Abstract
Polycystic ovary syndrome (PCOS) is a prevalent endocrine disorder among women of reproductive age and is associated with a variety of multi-system complications. The prevailing treatment strategy for PCOS is to individualize the interventions based on individual symptoms and patient complaints. However, optimal efficacy in treatment necessitates a focus on addressing the underlying pathogenic mechanisms. Uric acid (UA), the end product of purine metabolism, has been suggested to be involved in the development of several diseases, including PCOS. However, the precise mechanisms by which UA may affect PCOS remain incompletely understood. This literature review aims to investigate the correlation between UA and the various clinical presentations of PCOS, such as hyperandrogenism, insulin resistance (IR), ovulation disorders, obesity, and other related manifestations, through the analysis of epidemiological and clinical studies. The purpose of this study is to improve our comprehension of how UA contributes to each aspect of PCOS and their interrelationship, thus identifying the potential role of UA as a facilitator of PCOS. Furthermore, we explore potential pathways linking UA and PCOS, and propose therapeutic interventions based on these findings to optimize the management of this condition.
Background
Polycystic ovary syndrome (PCOS) is the most common endocrine disorder in reproductive-aged women [Citation1]. It is characterized by menstrual dysfunction, infertility, hyperandrogenism, hirsutism, acne, hyperinsulinemia, obesity, etc. Previous studies have shown that women with PCOS are prone to a certain degree of hyperuricemia [Citation2–4] and other metabolic disorders, including insulin resistance (IR) [Citation5], dyslipidemia [Citation6].
Hyperuricemia is defined as an abnormal increase in serum uric acid (SUA) levels. Uric acid (UA) is the end product of purine degradation in humans [Citation7]. The regulation of SUA levels is contingent upon the equilibrium between endogenous urate synthesis, primarily, and exogenous urate intake, as well as urate excretion [Citation8]. Disruptions in this equilibrium, such as those brought about by a diet high in purines [Citation9], excessive consumption of fructose [Citation10,Citation11] or alcohol [Citation12], or the presence of Chronic Kidney Disease (CKD) [Citation13], makes SUA levels increase accordingly. Besides, genetic factors can also contribute to SUA levels. And one study suggests that genetic variants have a greater effect on the onset and progress of hyperuricemia than diet [Citation14]. When SUA reaches 7.0 mg/dL (416 μmol/L) in men or 6.0 mg/dL (357 μmol/L) in women [Citation15], asymptomatic hyperuricemia can be diagnosed and urate crystals may gradually deposit in joints and/or other locations, leading to significant health complications. An illustrative instance is that, hyperuricemia exhibits a strong correlation with hypertension and other comorbidities [Citation16], thereby posing a considerable challenge for patients with PCOS.
Due to a lack of clear etiological understanding of PCOS, the current therapeutic approach is focused on managing its symptoms rather than addressing the root cause. Hence, there is a solid demand for further research to illustrate the exact cause of PCOS. As with how IR complemented our understanding of the pathogenesis of PCOS [Citation17], investigating the potential correlation between hyperuricemia and PCOS could also provide a different perspective. There is a notable correlation between elevated levels of SUA and the escalation in prevalence of both IR [Citation18] and metabolic syndrome [Citation11,Citation19,Citation20]. Considering that IR and metabolic syndrome have been identified as major underlying etiopathogenetic factors [Citation21], there may exist a potential association between hyperuricemia and the prevalence of PCOS. Further investigation into this potential correlation could provide valuable insights for improving comprehension and management of this condition. In this review, we aim to enhance comprehension of the mechanisms underlying the contribution of UA to various aspects of PCOS. Additionally, we explore potential pathways linking UA and PCOS, and propose therapeutic interventions based on these insights.
Uric acid metabolism
UA in vivo is synthesized mainly in liver and small intestine. Other tissues/organs like muscles, kidneys and vascular endothelium also contribute to UA production. The sources of it are exogenous purine from daily intake and endogenous purines from live and apoptotic cells [Citation22], in which xanthine oxidoreductase (XOR) plays a crucial role. XOR, one of the rate-limiting enzyme in purine metabolism, does not only catalyze the oxidative hydroxylation of hypoxanthine to xanthine, but also of xanthine to UA [Citation23]. The de novo biosynthesis of purines commences with the generation of 5′-phosphoribosyl 1-pyrophosphate (PRPP) from ribose 5′-phosphate and adenosine triphosphate (ATP) via the catalytic action of PRPP synthetase. Precedingly, the catabolism of ATP results in the formation of ADP and AMP, which are then metabolized into urate. Additionally, purines can be recycled through a salvage pathway that entails the enzyme hypoxanthine-guanine phosphoribosyl transferase [Citation24]. Given that uricase gene was inactivated in humans, uric acid became the end product of purine metabolism [Citation25].
The most important regulator of UA excretion is the kidney, for it excretes approximately 70% of daily UA production through transporters like organic anion transporter 4 (OAT4), glucose transporter 9 (GLUT9) and ABCG2 [Citation22]. And the reabsorption of UA in renal tubules via urate transporter 1 (URAT1), the urate-anion exchanger encoded by SLC22A12 gene, also contributes to UA homeostasis in vivo [Citation26]. It is worth noting that gastrointestinal tract does not only synthesize UA in vivo but also shoulders about 30% of UA excretion [Citation27,Citation28] (). The alteration of SUA levels has far-reaching effects on physiological homeostasis through a multitude of pathways, and elevated SUA levels/hyperuricemia have been implicated in the development or exacerbation of various comorbid conditions. Therefore, we make the assumption that hyperuricemia may associate with PCOS through several mechanisms.
Serum uric acid level and its impacts on the pathogenesis and progress of PCOS
Uric acid and BMI/prevalence of obesity
PCOS has always been thought to have a strong connection with obesity, mainly due to IR [Citation29]. It is similarly acknowledged that hyperuricemia possesses a significant association with obesity as well [Citation30,Citation31]. In a retrospective study undertaken by Zhang et al. it was observed that PCOS patients with hyperuricemia demonstrated elevated body weight and body mass index (BMI) than the non-hyperuricemia group. Moreover, the observed patterns of escalation in Visceral Adipose Tissue (VAT) mass, VAT volume, and VAT area displayed statistically significant associations with SUA levels [Citation32].
A previous study also indicates that there might exist a notable positive relationship between SUA levels and the prevalence of obesity among Chinese population [Citation33]. And a 10-year follow-up CARDIA study has proved that not only the prevalence of obesity, but BMI itself also has a significant association with SUA [Citation34–38]. For example, the fractional excretion of UA is relatively lower in individuals with obesity when compared to those with a normal BMI. However, it is plausible that weight reduction interventions could restore the fractional excretion of UA to a normal range in the obese populations [Citation39]. In addition, elevated levels of XOR activity have been observed in patients presenting with both obesity and dyslipidemia [Citation40], which further strengthen this hypothesized correlation.
On the other hand, a study on people with type 2 diabetes and WHO class II or higher obesity revealed that surgery-induced weight loss can bring about clinically relevant reductions in SUA levels [Citation41]. And in almost all study population not involved with gout, bariatric surgery resulted in a decrease in SUA levels to concentrations below saturation [Citation42–44]. Not only surgical interventions resulting in weight reduction, but also healthy dietary approaches aimed at inducing weight loss, can effectively decrease SUA levels [Citation45].
These studies demonstrate a correlation between body weight decreasing and SUA level reducing. Therefore, weight loss intervention has been widely recognized as a recommendation for the management of hyperuricemia and gout [Citation46–49]. It is also considered as a widely accepted therapeutic target for urate concentration (no greater than 360 mmol/L) in male individuals with elevated cardiovascular risk [Citation50]. In addition to that, it was observed that administration of allopurinol in fructose-fed rats was able to prevent weight gain. Importantly, this effect was not found to be attributed to any potential influence of allopurinol on dietary intake [Citation11]. Based on these observations, we assume that some certain effect on the management of hyperuricemia will contribute to the amelioration of PCOS including weight loss.
Thus far, the precise underlying mechanism through which weight loss contributes to reduced SUA levels remains poorly understood. However, this mechanism is believed to be influenced by multiple factors, including improved IR [Citation51] and reduced levels of reactive oxygen species (ROS) [Citation45]. This proposition is formulated based on the premise that the pathogenesis of obesity-associated hyperuricemia is influenced by the impact of IR, lipid peroxidation and ROS production. As PCOS is also frequently comorbid with obesity, dyslipidemia and other related factors, the association between hyperuricemia and PCOS has become increasingly evident.
Uric acid and reproductive hormones
Epidemiological investigations have demonstrated a positive correlation between age and the prevalence of hyperuricemia in various populations [Citation52,Citation53]. However, the distinct prevalence rates of hyperuricemia in elderly males and postmenopausal females suggest that reproductive hormones may exert a modulatory influence. Generally speaking, SUA level in males is higher than it in females [Citation54–56]. Furthermore, investigations revealed a noteworthy association between total testosterone levels and SUA levels among women with PCOS, thus implying a linkage between hyperandrogenism and hyperuricemia in this specific patient cohort [Citation4]. It is of noteworthy significance that the mitigation of androgen excess through the administration of antiandrogenic oral contraception leads to a substantial reduction in SUA levels [Citation30].
These observations and sex-based differences can also be attributed to the potential urate-lowering effect of female hormones, estrogen and progesterone in particular. The most compelling evidence supporting this claim is derived from an important study conducted by Mumford et al. that identified variations in UA levels throughout the menstrual cycle which also observed that the concentration of UA exhibited a peak during the follicular phase and experienced a subsequent decline during the luteal phase [Citation57]. In addition, this study also observed that SUA levels exhibited a positive correlation with follicle-stimulating hormone (FSH) levels, while displaying an inverse association with concentrations of estradiol and progesterone. Jung et al. have proposed that the observed reduction in UA levels is typically ascribed to the urate-lowering properties of progestogens, rather than estrogens [Citation58]. However, it is important to note that the potential influence of estrogens cannot be overlooked, given that previous research has shown a decrease in plasma UA concentration following the administration of conjugated and synthetic E2. In one such study investigating the effects of endogenous E2 on UA, this effect was observed [Citation59]. It is worth noting that the marked divergence in UA levels between males and females becomes less pronounced after women experience menopause [Citation60,Citation61]. But studies have shown that there is a decrease in the level of UA in postmenopausal women who have undergone hormone replacement therapy (HRT) [Citation62,Citation63]. These findings further corroborate the urate-lowering effect of female hormones.
A strong association also exists between testosterone levels and SUA levels, as supported by findings indicating that the administration of testosterone leads to a marked increase in plasma UA levels in postmenopausal women [Citation59]. Similarly, there is a significant reduction in SUA levels observed in individuals who have undergone castration surgery [Citation64]. This finding is congruent with previous research, which found that administration of an antiandrogenic oral contraceptive led to a significant reduction in SUA levels [Citation30]. It is hypothesized that androgens may raise SUA levels by inducing hepatic metabolism of purine nucleotides [Citation65]. Therefore, upon inhibition of androgenic activity, there is a subsequent decrease in SUA levels. In addition, testosterone has the potential to induce functional modifications in the renal UA reabsorption system via the upregulation of various transporters, such as URAT1, sodium-coupled monocarboxylate transporter 1 and GLUT9 [Citation66]. Given that hyperandrogenism is a central feature of PCOS and is implicated in various pathophysiological processes, it is plausible that its capacity to increase UA levels could serve as a connecting bridge between PCOS and hyperuricemia.
The notion has been postulated that E2 may potentially modulate the serum concentrations of UA via mechanisms that involve renal clearance, secretion, and reabsorption [Citation67]. The aforementioned hypothesis aligns with the findings of a prospective study carried out on transgender individuals. The study demonstrated that estrogen’s purported capacity to decrease urate levels may be linked to the upregulation of fractional excretion of uric acid [Citation68]. In addition, it has been observed that estrogen exerts renal-protective effects through the promotion of nitric oxide (NO) production.
ABCG2, a prominent transporter protein, facilitates the extrusion of UA from the cellular environment [Citation69]. This transporter is expressed in kidney, liver, and small intestine, as well as in the blood-brain and blood-placental barriers, and mammary epithelial cells. In the renal proximal tubule, ABCG2 expression is particularly notable and serves a pivotal function in UA excretion [Citation70]. The presence of estrogen receptor (ER) binding sites within the promoter region of ABCG2 has been documented, indicating that the transcriptional activity of ABCG2 can potentially be modulated by estrogen [Citation71]. However, the effect estrogen exhibits is down-regulating the ABCG2 protein expression [Citation72], which would decrease UA secretion in renal proximal tubule, leading to an elevation in SUA levels. This may seem incongruous with the generally lower SUA levels observed in females. However, this is in line with the hypothesis that the urate-lowering properties of progestogens, rather than estrogens, may be responsible for the observed reduction in SUA levels. Indeed, studies have shown that SUA levels experience a subsequent decline during the luteal phase, coinciding with the peak of progestin production [Citation57]. Therefore, the role of reproductive hormone in UA metabolism is complex, and further research is needed to clarify their relationship.
Uric acid and ovulation disorders and other reproductive disorders
Ovulation disorder is a central manifestation of PCOS [Citation73] and has been hypothesized to be linked with dysregulated oxidative metabolism. The heightened production of ROS exerts a deleterious impact on cellular functions and disrupts metabolic homeostasis by mechanisms inducing DNA damage, thus elevating UA synthesis [Citation74]. UA can be found in the follicular fluid of the female ovary, and a former study experimented on buffalo ovaries has proven the existence of association between increased levels of SUA and disruption of the redox homeostasis inside the follicle [Citation74]. This aberrant metabolic process is responsible for the elevated titer of UA in follicle fluid during oxidative stress [Citation75]. In light of these findings, it is plausible to consider UA level in follicle fluid as a potential marker for identifying follicular dysfunction or atresia. Thus, we assume that the pro-oxidative effect of UA may create a vicious cycle, further exacerbating the detrimental effects of oxidative stress on the follicle.
UA is a derivative of purine, and various studies have reported that purines have the potential to obstruct oocyte maturation [Citation76–78], and this observation could possibly explicate the correlation between UA and sporadic anovulation. The association suggests that reduced levels of estradiol, which is concomitant with elevated UA, could give rise to an upsurge in FSH levels that are imperative for commencing feedback mechanisms to counterbalance compromised follicular development. It has been reported that women who menstruate on a regular basis and have elevated levels of serum urate exhibit a markedly increased likelihood of experiencing anovulatory menstrual cycles [Citation56]. In comparison to non-obese women, obese women exhibit higher levels of UA in follicle fluid and ovarian follicles with increased oxidative stress and reduced antioxidant capacity among various metabolites [Citation79]. Given the shared characteristics of hormone imbalance and obesity among individuals with PCOS, UA may act as a potential predictor or promoter in the pathogenesis and progression of PCOS through mechanisms including oxidative stress. However, comprehensive investigations into this phenomenon remain scarce, and thus require further exploration.
Uric acid, glucose metabolism and insulin sensitivity
IR has been considered the principal etiological factor underlying the pathogenesis and progression of PCOS [Citation21,Citation29]. Empirical evidence has established that the use of insulin-sensitizing agents such as metformin has been efficacious in treating individuals diagnosed with PCOS who exhibit IR. However, the correlation between SUA levels and insulin resistance remains a matter of contention. Previous researches have indicated that elevated levels of SUA are commonly seen in individuals with prediabetic conditions, impaired glucose tolerance [Citation38,Citation80–84] or type 2 diabetes [Citation85]. Chou et al. conducted a community-based epidemiological study among an adult Chinese population without diabetes in Taiwan. The study revealed a significant positive and independent correlation between the SUA level and hyperinsulinemia in both genders, even after adjusting for potential confounding variables, such as hypertriglyceridemia, hypertension, obesity, and plasma glucose levels [Citation86]. Of note, the correlation between SUA level and hyperinsulinemia appears to be more pronounced among women than men. This observation provides further evidence that this association may contribute to the development of reproductive disorders in women. However, it has also been observed that some individuals diagnosed with diabetes generally exhibit lower SUA levels compared to those who are non-diabetic [Citation87,Citation88]. The results of these studies may suggest that there is a non-linear relationship between SUA levels and the development of glucose metabolic disorders, characterized by a bell-shaped curve. This biphasic relationship may be attributed to the uricosuric effect of glycosuria, which typically occurs when the blood glucose level exceeds 10 mmol/L (180 mg/dL) [Citation38]. In contrast, the study results also indicated a consistent rise in both SUA levels and the prevalence of hyperuricemia with increasing fasting C-peptide levels, in conjunction with a rise in serum insulin levels and IR. These findings necessitate further investigation to clarify the exact underlying mechanism.
The presence of an inverse relationship between heightened insulin levels and decreased renal excretion of urate has been revealed [Citation89–92]. This association may be attributed to the potential ability of insulin to promote the facilitation of renal urate reabsorption by means of activating URAT1 and the Na+-dependent anion co-transporter situated in the brush border membranes of the renal proximal tubule [Citation93]. As a consequence of the aforementioned mechanism, some scholars propose that hyperuricemia in patients with metabolic syndrome is a consequence of hyperinsulinemia secondary to IR [Citation89]. The current body of research has yet to elucidate the mechanisms through which insulin decreases UA reabsorption in the kidney. Furthermore, the question of whether insulin can stimulate UA production remains unanswered. In short, it is conceivable that the heightened insulin levels observed in patients with IR syndrome may contribute to their increased levels of SUA. However, this effect may be counteracted by the onset of diabetes, resulting in the occurrence of glycosuria and uricosuria, ultimately leading to decreased UA levels.
A prospective study was conducted to investigate the impact of either a low-energy diet or an insulin-sensitizing agent on IR in overweight hypertensive patients. The results indicated that the administration of intervention led to a significant improvement in IR, resulting in a decrease in SUA levels [Citation51]. And some suggest, once IR is improved, insulin-enhanced reabsorption of urate at the renal tubules will in turn reduce, thus lowering SUA levels in vivo.
Uric acid and lipid metabolism/dyslipidemia
In the study conducted by Zhang et al. mentioned previously [Citation32] , it was observed that PCOS patients with hyperuricemia exhibited more pronounced disturbances in lipid metabolism compared to their counterparts without hyperuricemia, which suggests a strong association between hyperuricemia and dyslipidemia in PCOS.
Hyperuricemia has been identified as a significant risk factor for the development of high LDL cholesterol and hypertriglyceridemia. The precise mechanistic underpinnings of the association between elevated UA levels and dyslipidemia have yet to be fully elucidated. Lanaspa et al. demonstrated the first causal link between UA and hepatic steatosis, mediated by enhanced lipogenic and triglyceride pathways. This effect is probably due to elevated generation of superoxide by the mitochondria. This metabolic perturbation ultimately triggers de novo lipogenesis, facilitating the synthesis of fatty acids within the liver [Citation94]. Additionally, the pathological accumulation of UA within the intracellular milieu can perturb physiological homeostasis and instigate adipose tissue deposition and gluconeogenesis. This effect is mediated by the activation of AMP deaminase and concomitant inhibition of AMP-activated kinase [Citation95]. AMPK plays a crucial role in fat metabolism by promoting fat oxidation through the inhibition of acetyl-CoA carboxylase 1 activity and the transportation of malonyl-CoA to the mitochondria [Citation96], as well as by activating peroxisome proliferator-activated receptor (PPAR)-α and its downstream target genes [Citation97].
High XOR [Citation98] and URAT1 [Citation99] activity in adipose tissue may also link dyslipidemia and hyperuricemia by enabling adipocytes and adipose tissue to generate and secrete UA. Based on this observation, it is plausible to suggest that dyslipidemia may contribute to the dysregulation of UA metabolism in adipose tissue. Oberbach et al. showed that high-fat diet-induced obese rats have increased XOR activity in subcutaneous but not visceral adipose tissue, suggesting a connection between adipose and UA metabolism in metabolic disorders like obesity [Citation100]. Further studies are warranted to investigate the potential mechanistic links between these two metabolic perturbations.
As noted previously, fructose has been implicated in the pathogenesis of metabolic syndrome, contributing to lipid accumulation and obesity. Nevertheless, the mechanisms underlying its lipogenic effects remain unclear. Indeed, despite the well-established association between fructose consumption and lipogenesis, a recent investigation found that only a small proportion (3%) of ingested fructose is converted to triglycerides [Citation101], suggesting that alternative pathways may be involved in the observed effects of fructose on lipid metabolism. Notably, fructose-enriched diets consistently raise SUA levels in short-term and long-term studies [Citation102]. The hepatic cell line, HepG2, when exposed to UA, exhibited an upregulation of the nicotinamide adenine dinucleotide phosphate (NADPH) oxidase isoform, NOX4, and subsequent enhanced translocation of the isoform to mitochondria. This event facilitated an increase in mitochondrial superoxide production, which in turn led to the reduction in activity of the enzyme aconitase. This decrease in aconitase activity led to the accumulation of citrate, which served as the substrate for de novo lipogenesis and ultimately stimulated lipogenesis [Citation94]. To corroborate this hypothesis, it has been demonstrated that pharmacological inhibition of UA biosynthesis with allopurinol effectively mitigates the lipogenic effects of fructose. Nakagawa et al. conducted a study that also demonstrated the efficacy of allopurinol in inhibiting and reversing the clinical features of metabolic syndrome in fructose-fed rats. Specifically, the study found that allopurinol treatment resulted in a reversal of hypertriglyceridemia and IR, among other observed benefits [Citation11]. In a similar vein, the therapeutic intervention of benzbromarone has been found to ameliorate the aberrant metabolic profile of fructose-fed rats, characterized by elevated levels of uric acid, triglycerides, and insulin [Citation103].
Furthermore, another study has demonstrated that allopurinol exhibits potential therapeutic benefits in mitigating the metabolic abnormalities associated with metabolic syndrome in rats induced by means other than fructose consumption. These findings suggest a potential broader role for UA and XOR in the pathophysiology of metabolic disorders beyond the context of high fructose intake [Citation104]. Of note, pharmacological intervention aimed at managing hypertriglyceridemia with fenofibrate or atorvastatin has been observed to decrease SUA levels as well [Citation105,Citation106].
In summary, UA may stimulate lipid production and vice versa. Therefore, UA dysregulation can trigger a positive feedback loop, causing dyslipidemia, obesity and other metabolic disorders.
Uric acid and oxidative stress
Oxidative stress is a condition characterized by a pathological state of excess pro-oxidants over antioxidants. This state has been implicated in the pathogenesis of various diseases, including PCOS [Citation107], hypertension [Citation108], metabolic syndrome [Citation109], and even certain malignant tumors [Citation110]. ROS generation is primarily attributed to enzymes such as NADPH oxidase, xanthine oxidase, mitochondrial enzymes, myeloperoxidase, lipoxygenase, and uncoupled NO synthase [Citation111].
Ames et al. firstly hypothesized in 1981 that plasma urate may serve as an antioxidant for its ability to scavenge singlet oxygen, hydroxyl radicals and oxo-heme oxidants [Citation112], and to protect proteins involved in antioxidant procedures from peroxynitrite [Citation113]. Despite its plausible antioxidative impact, heightened levels of SUA have been widely acknowledged as a harbinger of various metabolic disorders, including IR, dyslipidemia and obesity [Citation114–117] as well as cardiovascular disease (CVD) [Citation30,Citation118,Citation119]. Furthermore, it is postulated that the pathophysiological mechanisms underpinning these disorders involve oxidative stress, redox-dependent inflammation, and mitochondrial dysfunction, which are believed to constitute the critical links between these conditions [Citation109,Citation120,Citation121]. Under certain environmental conditions, the antioxidant properties of UA may be compromised. It has been documented that intracellular UA exerts solely pro-oxidative effects via its ability to activate NADPH oxidases and the subsequent generation of ROS [Citation122,Citation123], as well as through several other pathways, including but not limited to the reduction of antioxidant NO levels in endothelial cells, activation of peroxynitrite-mediated lipid oxidation, and stimulation of pro-inflammatory biomarkers [Citation119]. The involvement of NADPH oxidases in the production of ROS can result in the activation of pro-inflammatory signaling pathways mediated by mitogen-activated protein kinases [Citation119]. Furthermore, in the presence of lipid peroxides, the pro-oxidant effect of UA has been observed to be of notable magnitude [Citation124], thus involve itself in the pathogenesis of the metabolic syndrome.
Within the context of the IR syndrome, the malfunctioning of oxidative phosphorylation is speculated to elevate systemic adenosine concentrations by augmenting the intracellular levels of long-chain fatty acid coenzyme A esters [Citation125]. This elevation of adenosine levels, in turn, can lead to the retention of sodium, urate, and water in the renal system [Citation126–128]. Certain experts have postulated that prolonged elevation of extracellular adenosine levels could potentially facilitate the development of hyperuricemia by promoting the production of urate [Citation125].
Existing literature has reported a positive correlation between PCOS in women and elevated levels of oxidative stress [Citation2,Citation3]. The augmentation of XOR activity, as well as the presence of IR and dyslipidemia, are of considerable significance in the context of oxidative stress. Based on current evidence, it is posited that hyperuricemia-induced oxidative stress may be partly responsible for the pathogenesis of elevated levels of ROS in women with PCOS. Urate-lowering agents may help hyperuricemia and its complications by reducing oxidative stress and inflammation. However, more studies are needed to elucidate the mechanism linking hyperuricemia and PCOS before using these agents off-label.
Uric acid and long-term complications of PCOS
PCOS, in addition to commonly occurring endocrine disorders, has been linked with a variety of possible long-term consequences, including CKD and CVD [Citation129]. Furthermore, PCOS has been identified as a contributing factor in various complications related to pregnancy, including gestational diabetes, pregnancy-induced hypertension, and even pre-eclampsia [Citation130,Citation131].
Empirical evidence suggests that elevated SUA levels serve as a predictive indicator for the onset of obesity and hypertension [Citation132]. In light of the well-documented association between SUA levels and blood pressure, numerous studies have identified a possible correlation between heightened SUA levels and a reduced eGFR [Citation133,Citation134]. This relationship may be attributed to the effect of SUA on blood pressure regulation [Citation135], which is believed to be a consequence of enhanced vascular stiffness [Citation136,Citation137]. And it is postulated that oxidative stress stemming from hyperuricemia may contribute to the aging and apoptosis of endothel, ial cells. These mechanisms may, in turn, be implicated in the pathogenesis of hypertension and CKD [Citation138].
SUA levels increase in late pregnancy due to maternal clearance of fetal metabolites [Citation139]. Studies have indicated that nearly 50% of women who develop hypertensive disorders of pregnancy or gestational diabetes mellitus experience a marked rise in UA concentrations during pregnancy [Citation140,Citation141].
In summary, these findings not only emphasize the critical role of UA in these conditions but also suggest that optimal management of UA may have the potential to ameliorate and even prevent these complications.
Discussion
PCOS is a prevalent medical condition that primarily affects reproductive function. It has been established that PCOS is closely associated with various diseases such as diabetes, hypertension, CKD, and even endometrial cancer. Given the high morbidity rate of PCOS, it is of great importance to conduct extensive research on the underlying mechanisms and develop therapeutic strategies for optimal disease management. Each therapeutic approach is expected to target the underlying etiology of diseases and evolve in accordance with the constantly advancing knowledge of disease mechanisms. However, PCOS presents a significant challenge to clinicians due to the heterogeneous nature of the disease and the limited understanding of its pathogenesis. As a result, the current treatment approaches for PCOS are primarily focused on managing its symptoms, rather than targeting its causes.
The precise mechanisms of PCOS remain elusive, highlighting the need for further investigation. However, current research progress in this regard is less than satisfactory. In recent reports, an association between elevated SUA levels and PCOS has been established. SUA may play a crucial role in PCOS and its related metabolic disorders, as they share common risk factors. Elucidating the intricate relationship between hyperuricemia and PCOS may offer valuable insights into the pathogenesis mechanisms of PCOS. By filling one of the missing pieces of this puzzle, we may uncover novel therapeutic strategies to tackle this complex condition.
Previous literature has established robust associations between hyperandrogenism, obesity, IR, and ovulation disorders in PCOS. While some studies have examined the interplay between hyperuricemia and obesity, IR, and dyslipidemia as a collective, such an approach may cause bias given that BMI is an independent factor that has been linked to hyperuricemia even after controlling for IR and levels of total cholesterol and triglycerides [Citation35]. Therefore, this review seeks to explore the relationship between UA and the distinct clinical features of PCOS, including hyperandrogenism, menstrual irregularities, and metabolic dysfunction. By gathering current evidence, we aim to provide a comprehensive understanding of the complex mechanisms underlying the pathophysiology of PCOS, with a particular emphasis on the role of UA. This literature review provides evidence of a notable association between increased UA concentrations and various clinical manifestations and long-term complications in patients with PCOS. Specifically, elevated UA levels are correlated with conditions such as hyperandrogenism, IR, ovulation disorders, metabolic syndrome, and other related factors. These conditions are mutually interdependent and exacerbate one another, resulting in the formation of a self-perpetuating cycle. Hyperuricemia can trigger a cascade of CVD, CKD, metabolic disorders in women with PCOS, underscoring the importance of close monitoring of UA levels during PCOS treatment. Correcting metabolic abnormalities is especially important when hyperuricemia develops, as it affects the therapeutic outcomes of PCOS and the prevention of its related complications.
As research on PCOS progresses, it may be possible to illustrate the link between the metabolism of UA and PCOS. Such an association could potentially contribute to the diagnosis and treatment of PCOS. Thus, it is essential to offer appropriate care and tailored management to lower the risk and enhance the outcome of patients with both PCOS and hyperuricemia. In addition, a study by El‑Eshmawy et al. suggest that the combination of serum UA/Cr ratio and FAI demonstrates the potential for additive or synergistic effects in enhancing the diagnosis prediction of PCOS among obese women [Citation142]. Therefore, in the discourse of theoretical mechanisms, it is important to note that hyperuricemia may not solely instigate PCOS. Rather, it may potentially interact with other factors such as IR, hyperandrogenemia, and obesity, thereby intensifying the syndrome ().
Figure 2. The interactions between hyperuricemia and pathological manifestations of Polycystic Ovary Syndrome.
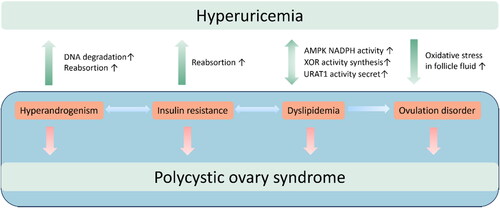
However, there is a lack of widely reported approaches to regulating and controlling UA metabolism to ameliorate its adverse effects on PCOS symptoms. Additionally, although there is substantial evidence to support the correlation between elevated levels of UA and the clinical manifestations of PCOS, the academic literature on the association between UA and ovulation disorders is relatively scarce. To note, the levels of UA in serum may not accurately represent the follicular fluid UA levels, as the latter may contain UA that has been secreted locally by granulosa cells. Thus, the potential efficacy of urate-lowering therapy in ameliorating the symptoms of PCOS remains equivocal. In order to substantiate this hypothesis, it is necessary to move from a theoretical framework to a practical one. One approach could be conducting animal experiments that are pertinent to the subject matter, to ascertain whether the implementation of urate-lowering interventions can ameliorate the clinical features of PCOS-like mice. If relating explorations yield satisfactory results, the investigation of the promising relationship between UA and PCOS will hold the potential to provide significant therapeutic benefits for individuals with hyperuricemia and associated aspects of PCOS. By gaining a better understanding of this potential correlation, healthcare providers may be able to develop more effective treatment strategies for patients who are affected by these conditions.
It is worth expecting that, a combination of basic experiments and clinical research will yield a better understanding of the specific mechanisms underlying the interaction between UA levels and PCOS, identifying the role of UA as a pivotal node within the complex interplay of pathophysiological mechanisms of PCOS and leading to the development of targeted therapies for PCOS patients, ultimately alleviating the burden of PCOS and its associated complications, including hyperuricemia, for affected individuals.
Authors contributions
Zhentao Gong wrote the original draft and prepared figures; Lingshan Zhang edited the original draft; Yingli Shi conceived the idea. All authors approved the final article. All authors have read and agreed to the published version of the manuscript.
Abbreviations | ||
PCOS | = | Polycystice ovary syndrome |
IR | = | Insulin resistance |
UA | = | Uric acid |
SUA | = | Serum uric acid |
CKD | = | Chronic kidney disease |
XOR | = | Xanthine oxidoreductase |
PRPP | = | 5’-phosphoribosyl 1-pyrophosphate |
ATP | = | Adenosine triphosphate |
ADP | = | Adenosine diphosphate |
AMP | = | Adenosine monophosphate |
OAT4 | = | Organic anion transporter 4 |
GLUT9 | = | Glucose transporter 9 |
URAT1 | = | Urate transporter 1 |
BMI | = | Body mass index |
ROS | = | Reactive oxygen species |
FSH | = | Follicle-stimulating hormone |
HRT | = | Hormone replacement therapy |
NO | = | Nitric oxide |
ER | = | Estrogen receptor |
eGFR | = | Estimated glomerular filtration rate |
NADPH | = | Nicotinamide adenine dinucleotide phosphate |
CVD | = | Cardiovascular disease |
Acknowledgement
We are grateful to Yan Liu, Ph.D. for the help with suggestions for writing.
Disclosure statement
The authors declare that the research was conducted in the absence of any commercial or financial relationships that could be construed as a potential conflict of interest.
Data availability statement
Not applicable.
Additional information
Funding
References
- Stephen F. Polycystic ovary syndrome. N. Engl. J. Med. 1995;333:1.
- Macut D, Simic T, Lissounov A, et al. Insulin resistance in non-obese women with polycystic ovary syndrome: relation to byproducts of oxidative stress. Exp Clin Endocrinol Diabetes. 2011;119(7):451–10. doi:10.1055/s-0031-1279740.
- Durmus U, Duran C, Ecirli S. Visceral adiposity index levels in overweight and/or obese, and non-obese patients with polycystic ovary syndrome and its relationship with metabolic and inflammatory parameters. J Endocrinol Invest. 2017;40(5):487–497. doi:10.1007/s40618-016-0582-x.
- Mu L, Pan J, Yang L, et al. Association between the prevalence of hyperuricemia and reproductive hormones in polycystic ovary syndrome. Reprod Biol Endocrinol. 2018;16(1):104. doi:10.1186/s12958-018-0419-x.
- Moran LJ, Misso ML, Wild RA, et al. Impaired glucose tolerance, type 2 diabetes and metabolic syndrome in polycystic ovary syndrome: a systematic review and Meta-Analysis. Hum Reprod Update. 2010;16(4):347–363. doi:10.1093/humupd/dmq001.
- Legro RS, Kunselman AR, Dunaif A. Prevalence and predictors of dyslipidemia in women with polycystic ovary syndrome. Am J Med. 2001;111(8):607–613. doi:10.1016/S0002-9343(01)00948-2.
- Johnson RJ, Lanaspa MA, Gaucher EA. Uric acid: a danger signal from the RNA world that may have a role in the epidemic of obesity, metabolic syndrome, and cardiorenal disease: evolutionary considerations. Semin Nephrol. 2011;31(5):394–399. doi:10.1016/j.semnephrol.2011.08.002.
- Jakše B, Jakše B, Pajek M, et al. Uric acid and Plant-Based nutrition. Nutrients. 2019;11(8):1736. doi:10.3390/nu11081736.
- Choi HK, Atkinson K, Karlson EW, et al. Purine-Rich foods, dairy and protein intake, and the risk of gout in men. N Engl J Med. 2004;350(11):1093–1103. doi:10.1056/NEJMoa035700.
- Khitan Z, Kim DH. Fructose: a key factor in the development of metabolic syndrome and hypertension. J Nutr Metab. 2013;2013:682673–682612. doi:10.1155/2013/682673.
- Nakagawa T, Hu H, Zharikov S, et al. A causal role for uric acid in fructose-induced metabolic syndrome. Am J Physiol Renal Physiol. 2006;290(3):F625–F631. doi:10.1152/ajprenal.00140.2005.
- Neogi T, Chen C, Niu J, et al. Alcohol quantity and type on risk of recurrent gout attacks: an Internet-Based Case-Crossover study. Am J Med. 2014;127(4):311–318. doi:10.1016/j.amjmed.2013.12.019.
- Wang W, Bhole VM, Krishnan E. Chronic kidney disease as a risk factor for incident gout among men and women: retrospective cohort study using data from the framingham heart study. BMJ Open. 2015;5(4):e006843–e006843. doi:10.1136/bmjopen-2014-006843.
- Major TJ, Topless RK, Dalbeth N, et al. Evaluation of the diet wide contribution to serum urate levels: meta-Analysis of population based cohorts. BMJ. 2018;363:k3951. doi:10.1136/bmj.k3951.
- Messerli FH, Frohlich ED, Dreslinski GR, et al. Serum uric acid in essential hypertension: an indicator of renal vascular involvement. Ann Intern Med. 1980;93(6):817–821. doi:10.7326/0003-4819-93-6-817.
- Yip K, Cohen RE, Pillinger MH. Asymptomatic hyperuricemia: is it really asymptomatic? Curr Opin Rheumatol. 2020;32(1):71–79. doi:10.1097/BOR.0000000000000679.
- Burghen GA, Givens JR, Kitabchi AE. Correlation of hyperandrogenism with hyperinsulinism in polycystic ovarian disease. J Clin Endocrinol Metab. 1980;50(1):113–116. doi:10.1210/jcem-50-1-113.
- Mazidi M, Katsiki N, Mikhailidis DP, et al. The link between insulin resistance parameters and serum uric acid is mediated by adiposity. Atherosclerosis. 2018;270:180–186. doi:10.1016/j.atherosclerosis.2017.12.033.
- Novak S, Melkonian AK, Patel PA, et al. Metabolic syndrome-related conditions among people with and without gout: prevalence and resource use. Curr Med Res Opin. 2007;23(3):623–630. doi:10.1185/030079906X167651.
- Choi HK, Ford ES. Prevalence of the metabolic syndrome in individuals with hyperuricemia. Am J Med. 2007;120(5):442–447. doi:10.1016/j.amjmed.2006.06.040.
- Goodarzi MO, Korenman SG. The importance of insulin resistance in polycystic ovary syndrome. Fertil Steril. 2003;80(2):255–258. doi:10.1016/S0015-0282(03)00734-9.
- El Ridi R, Tallima H. Physiological functions and pathogenic potential of uric acid: A review. J Adv Res. 2017;8(5):487–493. doi: 10.1016/j.jare.2017.03.003.
- Nishino T, Okamoto K. Mechanistic insights into xanthine oxidoreductase from development studies of candidate drugs to treat hyperuricemia and gout. J Biol Inorg Chem. 2015;20(2):195–207. doi:10.1007/s00775-014-1210-x.
- Mandal AK, Mount DB. The molecular physiology of uric acid homeostasis. Annu Rev Physiol. 2015;77(1):323–345. doi:10.1146/annurev-physiol-021113-170343.
- Oda M, Satta Y, Takenaka O, et al. Loss of urate oxidase activity in hominoids and its evolutionary implications. Mol Biol Evol. 2002;19(5):640–653. doi:10.1093/oxfordjournals.molbev.a004123.
- Xu L, Shi Y, Zhuang S, et al. Recent advances on uric acid transporters. Oncotarget. 2017;8(59):100852–100862. doi:10.18632/oncotarget.20135.
- Sorensen LB. Role of the intestinal tract in the elimination of uric acid. Arthritis Rheum. 1965;8(5):694–706. doi:10.1002/art.1780080429.
- Maiuolo J, Oppedisano F, Gratteri S, et al. Regulation of uric acid metabolism and excretion. Int J Cardiol. 2016;213:8–14. doi:10.1016/j.ijcard.2015.08.109.
- Montague CT, O’Rahilly S. The perils of portliness: causes and consequences of visceral adiposity. Diabetes. 2000;49(6):883–888. doi:10.2337/diabetes.49.6.883.
- Luque-Ramírez M, Alvarez-Blasco F, Uriol Rivera MG, et al. Serum uric acid concentration as non-Classic cardiovascular risk factor in women with polycystic ovary syndrome: effect of treatment with Ethinyl-Estradiol plus cyproterone acetate versus metformin. Hum Reprod. 2008;23(7):1594–1601. doi:10.1093/humrep/den095.
- Choi HK, McCormick N, Lu N, et al. Population impact attributable to modifiable risk factors for hyperuricemia. Arthritis Rheumatol. 2020;72(1):157–165. doi:10.1002/art.41067.
- Zhang Y,Cai M,Dilimulati D, et al. Correlation between serum uric acid and body fat distribution in patients with polycystic ovary syndrome. Front Endocrinol. 2021;12:782808 10.3389/fendo.2021.782808
- Zhu C, Cui R, Gao M, et al. The associations of serum uric acid with obesity-related acanthosis nigricans and related metabolic indices. Int J Endocrinol. 2017;2017:5438157. doi:10.1155/2017/5438157.
- Choi HK, Atkinson K, Karlson EW, et al. Obesity, weight change, hypertension, diuretic use, and risk of gout in men. Arch Intern Med. 2005;165(7):742–748. doi:10.1001/archinte.165.7.742.
- Rathmann W, Haastert B, Icks A, et al. Ten-Year change in serum uric acid and its relation to changes in other metabolic risk factors in young black and white adults: the CARDIA study. Eur J Epidemiol. 2007;22(7):439–445. doi:10.1007/s10654-007-9132-3.
- Chu NF, Wang DJ, Liou SH, et al. Relationship between hyperuricemia and other cardiovascular disease risk factors among adult males in Taiwan. Eur J Epidemiol. 2000;16(1):13–17. doi:10.1023/a:1007654507054.
- Ogura T, Matsuura K, Matsumoto Y, et al. Recent trends of hyperuricemia and obesity in japanese male adolescents, 1991 through 2002. Metabolism. 2004;53(4):448–453. doi:10.1016/j.metabol.2003.11.017.
- Cook DG, Shaper AG, Thelle DS, et al. Serum uric acid, serum glucose and diabetes: relationships in a population study. Postgrad Med J. 1986;62(733):1001–1006. doi:10.1136/pgmj.62.733.1001.
- Yamashita S, Matsuzawa Y, Tokunaga K, et al. Studies on the impaired metabolism of uric acid in obese subjects: marked reduction of renal urate excretion and its improvement by a Low-Calorie diet. Int J Obes. 1986;10(4):255–264.
- Martinez-Hervas S, Real JT, Ivorra C, et al. Increased plasma xanthine oxidase activity is related to nuclear factor kappa beta activation and inflammatory markers in familial combined hyperlipidemia. Nutr Metab Cardiovasc Dis. 2010;20(10):734–739. doi:10.1016/j.numecd.2009.06.003.
- Buchwald H, Estok R, Fahrbach K, et al. Weight and type 2 diabetes after bariatric surgery: systematic review and Meta-Analysis. Am J Med. 2009;122(3):248–256.e5. doi:10.1016/j.amjmed.2008.09.041.
- Dalbeth N, Chen P, White M, et al. Impact of bariatric surgery on serum urate targets in people with morbid obesity and diabetes: a prospective longitudinal study. Ann Rheum Dis. 2014;73(5):797–802. doi:10.1136/annrheumdis-2013-203970.
- Lu J, Bai Z, Chen Y, et al. Effects of bariatric surgery on serum uric acid in people with obesity with or without hyperuricaemia and gout: a retrospective analysis. Rheumatology. 2021;60(8):3628–3634. doi:10.1093/rheumatology/keaa822.
- Maglio C, Peltonen M, Neovius M, et al. Effects of bariatric surgery on gout incidence in the swedish obese subjects study: a non-Randomised, prospective, controlled intervention trial. Ann Rheum Dis. 2017;76(4):688–693. doi:10.1136/annrheumdis-2016-209958.
- Yokose C, McCormick N, Rai SK, et al. Effects of Low-Fat, mediterranean, or Low-Carbohydrate weight loss diets on serum urate and cardiometabolic risk factors: a secondary analysis of the dietary intervention randomized controlled trial (DIRECT). Diabetes Care. 2020;43(11):2812–2820. doi:10.2337/dc20-1002.
- Qaseem A, Harris RP, Forciea MA, Clinical Guidelines Committee of the American College of Physicians., et al. For the clinical guidelines committee of the American college of physicians management of acute and recurrent gout: a clinical practice guideline from the American college of physicians. Ann Intern Med. 2017;166(1):58–68. doi:10.7326/M16-0570.
- Hamburger M, Baraf HSB, Adamson TC, et al. 2011 Recommendations for the diagnosis and management of gout and hyperuricemia. Postgrad Med. 2011;123(6 Suppl 1):3–36. doi:10.3810/pgm.2011.11.2511.
- Yamanaka H, Japanese Society of Gout and Nucleic Acid Metabolism. Japanese guideline for the management of hyperuricemia and gout: second edition. Nucleosides Nucleotides Nucleic Acids. 2011;30(12):1018–1029. doi:10.1080/15257770.2011.596496.
- Richette P, Doherty M, Pascual E, et al. 2016 Updated EULAR evidence-based recommendations for the management of gout. Ann Rheum Dis. 2017;76(1):29–42. doi:10.1136/annrheumdis-2016-209707.
- Zhu Y, Zhang Y, Choi HK. The serum urate-lowering impact of weight loss among men with a high cardiovascular risk profile: the multiple risk factor intervention trial. Rheumatology (Oxford). 2010;49(12):2391–2399. doi:10.1093/rheumatology/keq256.
- Kawano Y, Okuda N, Minami J, et al. Effects of a Low-Energy diet and an insulin-sensitizing agent on ambulatory blood pressure in overweight hypertensive patients. J Hypertens. 2000;18(10):1451–1455. doi:10.1097/00004872-200018100-00013.
- Song P, Wang H, Xia W, et al. Prevalence and correlates of hyperuricemia in the Middle-Aged and older adults in China. Sci Rep. 2018;8(1):4314. doi:10.1038/s41598-018-22570-9.
- Trifirò G, Morabito P, Cavagna L, et al. Epidemiology of gout and hyperuricaemia in Italy during the years 2005-2009: a nationwide population-based study. Ann Rheum Dis. 2013;72(5):694–700. doi:10.1136/annrheumdis-2011-201254.
- Akasaka H, Yoshida H, Takizawa H, et al. The impact of elevation of serum uric acid level on the natural history of glomerular filtration rate (GFR) and its sex difference. Nephrol Dial Transplant. 2014;29(10):1932–1939. doi:10.1093/ndt/gfu197.
- Liu R, Han C, Wu D, et al. Prevalence of hyperuricemia and gout in mainland China from 2000 to 2014: a systematic review and Meta-Analysis. BioMed Res. Int. 2015;2015:1–12. doi:10.1155/2015/762820.
- Barbieri L, Verdoia M, Schaffer A, et al. Impact of sex on uric acid levels and its relationship with the extent of coronary artery disease: a Single-Centre study. Atherosclerosis. 2015;241(1):241–248. doi:10.1016/j.atherosclerosis.2015.03.030.
- Mumford SL, Dasharathy SS, Pollack AZ, et al. Serum uric acid in relation to endogenous reproductive hormones during the menstrual cycle: findings from the BioCycle study. Hum Reprod. 2013;28(7):1853–1862. doi:10.1093/humrep/det085.
- Jung JH, Song GG, Lee YH, et al. Serum uric acid levels and hormone therapy type: a retrospective cohort study of postmenopausal women. Menopause. 2018;25(1):77–81. doi:10.1097/GME.0000000000000953.
- Adamopoulos D, Vlassopoulos C, Seitanides B, et al. The relationship of sex steroids to uric acid levels in plasma and urine. Acta Endocrinol (Copenh). 1977;85(1):198–208. doi:10.1530/acta.0.0850198.
- Wingrove CS, Walton C, Stevenson JC. The effect of menopause on serum uric acid levels in non-obese healthy women. Metabolism. 1998;47(4):435–438. doi:10.1016/S0026-0495(98)90056-7.
- Hak AE, Curhan GC, Grodstein F, et al. Menopause, postmenopausal hormone use and risk of incident gout. Ann Rheum Dis. 2010;69(7):1305–1309. doi:10.1136/ard.2009.109884.
- Sumino H, Ichikawa S, Kanda T, et al. Reduction of serum uric acid by hormone replacement therapy in postmenopausal women with hyperuricaemia. Lancet. 1999;354(9179):650. doi:10.1016/S0140-6736(99)92381-4.
- Ioannou GN, Boyko EJ. Effects of menopause and hormone replacement therapy on the associations of hyperuricemia with mortality. Atherosclerosis. 2013;226(1):220–227. doi:10.1016/j.atherosclerosis.2012.10.044.
- Darlington LG, Ainsworth JG, Blight A, et al. Changes in urate metabolism after castration of patients suffering from carcinoma of the prostate. In: Harkness RA, Elion GB, Zöllner N, editors. Purine and pyrimidine metabolism in man VII. Advances in Experimental Medicine and Biology. Boston, MA: Springer US; 1991. Vol. 309A, pp. 235–238. doi:10.1007/978-1-4899-2638-8_53.
- Pizzichini M, Di Stefano A, Resconi G, et al. Influence of testosterone on purine nucleotide turnover in rat kidney. Horm Metab Res. 1990;22(6):334–338. doi:10.1055/s-2007-1004914.
- Hosoyamada M, Takiue Y, Shibasaki T, et al. The effect of testosterone upon the urate reabsorptive transport system in mouse kidney. Nucleosides Nucleotides Nucleic Acids. 2010;29(7):574–579. doi:10.1080/15257770.2010.494651.
- Puig JG, Mateos FA, Ramos TH, et al. Sex differences in uric acid metabolism in adults: evidence for a lack of influence of estradiol-17 beta (E2). Adv Exp Med Biol. 1986;195(Pt A):317–323. doi:10.1007/978-1-4684-5104-7_54.
- Yahyaoui R, Esteva I, Haro-Mora JJ, et al. Effect of long-term administration of Cross-Sex hormone therapy on serum and urinary uric acid in transsexual persons. J Clin Endocrinol Metab. 2008;
- Woodward OM, Köttgen A, Coresh J, et al. Identification of a urate transporter, ABCG2, with a common functional polymorphism causing gout. Proc Natl Acad Sci U S A. 2009;106(25):10338–10342. doi:10.1073/pnas.0901249106.
- Hoque KM, Dixon EE, Lewis RM, et al. The ABCG2 Q141K hyperuricemia and gout associated variant illuminates the physiology of human urate excretion. Nat Commun. 2020;11(1):2767. doi:10.1038/s41467-020-16525-w.
- Ee PLR, Kamalakaran S, Tonetti D, et al. Identification of a novel estrogen response element in the breast cancer resistance protein (ABCG2) gene. Cancer Res. 2004;64(4):1247–1251. doi:10.1158/0008-5472.can-03-3583.
- Imai Y, Ishikawa E, Asada S, et al. Estrogen-Mediated post transcriptional down-regulation of breast cancer resistance protein/ABCG2. Cancer Res. 2005;65(2):596–604.
- Stener-Victorin E, Padmanabhan V, Walters KA, et al. Animal models to understand the etiology and pathophysiology of polycystic ovary syndrome. Endocr Rev. 2020;41(4):bnaa010. doi:10.1210/endrev/bnaa010.
- Cassano E, Tosto L, Balestrieri M, et al. Antioxidant defense in the follicular fluid of water buffalo. Cell Physiol Biochem. 1999;9(2):106–116. doi:10.1159/000016307.
- Halliwell B. Free radicals and antioxidants: a personal view. Nutr Rev. 1994;52(8 Pt 1):253–265. doi:10.1111/j.1753-4887.1994.tb01453.x.
- Lavy G, Behrman HR, Polan ML. Purine levels and metabolism in human follicular fluid. Hum Reprod. 1990;5(5):529–532. doi:10.1093/oxfordjournals.humrep.a137136.
- Alexiou M, Leese HJ. Purine utilisation, de novo synthesis and degradation in mouse preimplantation embryos. Development. 1992;114(1):185–192. doi:10.1242/dev.114.1.185.
- Wen X, Perrett D, Jones N, et al. High follicular fluid adenosine levels may be pivotal in the metabolism and recycling of adenosine nucleotides in the human follicle. Metabolism. 2010;59(8):1145–1155. doi:10.1016/j.metabol.2009.09.037.
- Ruebel ML, Piccolo BD, Mercer KE, et al. Obesity leads to distinct metabolomic signatures in follicular fluid of women undergoing in vitro fertilization. Am J Physiol Endocrinol Metab. 2019;316(3):E383–E396. doi:10.1152/ajpendo.00401.2018.
- Herman JB,Goldbourt U. Uric acid and diabetes: observations in a population study. Lancet. 1982;2(8292):240–243. doi:10.1016/s0140-6736(82)90324-5 6124672
- Tuomilehto J,Zimmet P,Wolf E, et al. Plasma uric acid level and its association with diabetes mellitus and some biologic parameters in a biracial population of Fiji. Am J Epidemiol. 1988;127(2):321–336. doi:10.1093/oxfordjournals.aje.a114807 3337086
- Nakanishi N, Tatara K, Nakamura K, et al. Risk factors for the incidence of hyperuricaemia: a 6-Year longitudinal study of Middle-Aged japanese men. Int J Epidemiol. 1999;28(5):888–893. doi:10.1093/ije/28.5.888.
- Modan M, Halkin H, Karasik A, et al. Elevated serum uric acid ? A facet of hyperinsulinaemia. Diabetologia. 1987;30(9):713–718. doi:10.1007/BF00296994.
- Rathmann W, Funkhouser E, Dyer AR, et al. Relations of hyperuricemia with the various components of the insulin resistance syndrome in young black and white adults: the CARDIA study. Ann Epidemiol. 1998;8(4):250–261. doi:10.1016/S1047-2797(97)00204-4.
- Chou P, Lin KC, Lin HY, et al. Gender differences in the relationships of serum uric acid with fasting serum insulin and plasma glucose in patients without diabetes. J Rheumatol. 2001;28(3):571–576.
- Kono S, Shinchi K, Imanishi K, et al. Behavioural and biological correlates of serum uric acid: a study of Self-Defence officials in Japan. Int J Epidemiol. 1994;23(3):517–522. doi:10.1093/ije/23.3.517.
- Okada M, Ueda K, Omae T, et al. The relationship of serum uric acid to hypertension and ischemic heart disease in hisayama population, Japan. J Chronic Dis. 1982;35(3):173–178. doi:10.1016/0021-9681(82)90137-0.
- Facchini F, Chen YD, Hollenbeck CB, et al. Relationship between resistance to insulin-mediated glucose uptake, urinary uric acid clearance, and plasma uric acid concentration. JAMA. 1991;266(21):3008–3011.
- Muscelli E, Natali A, Bianchi S, et al. Effect of insulin on renal sodium and uric acid handling in essential hypertension. Am J Hypertens. 1996;9(8):746–752. doi:10.1016/0895-7061(96)00098-2.
- Maaten JCT, Voorburg A, Heine RJ, et al. Renal handling of urate and sodium during acute physiological hyperinsulinaemia in healthy subjects. Clin Sci (Lond). 1997;92(1):51–58. doi:10.1042/cs0920051.
- Dessein PH, Shipton EA, Stanwix AE, et al. Beneficial effects of weight loss associated with moderate calorie/carbohydrate restriction, and increased proportional intake of protein and unsaturated fat on serum urate and lipoprotein levels in gout: a pilot study. Ann Rheum Dis. 2000;59(7):539–543. doi:10.1136/ard.59.7.539.
- Enomoto A, Kimura H, Chairoungdua A, et al. Molecular identification of a renal urate–anion exchanger that regulates blood urate levels. Nature. 2002;417(6887):447–452. doi:10.1038/nature742.
- Kuwabara M, Borghi C, Cicero AFG, et al. Elevated serum uric acid increases risks for developing high LDL cholesterol and hypertriglyceridemia: a Five-Year cohort study in Japan. Int J Cardiol. 2018;261:183–188. doi:10.1016/j.ijcard.2018.03.045.
- Lanaspa MA, Sanchez-Lozada LG, Choi Y-J, et al. Uric acid induces hepatic steatosis by generation of mitochondrial oxidative stress. J Biol Chem. 2012;287(48):40732–40744. doi:10.1074/jbc.M112.399899.
- Cicerchi C, Li N, Kratzer J, et al. Uric acid-dependent inhibition of AMP kinase induces hepatic glucose production in diabetes and starvation: evolutionary implications of the uricase loss in hominids. Faseb J. 2014;28(8):3339–3350. doi:10.1096/fj.13-243634.
- Hardie DG, Corton J, Ching YP, et al. Regulation of lipid metabolism by the AMP-Activated protein kinase. Biochem Soc Trans. 1997;25(4):1229–1231. doi:10.1042/bst0251229.
- Barroso E, Rodríguez-Calvo R, Serrano-Marco L, et al. The PPARβ/δ activator GW501516 prevents the down-regulation of AMPK caused by a high-fat diet in liver and amplifies the PGC-1α-Lipin 1-PPARα pathway leading to increased fatty acid oxidation. Endocrinology. 2011;152(5):1848–1859. doi:10.1210/en.2010-1468.
- Tsushima Y, Nishizawa H, Tochino Y, et al. Uric acid secretion from adipose tissue and its increase in obesity. J Biol Chem. 2013;288(38):27138–27149. doi:10.1074/jbc.M113.485094.
- Sautin YY, Nakagawa T, Zharikov S, et al. Adverse effects of the classic antioxidant uric acid in adipocytes: NADPH oxidase-mediated oxidative/nitrosative stress. Am J Physiol Cell Physiol. 2007;293(2):C584–C596. doi:10.1152/ajpcell.00600.2006.
- Oberbach A, Neuhaus J, Schlichting N, et al. Sleeve gastrectomy reduces xanthine oxidase and uric acid in a rat model of morbid obesity. Surg Obes Relat Dis. 2014;10(4):684–690. doi:10.1016/j.soard.2013.12.010.
- Nikkilä EA. Control of plasma and liver triglyceride kinetics by carbohydrate metabolism and insulin. Adv Lipid Res. 1969;7:63–134. doi:10.1016/b978-0-12-024907-7.50009-9.
- van den Berghe G, Bronfman M, Vanneste R, et al. The mechanism of adenosine triphosphate depletion in the liver after a load of fructose. A kinetic study of liver adenylate deaminase. Biochem J. 1977;162(3):601–609. doi:10.1042/bj1620601.
- Gresser U, Gathof BS, Gross M. Benzbromarone and fenofibrate are lipid lowering and uricosuric: a possible key to metabolic syndrome? Adv Exp Med Biol. 1994;370:87–90. doi:10.1007/978-1-4615-2584-4_22.
- Baldwin W, McRae S, Marek G, et al. Hyperuricemia as a mediator of the proinflammatory endocrine imbalance in the adipose tissue in a murine model of the metabolic syndrome. Diabetes. 2011;60(4):1258–1269. doi:10.2337/db10-0916.
- Athyros VG, Elisaf M, Papageorgiou AA, et al. Effect of statins versus untreated dyslipidemia on serum uric acid levels in patients with coronary heart disease: a subgroup analysis of the greek atorvastatin and Coronary-Heart-disease evaluation (GREACE) study. Am J Kidney Dis. 2004;43(4):589–599. doi:10.1053/j.ajkd.2003.12.023.
- Milionis HJ, Kakafika AI, Tsouli SG, et al. Effects of statin treatment on uric acid homeostasis in patients with primary hyperlipidemia. Am Heart J. 2004;
- Agarwal A, Aponte-Mellado A, Premkumar BJ, et al. The effects of oxidative stress on female reproduction: a review. Reprod Biol Endocrinol. 2012;10(1):49. doi:10.1186/1477-7827-10-49.
- Griendling KK, Camargo LL, Rios FJ, et al. Oxidative stress and hypertension. Circ Res. 2021;128(7):993–1020. doi:10.1161/CIRCRESAHA.121.318063.
- Houstis N, Rosen ED, Lander ES. Reactive oxygen species have a causal role in multiple forms of insulin resistance. Nature. 2006;440(7086):944–948. doi:10.1038/nature04634.
- Prasad S, Gupta SC, Tyagi AK. Reactive oxygen species (ROS) and cancer: role of antioxidative nutraceuticals. Cancer Lett. 2017;387:95–105. doi:10.1016/j.canlet.2016.03.042.
- Apel K, Hirt H. REACTIVE OXYGEN SPECIES: metabolism, oxidative stress, and signal transduction. Annu Rev Plant Biol. 2004;55(1):373–399. doi:10.1146/annurev.arplant.55.031903.141701.
- Ames BN, Cathcart R, Schwiers E, et al. Uric acid provides an antioxidant defense in humans against oxidant- and Radical-Caused aging and cancer: a hypothesis. Proc Natl Acad Sci U S A. 1981;78(11):6858–6862. doi:10.1073/pnas.78.11.6858.
- Sautin YY, Johnson RJ. Uric acid: the Oxidant-Antioxidant paradox. Nucleosides Nucleotides Nucleic Acids. 2008;27(6):608–619. doi:10.1080/15257770802138558.
- Ali N, Perveen R, Rahman S, et al. Prevalence of hyperuricemia and the relationship between serum uric acid and obesity: a study on bangladeshi adults. Plos One. 2018;13(11):e0206850. doi:10.1371/journal.pone.0206850.
- Kuwabara M, Kuwabara R, Niwa K, et al. Different risk for hypertension, diabetes, dyslipidemia, and hyperuricemia according to level of body mass index in japanese and American subjects. Nutrients. 2018;10(8):1011. doi:10.3390/nu10081011.
- Lima WG, Martins-Santos MES, Chaves VE. Uric acid as a modulator of glucose and lipid metabolism. Biochimie. 2015;116:17–23. doi:10.1016/j.biochi.2015.06.025.
- Zeng C, Guo B, Wan Y, et al. The role of lipid profile in the relationship between particulate matters and hyperuricemia: a prospective population study. Environ Res. 2022;214(Pt 2):113865. doi:10.1016/j.envres.2022.113865.
- Cassano V, Crescibene D, Hribal ML, et al. Uric acid and vascular damage in essential hypertension: role of insulin resistance. Nutrients. 2020;12(9):2509. doi:10.3390/nu12092509.
- Borghi C, Rosei EA, Bardin T, et al. Serum uric acid and the risk of cardiovascular and renal disease. J Hypertens. 2015;33(9):1729–1741; discussion 1741. doi:10.1097/HJH.0000000000000701.
- Berg AH, Lin Y, Lisanti MP, et al. Adipocyte differentiation induces dynamic changes in NF-κB expression and activity. Am J Physiol Endocrinol Metab. 2004;287(6):E1178–E1188. doi:10.1152/ajpendo.00002.2004.
- Furukawa S, Fujita T, Shimabukuro M, et al. Increased oxidative stress in obesity and its impact on metabolic syndrome. J Clin Invest. 2004;114(12):1752–1761. doi:10.1172/JCI21625.
- Johnson RJ, Nakagawa T, Sanchez-Lozada LG, et al. Sugar, uric acid, and the etiology of diabetes and obesity. Diabetes. 2013;62(10):3307–3315. doi:10.2337/db12-1814.
- Gherghina M-E, Peride I, Tiglis M, et al. Uric acid and oxidative stress—relationship with cardiovascular, metabolic, and renal impairment. Int J Mol Sci. 2022;23(6):3188. doi:10.3390/ijms23063188.
- Bagnati M, Perugini C, Cau C, et al. When and why a Water-Soluble antioxidant becomes pro-Oxidant during Copper-Induced Low-Density lipoprotein oxidation: a study using uric acid. Biochem. J. 1999;340(1):143–152. doi:10.1042/0264-6021:3400143.
- Bakker SJL, Gans ROB, ter Maaten JC, et al. The potential role of adenosine in the pathophysiology of the insulin resistance syndrome. Atherosclerosis. 2001;155(2):283–290. doi:10.1016/S0021-9150(00)00745-0.
- Balakrishnan VS, Coles GA, Williams JD. A potential role for endogenous adenosine in control of human glomerular and tubular function. Am J Physiol. 1993;265(4 Pt 2):F504–F510. doi:10.1152/ajprenal.1993.265.4.F504.
- Fransen R, Koomans HA. Adenosine and renal sodium handling: direct natriuresis and renal Nerve-Mediated antinatriuresis. J Am Soc Nephrol. 1995;6(5):1491–1497. doi:10.1681/ASN.V651491.
- Balakrishnan VS, Coles GA, Williams JD. Effects of intravenous adenosine on renal function in healthy human subjects. Am J Physiol. 1996;271(2 Pt 2):F374–F381. doi:10.1152/ajprenal.1996.271.2.F374.
- Osibogun O, Ogunmoroti O, Michos ED. Polycystic ovary syndrome and cardiometabolic risk: opportunities for cardiovascular disease prevention. Trends Cardiovasc Med. 2020;30(7):399–404. doi:10.1016/j.tcm.2019.08.010.
- Palomba S, de Wilde MA, Falbo A, et al. Pregnancy complications in women with polycystic ovary syndrome. Hum Reprod Update. 2015;21(5):575–592. doi:10.1093/humupd/dmv029.
- Boomsma CM, Eijkemans MJC, Hughes EG, et al. A Meta-Analysis of pregnancy outcomes in women with polycystic ovary syndrome. Hum Reprod Update. 2006;12(6):673–683. doi:10.1093/humupd/dml036.
- Masuo K, Kawaguchi H, Mikami H, et al. Serum uric acid and plasma norepinephrine concentrations predict subsequent weight gain and blood pressure elevation. Hypertension. 2003;42(4):474–480. doi:10.1161/01.HYP.0000091371.53502.D3.
- Koratala A, Singhania G, Alquadan KF, et al. Serum uric acid exhibits inverse relationship with estimated glomerular filtration rate. Nephron. 2016;134(4):231–237. doi:10.1159/000448629.
- Kuwabara M, Bjornstad P, Hisatome I, et al. Elevated serum uric acid level predicts rapid decline in kidney function. Am J Nephrol. 2017;45(4):330–337. doi:10.1159/000464260.
- Sidoti A, Nigrelli S, Rosati A, et al. Body mass index, fat free mass, uric acid, and renal function as blood pressure levels determinants in young adults: BMI, FFM, uric acid, eGFR: BP determinants in young adults. Nephrology (Carlton). 2017;22(4):279–285. doi:10.1111/nep.12763.
- Mehta T, Nuccio E, McFann K, et al. Association of uric acid with vascular stiffness in the framingham heart study. Am J Hypertens. 2015;28(7):877–883. doi:10.1093/ajh/hpu253.
- Kuwabara M, Niwa K, Hisatome I, et al. Asymptomatic hyperuricemia without comorbidities predicts cardiometabolic diseases: five-Year japanese cohort study. Hypertension. 2017;69(6):1036–1044. doi:10.1161/HYPERTENSIONAHA.116.08998.
- Roumeliotis S, Roumeliotis A, Dounousi E, et al. Dietary antioxidant supplements and uric acid in chronic kidney disease: a review. Nutrients. 2019;11(8):1911. doi:10.3390/nu11081911.
- Carter J, Child A. Serum uric acid levels in normal pregnancy. Aust N Z J Obstet Gynaecol. 1989;29(3 Pt 2):313–314. doi:10.1111/j.1479-828X.1989.tb01751.x.
- Laughon SK, Catov J, Provins T, et al. Elevated First-Trimester uric acid concentrations are associated with the development of gestational diabetes. Am J Obstet Gynecol. 2009;201(4):402.e1-402–e5. doi:10.1016/j.ajog.2009.06.065.
- Weissgerber TL, Milic NM, Turner ST, et al. Uric acid: a missing link between hypertensive pregnancy disorders and future cardiovascular disease? Mayo Clin Proc. 2015;90(9):1207–1216. doi:10.1016/j.mayocp.2015.05.020.
- El-Eshmawy MM,Ibrahim A,Bahriz R, et al. Serum uric acid/creatinine ratio and free androgen index are synergistically associated with increased risk of polycystic ovary syndrome in obese women. BMC Endocr Disord. 2022;22(1):315.doi:10.1186/s12902-022-01240-y