was reverse transcribed using the High-Capacity RNA-to-cDNA™ Kit (Thermo Fisher Scientific, Waltham, MA, USA) according to the manufacturer’s instructions. Expression of human Anti-Mullerian Hormone Receptor Type 2 (AMHRII) was evaluated by semiquantitative RT-PCR using DreamTaq Green PCR Master Mix (2X) (Thermo Fisher Scientific, Waltham, MA, USA). For each PCR reaction, 15 μl samples were loaded in a 2% agarose gel. Ribosomal protein S7 (RpS7) was used as the housekeeping gene.
The presence of transcripts for bone differentiation markers was evaluated by SYBR Green Real-Time PCR on an Applied Biosystems Real-Time PCR System using PowerTrack™ SYBR Green Master Mix (Thermo Fisher Scientific, Waltham, MA, USA), following the manufacturer’s recommendations. Each sample was normalized to its RpS7 content. Normalized gene expression was evaluated using the 2−ΔΔCt method and expressed as fold increase over its unstimulated sample (basal level) [Citation24]. Primer’s specificity was confirmed by melting curves. The primers utilized are described in .
Table 1. Primer sequences used in RT-PCR.
Alizarin Red staining of calcium deposition/mineralization assay
HOb cells were stained with Alizarin Red S on day 14 of treatment for assessing the mineralized nodules. The osteogenic medium was removed, and the cell layers were rinsed three times with PBS and fixed with 10% neutral buffered formalin (Sigma-Aldrich, St. Louis, MO, USA) at room temperature for 15 min. Then, cell layers were washed twice with deionized water. The fixed cells were incubated with 40 mM Alizarin red-S pH 4.0 − 4.5 (Sigma-Aldrich, St. Louis, MO, USA) at room temperature for 20 min. After staining, cell layers were washed 4 times with an excess of deionized water and examined using Olympus BX53 inverted microscope. Pictures were acquired at a magnification 10X. To quantify Alizarin Red S retained minerals, stained cells were treated with 10% (v/w) cetylpyridinium chloride in Sodium Phosphate for 1 h to solubilize and release calcium-bound alizarin red into solution. The absorbance of the solution was read at 570 nm in triplicate.
Statistical analyses
Student’s t-test and one-way ANOVA test, followed by Student-Newman-Keuls’ post hoc test, were used to evaluate statistical significance within the different samples. The results were considered statistically significant at p < 0.05 (*), p < 0.01 (**) and p < 0.001 (***).
Results
Human osteoblasts express functional anti-Müllerian Hormone type II receptor
Before exploring the potential osteogenic effect of AMH, we investigated the presence of its specific type II receptor in human osteoblast cells. To this aim, AMHRII mRNA and protein expression were examined in HOb cells, using granulosa cells as a positive control for AMHRII expression. RT-PCR analysis detected AMHRII transcript in both cellular models without significant differences in mRNA expression levels (). Interestingly, Western blotting analysis also revealed the presence of a robust AMHRII protein expression in HOb cells that displayed a 25% higher AMHRII/GAPDH densitometric ratio than granulosa cells (). To evaluate the AMHRII functionality, we determined whether the exposure to exogenous AMH was able to activate AMHRII-mediated signaling by assessing the changes following rhAMH treatments in levels of SMAD proteins (pSMAD-1/5), whose phosphorylation is known to mediate the AMH signal transduction. Interestingly, an increase in pSMAD-1/5 levels was observed after a short time exposure to AMH (from 30 min until 2 h) indicating that AMH binding was able to activate its receptor. As expected, AMH treatment did not induce SMAD3 protein phosphorylation in HOb cells, contrary to what was observed in osteoblast cells treated with TGF-β3. Remarkably, BMP2 treatment induced the same SMAD 1/5 activation pattern observed following AMH exposure, thus proving that AMH mediates its effects through the same SMAD proteins activated by BMPs in HOb cells too ().
Figure 1. (A) Representative RT-PCR analysis of AMHRII transcript expression in human fetal osteoblast cell line (HOb) and granulosa cells of three independent cell cultures. (B) Representative Western blot analysis of AMHRII protein expression in human fetal osteoblast cell line (HOb) and granulosa cells. Densitometric absorbance values from three independent experiments were averaged (± S.D) and were expressed as arbitrary units (a.u.). (C) Western blot analysis of pSMAD1/5 expression in the human fetal osteoblast cell line (hob). in the figure was reported a representative blot. Densitometric absorbance values from three separate experiments were averaged (±S.D) and were expressed as arbitrary units (a.u.). Vinculin was used as internal control. ** p < 0.01; ***p < 0.001 versus respective control (CTRL). (D) Western blot analysis of pSMAD3 expression in the human fetal osteoblast cell line (hob). in the figure was reported a representative blot. Densitometric absorbance values from three separate experiments were averaged (±S.D) and were expressed as arbitrary units (a.u.). Vinculin was used as internal control. * p < 0.05 versus respective control (CTRL).
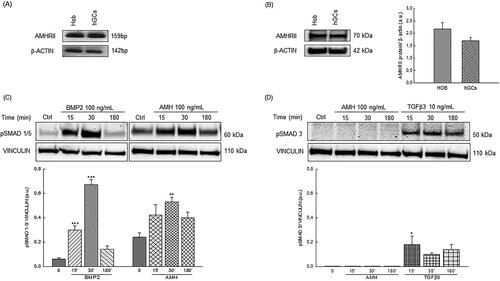
AMH did not affect osteoblasts viability and proliferation
The effect of prolonged AMH exposure on osteoblast growth and viability was evaluated every 24 h up to 72 h by performing the Trypan blue dye exclusion test and the CCK-8 assay. As reported in , cells treated with 100 ng/ml AMH showed no significant differences in terms of cell proliferation and viability when compared to the control, leading us to affirm that AMH treatment is not cytotoxic for human osteoblasts.
Figure 2. (A) Effect of AMH (100 ng/mL) at different times of treatment on cellular growth evaluated by trypan blue staining. Data are the mean ± S.D of three independent experiments performed in triplicate and were expressed as fold increase respect to the number of cells present at time 0 arbitrarily set to 1. **p < 0.01; ***p < 0.001. (B) Optical density of HOb cells treated for 24, 48 and 72 h with 100 ng/mL AMH after CCK-8 cytotoxicity assay. Data are the mean ± S.D of three independent experiments performed in triplicate. (C) Levels of expression of RUNX2, OX, OPN, and OC genes were analyzed by Real time-PCR. The levels of the transcripts were normalized with the housekeeping gene (RPS7). Data are the mean ± S.D of three independent experiments performed in triplicate and were expressed as fold increase respect to the respective control set equal to 1. *p < 0.05; **p < 0.01 versus CTRL.
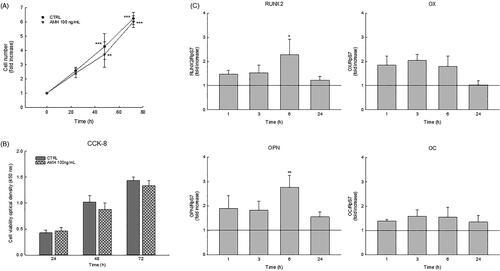
AMH positively modulates osteogenic genes in HOb cells
To evaluate the anabolic role of AMH in osteoblast differentiation, we assessed AMH action on crucial osteoblastic transcription factors such as RUNX2 and OSX and their downstream genes, osteopontin (OPN) and osteocalcin (OC), by culturing HOb cells with 100 ng/mL AMH for 1, 3, 6 and 24 h. The real-time PCR analysis showed that the mRNA expression of all genes analyzed was significantly increased after 1h of AMH exposure until 6 h of treatment, whereas the expression levels gradually returned to the control level after 24 h of AMH treatment ().
AMH treatment favors matrix mineralization in HOb cells
To determine whether AMH may promote the in vitro formation of mineralized nodules, HOb cells were cultured with the osteogenic medium in the absence or presence of AMH (100 ng/mL). We performed the real-time PCR analysis of mRNA levels of Alkaline phosphatase (ALP), an essential enzyme involved in the early stage of bone mineralization, during the first days of culture in the osteogenic differentiation medium. Intriguingly, as reported in , the presence of AMH induced a 70% increase in ALP gene expression in HOb cells cultured for 5 days in the osteogenic medium.
Figure 3. (A) Relative mRNA expression level by Real time-PCR of alkaline phosphatase (ALP) cultured in osteogenic medium both in the presence and in the absence of 100 ng/mL AMH. (B) Alizarin Red staining of calcium deposition in human fetal osteoblast cell line (HOb) plate view (upper) and microscopic view (lower) of the staining on day 14 of AMH (100 ng/mL) treatment. Intensity of Alizarin Red stains, which refers to quantity of mineralization process, was examined. Graph data are shown as mean ± S.D of three independent experiments. ***p < 0.001 versus control (CTRL).
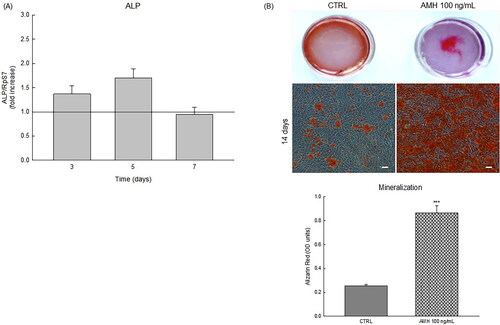
After 14 days of treatments, we evaluated bone nodule development by staining cells with Alizarin Red dye. The assay showed mineralized calcium nodules labeled as red spots (). Interestingly, AMH treatment raised the amounts of calcium in the matrix compared to the control condition. Consistently, quantification of Alizarin Red showed that AMH significantly promoted mineral deposition by osteoblasts, nearly up to 400% of the control, thus suggesting that it can stimulate bone cell differentiation and bone matrix formation in HOb cells.
Discussion
The enhancement of osteoblast proliferation and differentiation employing anabolic drugs specifically targeting the bone-forming osteoblasts may represent an effective therapeutic strategy to ameliorate both the quantity and the quality of bone tissue in osteoporotic patients [Citation11]. So far, various hormones, growth factors, and cytokines regulating the bone differentiation and mineralization have been identified. In particular, multiple growth factors belonging to the transforming growth factor β superfamily, especially the BMP proteins such as BMP-2, have a widely recognized role in inducing osteoblastic differentiation and bone deposition. However, their clinical use is limited due to their off-target extraskeletal effects and further studies are ongoing to identify more specific anabolic molecules [Citation25].
To deepen the role of TGF-β factors in bone formation by osteoblasts, we focused our attention on AMH, another member of this family whose function in bone remodeling has not been investigated. The Anti-Müllerian hormone is rather well known for its role as a regulator of reproductive organ differentiation and ovarian follicle development [Citation18].
Unlike BMP-2 and the other TGF-β factors, whose signaling pathways are functioning in numerous tissue and organs, AMH has long been thought to be a tissue-specific TGF-β growth factor since its expression, as well as the presence of its specific receptor type II, is mainly restricted to the reproductive organs. In these tissues, AMH has been reported to activate a BMP-like pathway mediated by BMP type I receptors such as activin-like kinase (ALK)2, ALK3, and ALK6 to trigger the SMADs 1,5, and 8 pathways [Citation26,Citation27].
These premises prompted us to investigate whether AMH, as other TGF-β superfamily members do, may promote osteoblast differentiation and bone formation by activating BMP-like pathways. Since a unique AMH type II receptor has been shown to be able to transduce the AMH signal, we first investigated the presence of its specific receptor in human osteoblast cells. Interestingly, the present study revealed for the first time the presence of a strong mRNA and protein expression of AMHRII in HOb cells, thus indicating that osteoblasts may represent a specific target for exogenous AMH treatments. The increase in SMAD 1/5 protein levels observed after rhAMH exposure, demonstrated that the receptor binding interaction of AMH and AMHRII was able to activate the SMAD-mediated AMH signaling pathway. Furthermore, since the signaling pathway activated by rhAMH induced the same SMAD 1/5 activation pattern observed after rBMP-2 exposure in HOb cells [Citation28], we concluded that AMH activates a BMP-like pathway also in these cells.
During the bone formation process, osteoblasts increase the expression of specific genes involved in extracellular matrix formation and mineral deposition [Citation29,Citation30]. Interestingly, we reported that AMH treatment may favor this process by upregulating mRNA levels of crucial osteoblastic transcription factors such as RUNX2 and OSX and extracellular matrix proteins including OPN and OC. Consistently, the prolonged exposure to AMH also promoted matrix mineralization in HOb cells, as demonstrated by both increased ALP gene expression and the greater amount of mineralized nodules observed in AMH-treated osteoblasts compared to untreated controls.
In conclusion, our findings proved for the first time interesting clues on the stimulatory effects of AMH on mature osteoblasts expressing its specific receptor, AMHRII, which signals via a BMP-like pathway, thus leading to osteogenic gene activation and deposition of mineralized calcium nodules without affecting cellular viability and proliferation. This study has therefore translation value in opening the perspective that AMH may be an effective candidate to counteract the bone loss in osteoporotic patients by developing a new therapy selectively targeting osteoblast with minimal off-target effect. In this view, functional studies on the action mechanism of AMH in the other bone cells will be essential to understand the complex mechanism by which it may influence bone homeostasis and pave the way for further studies.
Attestation statement
Data regarding any of the subjects in the study has not been previously published unless specified. Data will be made available to the editors of the journal for review or query upon request.
Disclosure statement
No potential conflict of interest was reported by the authors.
Additional information
Funding
References
- Matsuo K, Irie N. Osteoclast–osteoblast communication. Arch Biochem Biophys. 2008;473(2):1–6. doi:10.1016/j.abb.2008.03.027.
- Datta HK, Ng WF, Walker JA, et al. The cell biology of bone metabolism. J Clin Pathol. 2008;61(5):577–587. doi:10.1136/jcp.2007.048868.
- Ponzetti M, Rucci N. Osteoblast differentiation and signaling: established concepts and emerging topics. Int J Mol Sci. 2021;22(13):6651. doi:10.3390/ijms22136651.
- Feng X, McDonald JM. Disorders of bone remodeling. Annu Rev Pathol. 2011;6(1):121–145. doi:10.1146/annurev-pathol-011110-130203.
- Shen Y, Huang X, Wu J, et al. The global burden of osteoporosis, low bone mass, and its related fracture in 204 countries and territories, 1990-2019. Front Endocrinol. 2022;13:882241. doi:10.3389/fendo.2022.882241.
- Jang S, Park C, Jang S, et al. Medical service utilization with osteoporosis. Endocrinol Metab. 2010;25(4):326–339. doi:10.3803/EnM.2010.25.4.326.
- Johnell O, Kanis JA. An estimate of the worldwide prevalence and disability associated with osteoporotic fractures. Osteoporos Int. 2006;17(12):1726–1733. doi:10.1007/s00198-006-0172-4.
- Johnell O, Kanis J. Epidemiology of osteoporotic fractures. Osteoporos Int. 2005;16(S02):S3–S7. doi:10.1007/s00198-004-1702-6.
- Avioli LV. Senile and postmenopausal osteoporosis. Adv Intern Med. 1976;21:391–415.
- Eastell R. Treatment of postmenopausal osteoporosis. N Engl J Med. 1998;338(11):736–746. doi:10.1056/NEJM199803123381107.
- Marie PJ, Kassem M. Osteoblasts in osteoporosis: past, emerging, and future anabolic targets. Eur J Endocrinol. 2011;165(1):1–10. doi:10.1530/EJE-11-0132.
- Khosla S, Hofbauer LC. Osteoporosis treatment: recent developments and ongoing challenges. Lancet Diabetes Endocrinol. 2017;5(11):898–907. doi:10.1016/S2213-8587(17)30188-2.
- Jann J, Gascon S, Roux S, et al. Influence of the TGF-β superfamily on osteoclasts/osteoblasts balance in physiological and pathological bone conditions. Int J Mol Sci. 2020;21(20):7597. doi:10.3390/ijms21207597.
- Liu Q, Li M, Wang S, et al. Recent advances of osterix transcription factor in osteoblast differentiation and bone formation. Front Cell Dev Biol. 2020;8:601224. doi:10.3389/fcell.2020.601224.
- Nakashima K, Zhou X, Kunkel G, et al. The novel zinc finger-containing transcription factor osterix is required for osteoblast differentiation and bone formation. Cell. 2002;108(1):17–29. doi:10.1016/s0092-8674(01)00622-5.
- Matsumoto Y, Otsuka F, Hino J, et al. Bone morphogenetic protein-3b (BMP-3b) inhibits osteoblast differentiation via Smad2/3 pathway by counteracting Smad1/5/8 signaling. Mol Cell Endocrinol. 2012;350(1):78–86. doi:10.1016/j.mce.2011.11.023.
- Matsubara T, Kida K, Yamaguchi A, et al. BMP2 regulates osterix through Msx2 and Runx2 during osteoblast differentiation. J Biol Chem. 2008;283(43):29119–29125. doi:10.1074/jbc.M801774200.
- Durlinger AL, Visser JA, Themmen APN. Regulation of ovarian function: the role of anti-Müllerian hormone. Reproduction. 2002;124(5):601–609. doi:10.1530/rep.0.1240601.
- Josso N, di Clemente N. Transduction pathway of anti-Müllerian hormone, a sex-specific member of the TGF-β family. Trends Endocrinol Metab. 2003;14(2):91–97. doi:10.1016/s1043-2760(03)00005-5.
- Mullen RD, Wang Y, Liu B, et al. Osterix functions downstream of anti-Müllerian hormone signaling to regulate müllerian duct regression. Proc Natl Acad Sci U S A. 2018;115(33):8382–8387. doi:10.1073/pnas.1721793115.
- Baarends WM, Van Helmond MJ, Post M, et al. A novel member of the transmembrane serine/threonine kinase receptor family is specifically expressed in the gonads and in mesenchymal cells adjacent to the mullerian duct. Development. 1994;120(1):189–197. doi:10.1242/dev.120.1.189.
- Kim JH, Yang YR, Kwon KS, et al. Anti-Müllerian hormone negatively regulates osteoclast differentiation by suppressing the receptor activator of nuclear factor-κB ligand pathway.J Bone Metab. 2021;28(3):223–230. (doi:10.11005/jbm.2021.28.3.223.
- Strober W. Trypan blue exclusion test of cell viability. Curr Protoc Immunol. 1997;21(1):A-3B.
- Livak KJ, Schmittgen TD. Analysis of relative gene expression data using real-time quantitative PCR and the 2− ΔΔCT method. Methods. 2001;25(4):402–408. doi:10.1006/meth.2001.1262.
- Rodan GA, Martin TJ. Therapeutic approaches to bone diseases. Science. 2000;289(5484):1508–1514. doi:10.1126/science.289.5484.1508.
- Howard JA, Hart KN, Thompson TB. Molecular mechanisms of AMH signaling. Front Endocrinol. 2022;13:927824. doi:10.3389/fendo.2022.927824.
- Cate RL. Anti-Müllerian hormone signal transduction involved in müllerian duct regression. Front Endocrinol. 2022;13:905324. doi:10.3389/fendo.2022.905324.
- Zou ML, Chen ZH, Teng YY, et al. The smad dependent TGF-β and BMP signaling pathway in bone remodeling and therapies. Front Mol Biosci. 2021;8:593310. 5doi:10.3389/fmolb.2021.593310.
- Murshed M. Mechanism of bone mineralization. Cold Spring Harb Perspect Med. 2018;8(12):a031229. doi:10.1101/cshperspect.a031229.
- Komori T. Regulation of osteoblast differentiation by transcription factors. J Cell Biochem. 2006;99(5):1233–1239. doi:10.1002/jcb.20958.