Abstract
Objective
Comparison of hormonal, metabolic and inflammatory markers of glutathione with metformin and Diane-35 in a rat model of PCOS induced by dehydroepiandrosterone.
Methods
Twenty-five female rats were randomized into four groups. Group 1 was administered a subcutaneous dose of 0.2 ml saline/day. Group 2 was given 0.2 ml of 1% carboxymethyl cellulose (CMC)/day orally for 28 days. A PCOS model was established with DHEA in rats. Group 3 was given 4.5 mg/kg/day of Diane-35 orally dissolved in 1% CMC for 28 days. Group 4 was given 300 mg/kg/day of metformin orally dissolved in 1 ml of saline for 28 days, and Group 5 was administered 100 mg/kg of glutathione intraperitoneally on days 35, 42, and 49. On day 56, the rats were sacrificed. Serum markers and follicle count were examined.
Results
Serum IL-6, hs-CRP, insulin, testosterone, SHBG, and MDA values were significantly lower in the glutathione group than in the PCOS group (p = 0.0006, p = 0.023, p = 0.0082, p = 0.0007, p = 0.0048, and p < 0.0001, respectively).
The number of all follicles was similar between the control and glutathione groups (p < 0.05). When we compared the other groups with the PCOS group, the number of primary, secondary, atretic, and cystic follicles was significantly lower in the metformin and glutathione groups. The number of primordial and antral follicles was significantly higher than in the PCOS group.
Conclusions
Glutathione plays anti-inflammatory and antioxidant roles, similar to metformin, by lowering serum IL-6, insulin, testosterone, CRP, and MDA levels; decreasing atretic/cystic follicle count; and improving antral follicle count and folliculogenesis in PCOS patients.
Keywords:
Introduction
Polycystic ovary syndrome (PCOS), which is one of the most common clinical issues in reproductive-age women, is characterized by hyperandrogenism, ovulatory (and menstrual) dysfunction, and polycystic ovarian morphology according to the Rotterdam Criteria (2003) [Citation1,Citation2]. Moreover, as well as being a gynecologic condition, PCOS is a metabolic and multifactorial disorder caused by genetic and environmental factors [Citation3]. It is a disorder grounded in neuroendocrine irregularities, distinguished by an elevated gonadotropin-releasing hormone (GnRH) level and an increased ratio of luteinizing hormone (LH) to follicle-stimulating hormone (FSH). The increase in LH to FSH levels is believed to initiate PCOS pathogenesis. This hormonal imbalance promotes theca cell proliferation and androgen secretion. Reduced FSH limits aromatase production by granulosa cells, hindering androgen-to-estrogen conversion and resulting in excess ovarian androgen. This excess contributes to hyperandrogenemia symptoms such as hirsutism, acne, and alopecia, and it disrupts follicular development, leading to anovulation [Citation4]. Most affected individuals, especially women with hyperandrogenism, have metabolic abnormalities, primarily insulin resistance, and compensatory hyperinsulinemia [Citation5]. This syndrome affects between 5% and 20% of reproductive-age women. Those with PCOS are more likely to develop metabolic abnormalities, type 2 diabetes mellitus (T2DM), infertility, obstetric problems, endometrial and ovarian cancer, hypertension, hyperlipidemia, cardiovascular and cerebrovascular events, venous thromboembolism, and mental disorders. In 2004, the economic consequences of PCOS in the United States alone exceeded $4 billion, excluding the costs associated with related obstetric problems, T2DM, and other illnesses [Citation6].
Oxidative stress, which has been linked to the pathophysiology of cardiovascular disorders, has also been found in women with PCOS [Citation4,Citation7]. The imbalance between oxidants and antioxidants, as well as the excessive generation of reactive oxygen species (ROS), is referred to as oxidative stress. According to recent research, oxidative stress may have a role in the development of PCOS via numerous mechanisms and may be a potential inducer of PCOS pathogenesis. Insulin resistance and hyperglycemia, both of which are strongly linked to PCOS, contribute to oxidative stress [Citation8]. In theca cells, hyperinsulinemia stimulates androgen production, resulting in androgen-mediated inhibition of sex hormone-binding globulin (SHBG) production in hepatocytes [Citation9]. Lipid peroxidation is another source of oxidative stress. In women with PCOS, levels of indicators that may suggest lipid peroxidation, such as thiobarbituric acid-reactive compounds, oxidized low-density lipoprotein, and malondialdehyde (MDA), are much higher than in controls. Furthermore, serum lipid peroxide levels are elevated in women with PCOS [Citation10]. Blood MDA levels, a known marker of oxidative stress, have been demonstrated to be considerably higher, regardless of weight, in women with PCOS [Citation7]. Oxidative stress also induces autophagy, which is normally an essential process for folliculogenesis; its persistent activation may lead to a harmful effect on the ovaries. An animal study demonstrated that treatment with clove (S. aromaticum) was associated with decreased autophagy activity in PCOS-induced rats [Citation11].
PCOS is also associated with low-grade inflammation and elevated inflammatory cytokines, which contribute to the syndrome’s development. Most investigations addressing the state of chronic low-grade inflammation in PCOS have focused on circulating C-reactive protein (C-CRP), TNF, interleukin-6 (IL-6), and IL-18 levels. Low-grade chronic inflammation, as measured by high C-CRP levels, is a risk factor for both coronary heart disease (CHD) and T2DM. Insulin resistance and hyperglycemia increase ROS and result in altered levels of inflammatory markers in PCOS patients [Citation12,Citation13].
Metformin and Diane-35 (2 mg cyproterone acetate and 35 μg ethinyl estradiol) are commonly used for the clinical treatment of PCOS to reduce serum androgen levels and regulate menstrual dysfunction, ovulation, glucose metabolism, and insulin resistance [Citation14]. Glutathione is a powerful antioxidant required for the control of disulfide bonds in proteins as well as the removal of electrophiles and oxidants [Citation15]. Studies have demonstrated that glutathione levels are low in PCOS patients [Citation7,Citation16]. The purpose of this study is to investigate how glutathione affects hormonal, metabolic, and inflammatory indicators in a dehydroepiandrosterone (DHEA)-induced PCOS rat models compared to metformin and Diane-35.
Materials and methods
Animals and treatments
DHEA-induced PCOS in rats was created with subcutaneous administration of DHEA at a dosage of 6 mg/100 g/day for 34 days. DHEA treatment is a widely used animal model for PCOS [Citation17]. The DHEA-induced PCOS model presents several characteristics analogous to human PCOS, including insulin resistance, impaired follicular maturation, anovulation, and hyperandrogenism [Citation18]. Group 1 (Control group) (n = 5) was administered a subcutaneous dose of 0.2 ml saline/day for the same amount of time as the PCOS group. PCOS was confirmed by unilateral oophorectomy in two rats on day 35. After a 10-day follow up for follicle evaluation in the ovaries and vaginal smear, all rats had lost their regular estrous cycles. Twenty-five (five rats per group) 21-day-old (weight 45–50 g) female Wistar albino rats were randomized into four groups after DHEA-induced PCOS was confirmed. Group 2 (PCOS group, n = 5) was given 0.2 ml of 1% carboxymethyl cellulose (CMC)/day orally for 28 days; Group 3 (n = 5) was given 4.5 mg/kg/day of Diane-35 orally dissolved in 1% CMC for 28 days; Group 4 (n = 5) was given 300 mg/kg/day of metformin orally dissolved in 1 ml of saline for 28 days; Group 5(n = 5) was administered 100 mg/kg of glutathione intraperitoneally on days 35, 42, and 49. The treatment durations were consistent with those in comparable studies [Citation19]. The interventions in each group are shown in . On day 56, the rats were sacrificed by intracardiac blood sampling for evaluation of serum markers of inflammation (high-sensitivity CRP [hs-CRP] and IL-6), testosterone, insulin, MDA, and SHBG levels. The ovaries were removed for the assessment of follicle count.
Ethical approval
This study was approved by the Experimental Animal Laboratory Ethics Committee of Bezmialem Vakif University (Approval Number: 2022/03).
Measurement of serum biomarkers
Serum levels of IL-6 (AGF Scientific, USA, cat#720267), testosterone (AGF Scientific, USA, cat#720991), insulin (AGF Scientific, USA, cat#720161), SHBG (AGF Scientific, USA, cat#720847), and MDA (AGF Scientific, USA, cat#720889) were measured using the ELISA method, with concentrations determined according to the kit manufacturers’ instructions.
Histological analysis and ovarian follicle count
A histologist performed a blind histopathologic examination for ovarian follicle count. Following 10% neutral formalin for 72 h fixation, the ovaries were rinsed with water, dehydrated gradually using various concentrations of alcohol (70%, 90%, 96%, and 100%), and cleaned with xylene. For the paraffin block preparation, the samples were submerged in paraffin at 60 °C and incubated overnight. Slides were prepared by cutting sections with a thickness of 5 μm from the paraffin blocks and staining with hematoxylin and eosin (H&E) for histomorphometric analysis. Follicle count was performed under a photomicroscope (Nikon Eclipse i5, Tokyo, Japan). Ten sections from each ovary were taken at 100-μm intervals to count the follicles. Follicles with an oocyte nucleus were scored and grouped as follows: primordial (if the oocyte was surrounded by a single layer of squamous granulosa cells); primary (if a single layer of cuboidal granulosa cells was observed); secondary (if they had multiple layers of cuboidal granulosa cells); antral (if an antrum was present in the granulosa cell layers); and atretic (if the oocyte was degenerated and multiple layers of pycnotic granulosa cells were observed) [Citation20].
Statistical analysis
A sample size and power calculation determined that sufficient statistical power required five rats for each group (power = 0.80, type 1 error = 0.05 and type 2 error = 0.20). The power calculation was based on serum concentrations of MDA reported by Furat Rencber et al. using the standard formula/program [Citation19]. The data were analyzed using GraphPad Prism 6.0(GraphPad Software, Inc., La Jolla, CA, USA) with one-way ANOVA followed by the Tukey test as a post-test. Insulin, testosterone, IL-6, MDA, SHBG and hs-CRP serum concentrations and follicle count results were evaluated statistically. All data were presented as mean ± standard error; p values were regarded as significant when they were less than 0.05 (* p < 0.05, ** p < 0.01, *** p < 0.001, ****, p < 0.0001).
Results
Serum IL-6, hs-CRP, insulin, testosterone, SHBG, and MDA values in all groups are shown in . Due to technical problems with sampling in the control group, we could not obtain optimal results for any of the serum biomarkers except testosterone. Statistically significant differences were found in terms of all parameters between the four groups(p < 0.05). When we analyzed subgroup differences, we found that serum IL-6 levels were significantly lower in the metformin and glutathione groups than in the PCOS group (metformin vs PCOS; 10.5 ± 4.02 vs 32.16 ± 6.97 ng/L, p < 0.0001; lutathione vs PCOS; 16.13 ± 1.3 vs 32.16 ± 6.97 ng/L, p = 0.0006, respectively) (, ). Likewise, insulin levels were significantly lower in the metformin and glutathione groups than in the PCOS group (metformin vs PCOS; 10.49 ± 4.71 vs 39.33 ± 18 mIU/L, p = 0.0015; glutathione vs PCOS; 13.59 ± 2.84 vs 39.33 ± 18 mIU/L, p = 0.0082, respectively) ().
Figure 2. Serum concentrations of IL-6, insulin, testosterone, hs-CRP, MDA and SHBG in study groups.
PCOS; Polycystic Ovary Syndrome, DHEA; Dehidroepiandrosteron, GSH; glutathione, IL-6; interleukin-6, hs-CRP; high-sensitive C-reactive protein, MDA; Malondialdehyde; SHBG; Sex hormone binding globulin
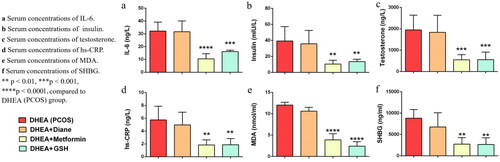
Table 1. Serum IL-6, insulin, testosterone, hs-CRP, MDA and SHBG levels in study groups.
Testosterone and SHBG levels were significantly lower in the metformin and glutathione groups than in the PCOS group (metformin vs PCOS for testosterone; 551.9 ± 236.8 vs. 1949 ± 689.6 ng/L, p = 0.0009 and glutathione vs PCOS for testosterone; 555.1 ± 352.5 vs. 1949 ± 689.6 ng/L, p = 0.0007, metformin vs PCOS for SHBG; 2736 ± 1538 vs 8824 ± 2023 ng/L, p = 0.0052 and glutathione vs PCOS for SHBG; 2664 ± 1565 vs 8824 ± 2023 ng/L, p = 0.0048, respectively). hs-CRP levels were also lower in the metformin and glutathione groups than in the PCOS group (1.83 ± 0.79 vs. 5.74 ± 2.13 ng/L, p = 0.0021 and 1.86 ± 0.97 vs. 5.74 ± 2.13 ng/L, p = 0.0023, respectively). Lastly, MDA levels were significantly lower in the metformin and glutathione groups than in the PCOS group (metformin vs PCOS; 3.88 ± 1.45 vs 12.03 ± 0.66 nmol/ml, p < 0.0001, glutathione vs PCOS; 2.41 ± 1.04 vs 12.03 ± 0.66 nmol/ml, p < 0.0001) (, ).
All serum parameters were higher in the Diane-35 group compared to the metformin and glutathione groups (metformin vs Diane-35 for IL-6; 10.5 ± 4.02 vs 31.69 ± 8.37 ng/L, p < 0.0001, glutathione vs Diane-35 for IL-6; 16.13 ± 1.13 vs 31.69 ± 8.37 ng/L, p = 0.0009), (metformin vs Diane-35 for insulin; 10.49 ± 4.71 vs 35.79 ± 16.72 mIU/L, p = 0.0025, glutathione vs Diane-35 for insulin; 13.59 ± 2.84 vs 35.79 ± 16.72 mIU/L, p = 0.0157), (metformin vs Diane-35 for testosterone; 551.9 ± 236.8 vs 1837 ± 798.6 ng/L, p = 0.001, glutathione vs Diane-35 for testosterone; 555.1 ± 352.5 vs 1837 ± 798.6 ng/L, p = 0.0007), (metformin vs Diane-35 for SHBG; 2736 ± 1538 vs 6768 ± 3321 ng/L, p = 0.054, glutathione vs Diane-35 for SHBG; 2664 ± 1565 vs 6768 ± 3321 ng/L, p = 0.049), (metformin vs Diane-35 for hs-CRP; 1.83 ± 0.79 vs 4.97 ± 1.98 ng/L, p = 0.008, glutathione vs Diane-35 for hs-CRP; 1.86 ± 0.97 vs 4.97 ± 1.98 ng/L, p = 0.009), (metformin vs Diane-35 for MDA; 3.88 ± 1.45 vs 10.62 ± 0.89 nmol/ml, p < 0.0001, glutathione vs Diane-35 for MDA; 2.41 ± 1.04 vs 10.62 ± 0.89 nmol/ml, p < 0.0001) (, ). No significant difference was observed between the Diane-35 and PCOS groups in terms of the analyzed serum markers. Likewise, there was no statistically significant difference between the metformin and glutathione groups for all parameters.
When we examined the groups in terms of primordial, primary, secondary, antral, atretic, and cystic follicle count, there was a statistically significant difference between all groups except for the number of primordial follicles. The primary, secondary, atretic, and cystic follicle counts were lower in the control group compared to the PCOS group (p < 0.05), while the antral follicle count was higher (p < 0.001). The antral follicle count was higher and atretic follicles were lower in the control group than in the Diane-35 group (p < 0.001, p < 0.05, respectively). All follicle counts were similar between the control and metformin groups, except for antral follicles. Antral follicles were more numerous in the control group than in the metformin group (p = 0.04). The number of all follicles was similar between control and glutathione groups (p < 0.05). When we compared all other groups with the PCOS group, the number of primary, secondary, atretic, and cystic follicles was significantly lower in the metformin and glutathione groups, while the number of primordial and antral follicles was significantly higher than the PCOS group (, ). Histological images are provided in .
Figure 3. Comparative follicle assessment in study groups.
PCOS; Polycystic Ovary Syndrome, DHEA; Dehidroepiandrosteron, GSH; glutathione, IL-6; interleukin-6, hs-CRP; high-sensitive C-reactive protein, MDA; Malondialdehyde; SHBG; Sex hormone binding globulin. a) Number of primordial, primary, secondary and antral follicles in study groups. *p < 0.05, **p < 0.01, ***p < 0.001, compared to DHEA (PCOS) group. +p < 0.05, ++p < 0.01, +++p < 0.001, compared to Control group. b) Number or atretic and cystic follicles. *p < 0.05, **p < 0.01, ***p < 0.001, ****p < 0.0001, compared to DHEA (PCOS) group. +p < 0.05, ++++p < 0.0001, compared to Control group.
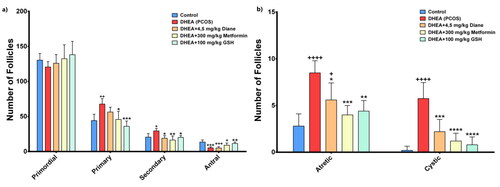
Figure 4. Photomicrographs of the representative ovary cross-sections in all experimental groups. Control group showed normal ovarian structures with several healthy follicles (arrow) at different developmental stages and corpus luteum (CL). DHEA (PCOS) group showed multiple cystic follicles (*) with a thin layer of flattened granulosa cells and a few healty follicles and CL. In the DHEA + 4,5 mg/kg Diane and DHEA + 300 mg/kg Metformin groups, number of cystsic follicles were decreased with the presence of growing healthy follicles. DHEA + 100 mg/kg GSH group showed a marked reduction in the of cystsic follicles with the presence of growing healthy follicles which were almost comparable with Control group. Healthy follicle (arrow), corpus luteum (CL), cystic follicle (*). Hematoxylin and eosin staining, Bar: 1000 µm in lower magnification and 500 µm in high magnification.
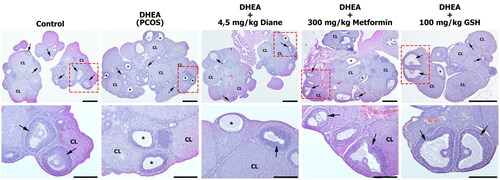
Table 2. Comparison of the primordial, primary, secondary, antral, atretic and cystic follicle counts in study groups.
Discussion
Combined oral contraceptive drugs, metformin, and antiandrogens are widely used in clinical practice for the pharmacological treatment of PCOS to improve menstrual regulation and glucose metabolism as well as reduce insulin resistance and serum androgen levels. In the current study, comparative analysis was performed to investigate the effects of glutathione on antioxidative and anti-inflammatory markers as well as insulin and androgen levels as a potential treatment strategy for PCOS when compared to the effect of metformin and Diane-35 in the DHEA-induced PCOS rat model. To the best of our knowledge, this is the first study to investigate the effects of glutathione, a powerful antioxidant, on PCOS by means of several markers in an experimental rat model. Promising results were observed, comparable to metformin and Diane-35 treatment.
IL-6 is a pleiotropic cytokine with a modulating role in ovarian development; it is involved in processes such as oocyte maturation, fertilization, and implantation [Citation21]. In previous studies, IL-6 has been suggested to be the key mediator of low-grade chronic inflammation and be increased in PCOS patients [Citation22,Citation23]. Metformin is reported as a relieving agent for chronic low-grade inflammation in women with PCOS based on its IL-6-lowering effect [Citation22]. On the other hand, in the meta-analysis study published by Wang et al. no significant changes in IL-6 levels were detected after metformin therapy in women with PCOS [Citation24]. In the current study, the decreased serum values of IL-6 in the glutathione group showed similar results to the metformin group, while the results of both groups were significantly better compared with the PCOS group. Mishra and Dingli showed that metformin decreased the expression of IL-6R via AMPK, mTOR, and miR34a [Citation25]. Although we did not analyze receptor activity, the IL-6-lowering effect of metformin could involve its receptor. Moreover, decreased levels of IL-6 have been reported after treatment with buthionine sulphoximine, a glutathione depletor, in rats [Citation26]. As suggested in this study, the glutathione-regulated NF-kB transcription factor, which is reported to be involved in the production of cytokines such as IL-1b, IL-6, and TNF-a, could be responsible for the IL-6 decrease [Citation26–28]. CRP is synthesized both in the liver, with the stimulation of IL-6 and TNF-α, and in adipose tissue. Several studies have reported the association between elevated CRP levels and PCOS [Citation12,Citation13,Citation29]. Most of the studies have indicated a decreased level of serum CRP in PCOS women after metformin therapy [Citation24,Citation30]. Accordingly, we observed a similar effect on hs-CRP levels in PCOS rats after metformin and glutathione treatment. The effect of glutathione supplementation on inflammatory markers has not been investigated well in the literature. However, glutamine, the amino acid source of glutathione, was shown to decrease the serum levels of CRP and IL-6, IL-18, and TNF-α in a PCOS rat model [Citation31]. Likewise, Raygan et al. reported that selenium intake, a cofactor for glutathione production, decreased hs-CRP and increased serum glutathione levels in patients with congestive heart failure [Citation32]. Similarly, Razavi et al. found that, although glutathione levels were not affected by selenium supplementation, selenium decreased hs-CRP and MDA levels in PCOS patients [Citation33]. Moreover, in Askari et al.’s study, hs-CRP and IL-6 levels were reduced following oral supplementation of N-acetylcysteine, one of the sources of cysteine, an amino acid that is involved in glutathione production [Citation34]. Khodaeifar et al. suggested that when they administered A. graveolens and C. zeylanicum extracts to rats, both combined and separately, MDA levels in the ovarian tissue decreased significantly [Citation35].
The underlying mechanism could be the anti-inflammatory and antioxidant role of metformin, which leads to a decrease in ROS production via stimulating AMPK-dependent pathways in macrophages, as indicated by Nassif et al. [Citation36]. As an indicator of lipid peroxidation, MDA levels were analyzed to show the antioxidant role of the treatment groups in our study. MDA levels were significantly lower in the metformin and glutathione groups compared to the PCOS group in our study. Contrary to our findings, Rencber et al. could not find any difference after metformin treatment in PCOS rats. Metformin treatment had failed to reduce plasma AMH levels, whereas resveratrol and combination of resveratrol with metformin had reduced the AMH levels in statistical significance [Citation19]. However, there are studies reporting that glutathione levels are lower in PCOS patients, as are other antioxidant levels. In Sulaiman et al.’s case control research, including 51 PCOS and 45 healthy women, the PCOS group had decreased glutathione levels and total antioxidant capacity. Due to elevated androgen production, ROS formation increases in PCOS patients, which may cause glutathione depletion [Citation37]. In the current study, IL-6, hs-CRP and MDA were lower in the glutathione group than in the PCOS group, as in the metformin group. Glutathione treatment may have a positive effect in terms of relieving low-grade inflammation and improving fertility in PCOS patients via its antioxidative feature. Research on ovarian tissue damage resulting from oxidative stress includes studies on ischemia-reperfusion injury using rat models. Substances such as chrysin, minocycline, and Olea europaea are being investigated for their antioxidant effects [Citation38–40].
PCOS is strongly associated with higher insulin levels, insulin resistance, and hyperglycemia [Citation8]. Hyperinsulinemia stimulates theca cells to produce testosterone, which is responsible for hirsutism in PCOS patients, and increased testosterone has an inhibitory effect on SHBG synthesis [Citation4]. Unbound steroid hormones consequently modulate the activity of the critical regulatory enzyme of androgen biosynthesis, P450c17, within the ovarian theca interna and interstitial cells [Citation41]. Previous studies have shown that metformin decreased insulin levels in PCOS patients [Citation42]. Similarly, in our study, insulin levels were lower in the metformin and glutathione groups than in the PCOS group. Due to the decrease in insulin levels, testosterone levels were also significantly lower in the metformin group in the current study. Rencber et al. reported a similar result with metformin treatment in PCOS rats [Citation19]. These findings support the anti-steroidogenic effects of metformin reported in previous studies [Citation43,Citation44]. The effect of glutathione supplementation on insulin levels have not been studied previously. In a randomized-controlled study, Søndergård et al. investigated the effects of 3-week oral supplementation of glutathione, resulting in increased insulin sensitivity in obese men with and without T2DM [Citation45]. Raygan et al. found that the intake of selenium, a cofactor of glutathione synthesis, decreased serum insulin levels in patients with congestive heart failure [Citation32].
Hyperinsulinemia leads to increased, testosterone levels, and high levels of testosterone regulate SHBG synthesis via a negative feedback mechanism in the liver. In Xing’s meta-analysis study, metformin had a relieving effect for hyperandrogenemia in PCOS patients via elevating SHBG levels [Citation46]. Similarly, it has been reported that metformin upregulates the level of SHBG via the PI3K/AKT pathway in PCOS rats with insulin resistance [Citation47]. On the other hand, Al-Noza et al. reported lower levels of testosterone and SHBG after metformin therapy in PCOS patients [Citation48]. Similar to these findings, we observed lower testosterone and SHBG levels after metformin and glutathione treatment in the current study. Although metformin may decrease insulin and total testosterone, free testosterone levels might still be high enough to inhibit SHBG synthesis. Free testosterone was not evaluated in the current study, so the decrease in SHBG levels could be related to low total testosterone levels, because most of the circulating testosterone was bound to SHBG in the body [Citation49].
Moreover, primary, atretic, and cystic follicle counts were significantly lower in the metformin and glutathione groups, while the number of antral follicles was significantly higher than that in the PCOS group in our study. Rencber et al. reported similar results in a PCOS rat model with metformin and/or resveratrol treatment, suggesting that metformin and glutathione could maintain folliculogenesis with their antioxidative effects [Citation19]. Although not consistent with previous studies, we report that Diane-35 does not cause significant changes in any of the serum parameters in PCOS patients. In a meta-analysis study by de Medeiros et al. nine out of the 11 studies reported that 35 µg ethynyl estradiol (EE) and 2 mg cyproterone acetate (CPA) (Diane-35) decreased CRP [Citation50]. According to the same study, oral contraceptives have no significant effect on IL-6. Feng et al. reported that Diane-35 decreased serum testosterone levels in PCOS patients [Citation14]. This contradiction may be due to the low population size of our study, as it was an animal study or liver-first pass effect of oral administration.
Our study has several limitations. The first is that it was an experimental animal study with a relatively small sample size, but this is a general limitation common in animal studies. The second is that experimental studies ought to be designed to determine the optimum dosage, schedule, and duration of glutathione treatment. Moreover, data obtained using an experimental animal model may not predict accurate results in humans directly, and future studies are needed to investigate the effect of glutathione on women with PCOS.
Conclusion
In conclusion, our study is the first to examine the comparative effects of glutathione with metformin and Diane-35 on oxidative and inflammatory markers in PCOS. Glutathione plays anti-inflammatory and antioxidant roles in the same way as metformin by lowering serum IL-6, insulin, testosterone, CRP, and MDA levels as well as atretic/cystic follicle count; it also improves antral follicle count and folliculogenesis in PCOS patients. It is anticipated that oxidative stress and antioxidants will continue to garner the interest of numerous researchers for years to come. A variety of additional substances are likely to be proposed, with their respective benefits being meticulously documented and analyzed. We believe that our findings will shed light on future clinical studies on PCOS therapy, including detailed analyses of the dose and method of use.
Disclosure statement
No potential conflict of interest was reported by the author(s).
Data availability statement
The data that support the findings of this study are available from the corresponding author, Pınar Özcan, upon reasonable request.
Correction Statement
This article has been corrected with minor changes. These changes do not impact the academic content of the article.
Additional information
Funding
References
- Azziz R, Carmina E, Chen Z, et al. Polycystic ovary syndrome. Nat Rev Dis Primers. 2016;2:1. doi:10.1038/NRDP.2016.57.
- Fauser BCJM. Revised 2003 consensus on diagnostic criteria and long-term health risks related to polycystic ovary syndrome. Fertil Steril. 2004;81:19–8. doi:10.1016/j.fertnstert.2003.10.004.
- Dludla PV, Mazibuko-Mbeje SE, Nyambuya TM, et al. The beneficial effects of N-acetyl cysteine (NAC) against obesity associated complications: a systematic review of pre-clinical studies. Pharmacol Res. 2019;146:104332. doi:10.1016/J.PHRS.2019.104332.
- Howkins J, Bourne G. Chapter 32 disorders of the ovary. In: Padubidri VG, Daftary SN, editors. Shaw’s textb gynecol. 16th ed. New Delhi: elsevier India Private Limited; 2014. p. 429–434.
- Vink JM, Sadrzadeh S, Lambalk CB, et al. Heritability of polycystic ovary syndrome in a dutch twin-family study. J Clin Endocrinol Metab. 2006;91(6):2100–2104. doi:10.1210/jc.2005-1494.
- Azziz R, Marin C, Hoq L, et al. Health care-related economic burden of the polycystic ovary syndrome during the reproductive life span. J Clin Endocrinol Metab. 2005;90(8):4650–4658. doi:10.1210/JC.2005-0628.
- Sabuncu T, Vural H, Harma M, et al. Oxidative stress in polycystic ovary syndrome and its contribution to the risk of cardiovascular disease. Clin Biochem. 2001;34(5):407–413. doi:10.1016/S0009-9120(01)00245-4.
- Verit FF, Erel O. Oxidative stress in nonobese women with polycystic ovary syndrome: correlations with endocrine and screening parameters. Gynecol Obstet Invest. 2008;65(4):233–239. doi:10.1159/000113046.
- Qu X, Donnelly R. Sex hormone-Binding globulin (SHBG) as an early biomarker and therapeutic target in polycystic ovary syndrome. Int J Mol Sci. 2020;21(21):1–17. doi:10.3390/IJMS21218191.
- Nur Torun A, Vural M, Cece H, et al. Paraoxonase-1 is not affected in polycystic ovary syndrome without metabolic syndrome and insulin resistance, but oxidative stress is altered. Gynecol Endocrinol. 2011;27(12):988–992. doi:10.3109/09513590.2011.569798.
- Soltani M, Moghimian M, Abtahi-Evari SH, et al. The effects of clove oil on The biochemical and HistologicalParameters, and autophagy markers in polycystic OvarySyndrome-model rats. Int J Fertil Steril. 2023;17(3):187–194. doi:10.22074/IJFS.2022.543640.1260.
- Kelly CCJ, Lyall H, Petrie JR, et al. Low grade chronic inflammation in women with polycystic ovarian syndrome. J Clin Endocrinol Metab. 2001;86(6):2453–2455. doi:10.1210/JCEM.86.6.7580.
- Lu J, Wang Z, Cao J, et al. A novel and compact review on the role of oxidative stress in female reproduction. Reprod Biol Endocrinol. 2018;16(1):80. doi:10.1186/S12958-018-0391-5.
- Feng W, Jia YY, Zhang DY, et al. Management of polycystic ovarian syndrome with diane-35 or diane-35 plus metformin. Gynecol Endocrinol. 2016;32(2):147–150. doi:10.3109/09513590.2015.1101441.
- Kuşçu NK, Var A. Oxidative stress but not endothelial dysfunction exists in non-obese, young group of patients with polycystic ovary syndrome. Acta Obstet Gynecol Scand. 2009;88(5):612–617. doi:10.1080/00016340902859315.
- Hammond CL, Lee TK, Ballatori N. Novel roles for glutathione in gene expression, cell death, and membrane transport of organic solutes. J Hepatol. 2001;34(6):946–954. doi:10.1016/S0168-8278(01)00037-X.
- Osuka S, Nakanishi N, Murase T, et al. Animal models of polycystic ovary syndrome: a review of hormone-induced rodent models focused on hypothalamus-pituitary-ovary axis and neuropeptides. Reprod Med Biol. 2019;18(2):151–160. doi:10.1002/RMB2.12262.
- Motta AB. Dehydroepiandrosterone to induce murine models for the study of polycystic ovary syndrome. J Steroid Biochem Mol Biol. 2010;119(3-5):105–111. doi:10.1016/J.JSBMB.2010.02.015.
- Furat Rencber S, Kurnaz Ozbek S, Eraldemlr C, et al. Effect of resveratrol and metformin on ovarian reserve and ultrastructure in PCOS: an experimental study. J Ovarian Res. 2018;11(1):55. doi:10.1186/S13048-018-0427-7.
- Pedersen T, Peters H. Proposal for a classification of oocytes and follicles in the mouse ovary. J Reprod Fertil. 1968;17(3):555–557. doi:10.1530/JRF.0.0170555.
- Tumu VR, Govatati S, Guruvaiah P, et al. An interleukin-6 gene promoter polymorphism is associated with polycystic ovary syndrome in South ındian women. J Assist Reprod Genet. 2013;30(12):1541–1546. doi:10.1007/S10815-013-0111-1.
- Xu X, Du C, Zheng Q, et al. Effect of metformin on serum interleukin-6 levels in polycystic ovary syndrome: a systematic review. BMC Womens Health. 2014;14:93. doi:10.1186/1472-6874-14-93.
- Lin YS, Tsai SJ, Lin MW, et al. Interleukin-6 as an early chronic inflammatory marker in polycystic ovary syndrome with insulin receptor substrate-2 polymorphism. Am J Reprod Immunol. 2011;66(6):527–533. doi:10.1111/J.1600-0897.2011.01059.X.
- Wang J, Zhu L, Hu K, et al. Effects of metformin treatment on serum levels of C-reactive protein and interleukin-6 in women with polycystic ovary syndrome: a meta-analysis: a PRISMA-compliant article. Medicine. 2017;96(39):e8183. doi:10.1097/MD.0000000000008183.
- Mishra AK, Dingli D. Metformin inhibits IL-6 signaling by decreasing IL-6R expression on multiple myeloma cells. Leukemia. 2019;33(11):2695–2709. doi:10.1038/S41375-019-0470-4.
- Wrotek S, Domagalski K, Jędrzejewski T, et al. Buthionine sulfoximine, a glutathione depletor, attenuates endotoxic fever and reduces IL-1β and IL-6 level in rats. Cytokine. 2017;90:31–37. doi:10.1016/J.CYTO.2016.10.005.
- Kil IS, Kim SY, Park JW. Glutathionylation regulates IkappaB. Biochem Biophys Res Commun. 2008;373(1):169–173. doi:10.1016/J.BBRC.2008.06.007.
- Baeuerle PA, Henkel T. Function and activation of NF-kappa B in the immune system. Annu Rev Immunol. 1994;12(1):141–179. doi:10.1146/ANNUREV.IY.12.040194.001041.
- Rudnicka E, Suchta K, Grymowicz M, et al. Chronic low grade ınflammation in pathogenesis of PCOS. Int J Mol Sci. 2021;22(7):789. doi:10.3390/IJMS22073789.
- Duan X, Zhou M, Zhou G, et al. Effect of metformin on adiponectin in PCOS: a meta-analysis and a systematic review. Eur J Obstet Gynecol Reprod Biol. 2021;267:61–67. doi:10.1016/J.EJOGRB.2021.10.022.
- Wu G, Hu X, Ding J, et al. The effect of glutamine on dehydroepiandrosterone-induced polycystic ovary syndrome rats. J Ovarian Res. 2020;13(1):57. doi:10.1186/S13048-020-00650-7.
- Raygan F, Behnejad M, Ostadmohammadi V, et al. Selenium supplementation lowers insulin resistance and markers of cardio-metabolic risk in patients with congestive heart failure: a randomised, double-blind, placebo-controlled trial. Br J Nutr. 2018;120(1):33–40. doi:10.1017/S0007114518001253.
- Razavi M, Jamilian M, Kashan ZF, et al. Selenium supplementation and the effects on reproductive outcomes, biomarkers of ınflammation, and oxidative stress in women with polycystic ovary syndrome. Horm Metab Res. 2016;48(3):185–190. doi:10.1055/S-0035-1559604.
- Askari M, Faryabi R, Mozaffari H, et al. The effects of N-Acetylcysteine on serum level of inflammatory biomarkers in adults. Findings from a systematic review and meta-analysis of randomized clinical trials. Cytokine. 2020;135:155239. doi:10.1016/J.CYTO.2020.155239.
- Khodaeifar F, Mohammad Bagher Fazljou S, Khaki A, et al. Investigating the role of hydroalcoholic extract of apium graveolens and cinnamon zeylanicum on metabolically change and ovarian oxidative ınjury in a rat model of polycystic ovary syndrome. Aras part med. International J Women’s Health Reproduct Sci. 2018;7(1):92–98. doi:10.15296/ijwhr.2019.15.
- Nassif RM, Chalhoub E, Chedid P, et al. Metformin ınhibits ROS production by human M2 macrophages via the activation of AMPK. Biomedicines. 2022;10(2):319. doi:10.3390/BIOMEDICINES10020319.
- Sulaiman MAH, Al-Farsi YM, Al-Khaduri MM, et al. Polycystic ovarian syndrome is linked to increased oxidative stress in omani women. Int J Womens Health. 2018;10:763–771. doi:10.2147/IJWH.S166461.
- Mohammadi Z, Hosseinianvari S, Ghazalian N, et al. The ımpact of chrysin on The folliculogenesis and ovarian apoptosis in Ischemia-Reperfusion ınjury in The rat model. Int J Fertil Steril [Internet]. 2022;16:299–305. doi:10.22074/IJFS.2021.540364.1200.
- Roshanaee MK, Abtahi-Eivary SH, Shokoohi M, et al. Protective effect of minocycline on bax and bcl-2 gene expression, histological damages and oxidative stress ınduced by ovarian torsion in adult rats. Int J Fertil Steril. 2022;16(1):30–35. doi:10.22074/IJFS.2021.522550.1069.
- Shokoohi M, Soltani M, Abtahi-Eivary SH, et al. Effect of hydro-alcoholic extract of Olea europaea on apoptosis-related genes and oxidative stress in a rat model of torsion/detorsion-induced ovarian damage. Asian Pac J Reprod. 2019;8(4):148–156. doi:10.4103/2305-0500.262831.
- Abdallah ABE, El-Ghannam MA, Hasan AA, et al. Selenium nanoparticles modulate Steroidogenesis-Related genes and ımprove ovarian functions via regulating androgen receptors expression in polycystic ovary syndrome rat model. Biol Trace Elem Res. 2023;201(12):5721–5733. doi:10.1007/S12011-023-03616-0.
- Sharma N, Lugani Y, Kaur A, Ahuja VK. Effect of metformin on insulin levels, blood sugar, and body mass index in polycystic ovarian syndrome cases. J Family Med Prim Care, 2019;8:2691, 2695. doi:10.4103/JFMPC.JFMPC_490_19.
- Attia GR, Rainey WE, Carr BR. Metformin directly inhibits androgen production in human thecal cells. Fertil Steril. 2001;76(3):517–524. doi:10.1016/S0015-0282(01)01975-6.
- Mansfield R, Galea R, Brincat M, et al. Metformin has direct effects on human ovarian steroidogenesis. Fertil Steril. 2003;79(4):956–962. doi:10.1016/S0015-0282(02)04925-7.
- Søndergård SD, Cintin I, Kuhlman AB, et al. The effects of 3 weeks of oral glutathione supplementation on whole body insulin sensitivity in obese males with and without type 2 diabetes: a randomized trial. Appl Physiol Nutr Metab. 2021;46(9):1133–1142. doi:10.1139/APNM-2020-1099.
- Xing C, Li C, He B. Insulin sensitizers for ımproving the endocrine and metabolic profile in overweight women With PCOS. J Clin Endocrinol Metab. 2020;105(9):2950–2963. doi:10.1210/CLINEM/DGAA337.
- Xing C, Lv B, Zhao H, et al. Metformin and exenatide upregulate hepatocyte nuclear factor-4α, sex hormone binding globulin levels and improve hepatic triglyceride deposition in polycystic ovary syndrome with insulin resistance rats. J Steroid Biochem Mol Biol. 2021;214:105992. doi:10.1016/J.JSBMB.2021.105992.
- Al-Nozha O, Habib F, Mojaddidi M, et al. Body weight reduction and metformin: roles in polycystic ovary syndrome. Pathophysiology. 2013;20(2):131–137. doi:10.1016/J.PATHOPHYS.2013.03.002.
- Keevil BG, Adaway J. Assessment of free testosterone concentration. J Steroid Biochem Mol Biol. 2019;190:207–211. doi:10.1016/J.JSBMB.2019.04.008.
- De Medeiros SF, Medeiros MD, Santos NDS, et al. Combined oral contraceptive effects on low-grade chronic ınflammatory mediators in women with polycystic ovary syndrome: a systematic review and meta-analysis. Int J Inflam. 2018;2018:9591509. doi:10.1155/2018/9591509.