ABSTRACT
In fish, social interactions are commonly accompanied by acoustic signalling. Males have been found to produce sounds in a large range of species, but female sounds have received considerably less attention. But even in males, there remain significant taxonomical gaps, e.g. in one of the largest fish families, the wrasses. Here, we investigate sound production in territorial males and females of the corkwing wrasse in a field study in Norway. We complemented an in-depth analysis of the visual and acoustic behavioural repertoire of territorial males by descriptions of female behaviours. Males as well as females produced sounds in courtship and agonistic contexts. We recorded four types of sounds and found significant differences between male and female sound properties. We hereby provide one of the first descriptions of sound production in wrasses and, to our knowledge, the first one to also investigate female sound production. Our study shows that wrasses are an underappreciated family when it comes to sound production. With a repertoire of four different calls, corkwing wrasses are remarkably versatile among fishes. Our results highlight the potential and importance of future research about sound communication in neglected taxa, and in both sexes.
Introduction
Very few animals do not produce sounds of some kind. In most animals studied for their sound production, males are historically the predominantly investigated sex (Gerhardt and Huber Citation2002; Drosopoulos and Claridge Citation2005; Ladich Citation2015a; Riebel et al. Citation2019). Males are often the more obviously courting partners, signalling their quality with conspicuous colours, ornamentations or behaviours (Darwin Citation1872; Andersson Citation1994). It is therefore only natural to think that they would also be the part predominantly using acoustic signals, at least in a courtship context. Although this is true in many cases throughout the animal kingdom, there are also remarkable exceptions. For example, in many tropical bird species, females sing just as complex songs as males do, many engaging in duets (Langmore Citation1998; Slater and Mann Citation2004; Hoffmann et al. Citation2019). However, also in species in which female acoustic communication might not be this obvious, it is still worth considering. An increasing body of research describes the role of courtship signalling in females. Outside of birds, female sound production has been found in a diverse range of taxa, e.g. cicadas (Luo and Wei Citation2015), frogs (Roy Citation1997; Emerson and Boyd Citation1999), various monkey species (Sekulic Citation1983; Geissmann Citation2002; Townsend et al. Citation2008), elk bugles (Feighny et al. Citation2006), and fish (Ladich and Schleinzer Citation2020; Liesch and Ladich Citation2020).
The importance of female courtship gets especially clear when males provide a resource like a nesting site or brood care, which can only be provided to a limited number of females (Forsgren et al. Citation2004; Heubel et al. Citation2008). This scenario leads to increased female-female competition and male mate choice, and is commonly found in fish (Sargent et al. Citation1986; Kvarnemo and Ahnesjo Citation1996; Amundsen and Forsgren Citation2001).
In fish, communication can involve all sensory organs: visual, chemical, acoustic, and in some species, electric cues are exchanged between individuals (Van der Sluijs et al. Citation2011). In the last decades, a mounting body of research has shown the prevalence of sound production and acoustic communication in fish, which have long been widely assumed to be silent (Kasumyan Citation2008; Ladich Citation2015). All fish are thought to perceive sound (Popper and Fay Citation2011), and more and more species are described to produce sounds (Kasumyan Citation2008; Ladich Citation2015). In a social context, calling activities during mate attraction, courtship and spawning (Myrberg and Lugli Citation2006; Amorim et al. Citation2015), as well as agonistic interactions (Ladich Citation1997; Ladich and Myrberg Citation2006), are most commonly observed.
As in other taxa, descriptions of sound production in fish usually still only consider male calls, although increasing evidence shows that sound production is often not limited to one sex (Ladich Citation2015a; Ladich and Maiditsch Citation2018; Liesch and Ladich Citation2020). For example, females may produce agonistic sounds (Hawkins and Rasmussen Citation1978; Brantley and Bass Citation1994). In some species, females also produce courtship-related sounds (Ueng et al. Citation2007; Oliveira et al. Citation2014). In croaking gouramis, females are even the only sex calling in a spawning context (Ladich Citation2007). Hence, to truly understand the role of acoustic communication in social interactions, both sexes need to be studied, even if it is more challenging.
Wrasses (Labridae) are a largely blank page in sound research, in spite of being the fifth largest fish family worldwide and second largest in marine environments with more than 500 species described (Westneat and Alfaro Citation2005; Parenti and Randall Citation2011). They play an important role in the ecosystems they inhabit, acting e.g. as corallivores (McIlwain and Jones Citation1997), or cleanerfish (Slobodkin and Fishelson Citation1974). Wrasses are also economically important, because they are used in aquacultures to remove parasites from farmed fish (Blanco Gonzalez and de Boer Citation2017), and their often flamboyant colours and shapes make them popular among divers and aquarists (Wabnitz Citation2003; McCauley et al. Citation2008; Prakash et al. Citation2017). Wrasses have no known structural mechanisms for enhancement of sound pressure perception (i.e. air-filled cavities or the swim bladder close to the inner ear) and relatively small otoliths compared to genera that have specialised adaptations for sound production (Cruz and Lombarte Citation2004). Paxton et al. (Citation2000) suggested that visual communication may have become more important than sound production in this family that mainly inhabits shallow waters, where light is abundant. However, the lack of a known structural mechanisms for the enhancement of sound pressure perception does not necessarily mean that a species is not pressure sensitive (Myrberg and Spires Citation1980; Popper and Fay Citation2011). Furthermore, more recent studies of Hawaiian reef-inhabiting wrasses have unveiled male sound production (Tricas and Boyle Citation2014), especially during courtship and spawning (Boyle and Cox Citation2009). Thus, the question arises whether sound production is more common in wrasses than anticipated.
In this study, we investigated the sound production of a temperate wrasse species, whose males are territorial nest builders, the corkwing wrasse (Symphodus melops L. 1758). We chose the corkwing wrasse as a representative of colourful, shallow-water inhabiting wrasses that are thought to mainly rely on visual communication. The aim of this study was to investigate sound production and to provide behavioural context for the sounds for both sexes of the corkwing wrasse.
Materials and methods
We observed territorial males of the corkwing wrasse throughout one reproductive season (May-July 2016). We recorded all visual and acoustic behaviours of the males at their nests, as well as behaviours and sounds of females visiting the nest sites. We conducted a detailed analysis of the behaviour and the sounds produced during courtship by territorial males. Additionally, we analysed the sounds we recorded of female corkwing wrasses and described the associated behaviours.
Studied species
The corkwing wrasse is a diurnal, temperate fish commonly found in the sublittoral zone (usually <5 m depth) on rocky shores in the eastern Atlantic, ranging from Morocco to the southern and mid-Norwegian coasts, well into the Baltic and on the Azores (Quignard and Pras et al. Citation1986). It can live up to 9 years and reach a size of up to 24 cm in total length (Darwall et al. Citation1992; Knutsen et al. Citation2013). During spawning season, the territorial males build and guard elaborate nests among rocks or crevices using various algae species. Several females can spawn sequentially in these nests and males can go through several breeding cycles in one spawning season (Potts Citation1974; Uglem and Rosenqvist Citation2002). Each breeding cycle consists of three phases: 1. A building phase during which males collect algae from the surroundings and stuff them into each other to construct a bird-like nest while chasing away other males and females; 2. A courtship phase in which males are actively looking out for females, show courtship displays and spawn with females; and 3. A fanning phase, in which males provide brood care by fanning water towards the nest while rarely leaving it and rejecting approaching females (Potts Citation1974, Citation1985). In this species 3–20% of the males are female-mimicking sneaker males (Darwall et al. Citation1992; Halvorsen et al. Citation2016), which try to steal fertilisations by releasing sperm during spawning events between females and a territorial male.
Location and time
The study took place between 21 May 2016 and 6 July 2016 at the Austevoll Research Station (Institute of Marine Research, Bergen, Norway), which covers the corkwing spawning period in this area (Skiftesvik et al., Citation2014). The field experiment was carried out in Haukanesvågen, a bay on the island Austevoll, Western Norway (60°4ʹ 59.8” N; 5°16ʹ 24.9” E; ). This is a well-protected bay, where no large waves arrive or are able to build up, which could cause natural noise and turbidity detrimental to our recordings. The experiment took place in the inner part of the bay in which the water depth was less than 5 m (ca 3900 m2 in total). During the experimental period, the tidal difference between minimum low and maximum high water was ca. 90 cm in this area.
Figure 1. Map of the study location. (a) Location of the island Austevoll in Norway (blue box) and the bay in which the study took place (red box). (b) The bay in which the study took place. The experimental area is marked in yellow
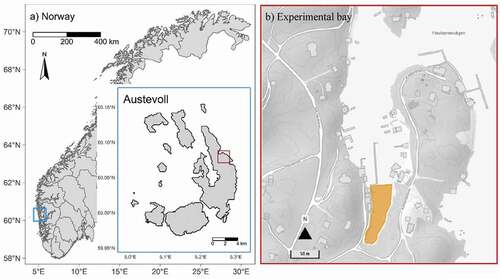
The experimental bay contained a small, unfrequently used marina for recreational boats. An industrial boat for aquaculture was leaving and coming back daily (except Sundays). Otherwise, the bay was very quiet, and unaffected by noise from ferries or boats passing by in the open water outside the bay.
Audio and video recording
We snorkelled in the bay every day starting along the eastern margin and going back in S-lines towards the entrance to find corkwing wrasse nests ()). Upon first discovery, we marked every nest with a numbered red floater (oval shape, 8 × 5 cm, commonly used for fishing nets) approximately 30 cm above the sea floor (). We took the first video and sound recordings at the nest one or 2 days after finding a nest with an active male, after we observed signs of the male being in the courtship phase of the breeding cycle. The probability to record courtship and agonistic interactions, which are typical situations for acoustic communication, is highest during this phase. In total, we recorded video and audio material at 18 nests on two sequential days each.
Figure 2. Schematic drawing of the experimental setup for the video and audio recording of corkwing wrasses (top: male, bottom: female) at their nest sites. Nest sites were marked with a numbered red floater. A GoPro camera tied to a stone was placed 50 cm in front of the entrance of the nest for video recording. A hydrophone was placed next to the nest entrance for audio recording. Illustration of corkwing wrasses: Stein Mortensen
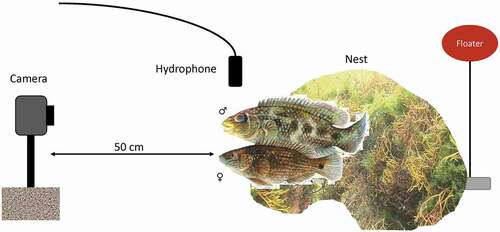
We recorded videos with a GoPro Hero 3 camera tied to a stone, placed ca. 50 cm in front of a nest entrance (). To record sounds, we placed a hydrophone 10–20 cm from the nest entrance, carefully avoiding the hydrophone to block the entrance, thereby disturbing the nest-holding male. Sound recordings were made with SM2+ recorder (Wildlife Acoustics, Inc., Maynard MA, USA; Sampling rate 48 kHz, to 16 bit WAV, 2 Hz high-pass filter, −115 dBV equivalent input noise) with 24 dB gain and a standard hydrophone (HTI-96- 92 MIN: Recording bandwidth: 2 Hz – 48 kHz, Sensitivity −165 dB re 1 V/μPa). We aimed for 30 minutes total time for analysis per recording. Accordingly, after placing the recording equipment, the experimenter started a timer only upon arrival by the shore, where the person waited sitting still for 30 minutes to avoid noise production from swimming sounds.
Identification and analysis of sounds produced by corkwing wrasses
We visually investigated the spectrograms of the recorded sounds using PRAAT 5.4.01 (www.praat.org), marking potential fish sounds. We synchronised the video and audio files using Blender (Blender 2.77, https://www.blender.org/). After synchronisation, we carefully determined for every potential fish sound if there was a corresponding visual clue in one of the fish present in the synchronised videos. Visual clues could be distinguishable movements of the fish body (quiver, sudden strong movement) or opening and closing of the fish mouth at the same time as the sound occurs. In cases in which it was not immediately clear which fish produced a sound, we re-watched the scene in a slower speed (if necessary frame by frame).
We were able to identify four types of sounds unequivocally produced by the corkwing wrasse. We named the sounds after the auditory associations we had when hearing them: grunts, deep grunts, clicks and plops (see Results section Sound production in corkwing wrasse).
(De-)selection of sounds for statistical analyses
Clicks as well as plops are clearly assignable to corkwing wrasses in some scenes, but are problematic to analyse in our field setting, as it was impossible to reliably identify the sound-producing individual for every click or plop (see Results section Single pulse sounds). There are several reasons for this: First, the observed fish moved a lot, often turning their head away from the video camera, so that an observation of their mouth movements was often not possible. This is especially problematic in videos with several fish present at the same time. Second, our recorded sound files, especially those with many fish, contain a high number of click and plop sounds. Many of them have a low amplitude and are not clearly associated with any behaviour of visible fish. Third, plops and especially clicks are loud enough to be heard in the sound files even when there is no fish close to the hydrophone. Trying to identify the sound producing individual for every click or plop turned out to be excessively time-consuming and therefore impossible. We therefore had to exclude them from the statistical behavioural classification of sounds (see below).
Analysis of sound properties
Grunts and deep grunts both consist of repetitive elements. Because these repetitive elements differed considerably for the two grunt types, we named them differently: ‘pulses’ for grunts, and ‘sound cycles’ for deep grunts. We used the program PRAAT 5.4.01 (www.praat.org) for the sound analyses.
We selected high-quality grunts and deep grunts by only including those in the analysis of sound properties, that had a signal-to-noise ratio of 2 or higher for the three pulses/sound cycles with the highest amplitude. To do so, we compared the sound pressure of the pulse/cycle with the third highest amplitude to the sound pressure of three randomly chosen points in the background noise within 0.5 s before the grunt or deep grunt. If the sound pressure of that pulse/cycle was at least twice as high as the background noise, we analysed the properties of the grunt or deep grunt. For the analysis of the properties of the grunt types, we considered four parameters: 1. number of pulses/cycles per sound, 2. duration of the sound, 3. number of pulses/cycles per second, 4. dominant frequency.
To quantify the number of pulses/cycles per sound, we marked every discernible pulse/cycle in the wave form of each grunt at the zero crossing following the highest peak in the pulse/cycle and counted the marked zero crossings. To determine the duration of a sound, we measured the time between the marked zero crossings of the first and last discernible pulse/cylcle. To calculate the number of pulses/cycles per second, we divided the number of pulses/cycles by the duration of the sound. To determine the dominant frequency, we investigated the three loudest pulses within a sound for the frequency with the highest sound pressure level and took the average of these three frequencies.
For the analysis of sound properties for clicks and plops, we only used sounds for which we could clearly identify the sound-producing fish. We described clicks and plops using two parameters: 1. Dominant frequency, 2. sound pressure level difference between lower and higher frequencies.
To determine the dominant frequency of the sound, we investigated the power spectrum of the click or plop for the frequency with the highest sound pressure level. We derived the power spectrum from the zero crossing of the waveform between the highest and lowest amplitude. To calculate the sound pressure level difference, we substracted the sound pressure level of the fifth harmonic from the sound pressure level of the dominant frequency.
Comparison of sound properties
For the comparisons of sound properties, we first averaged the data for male sounds on the individual level. We were not able to do this for females, as there was no way of repeatedly identifying individual females in the videos reliably.
We compared the dominant frequency and duration between male grunts and deep grunts to determine differences between the two call types. We compared all sound properties between male and female grunts to test for sex-specific differences. For clicks and plops, we first tested for sex-specific differences of the analysed properties. We then tested for differences between the both types of single-pulse sounds.
For statistical analyses, we first investigated the properties of the tested sounds for normality using Shapiro-Wilk tests. If data were normally distributed according to Shapiro–Wilk test (P > 0.05), we used t-tests to examine the differences in sound properties. If the Shapiro–Wilk test showed a significant deviation from a normal distribution (P < 0.05), we log-transformed the data to achieve normality, or used Mann–Whitney U tests where a normal distribution could not be achieved by data transformation. For the statistical analysis of sounds we used R (Version 3.3.1, https://www.r-project.org/). We assumed a difference between sound properties to be significant if the P-value of the respective test was < 0.05.
Linking sound to behaviour
Quantification of sounds and behaviours
We only used grunts and deep grunts of territorial males for the statistical assignment of sound to behavioural categories. For the behavioural classification of grunts and deep grunts produced by territorial males, we chose a time span of 10 minutes within each 30 minutes recording. The 10 minutes started 5 s before the first scene in which the focal nest-holding male reacted actively towards a female. Within the selected 10 minutes, we counted the respective number of grunts and deep grunts produced by the focal male.
We established an ethogram for territorial males of the corkwing wrasse based on the work of Potts (Citation1974) and Rodrigues et al. (Citation2015). We also added some newly described behaviours (indicated in ). We watched the videos at half speed and counted the occurrences of every behaviour in the selected 10 minutes for every video using JWatcher (http://www.jwatcher.ucla.edu/).
Table 1. Ethogram of territorial males of corkwing wrasses used for quantification of behaviours. The descriptions of behaviours are based on the work of Potts (Citation1974) and Rodrigues et al. (Citation2015), but additionally some new descriptions are included
We counted grunts and deep grunts and quantified visual behaviours in both videos we had of each male. We averaged the resulting numbers before the statistical analysis. One male was only recorded once, because he deserted his nest before we could film him a second time.
Territorial males
We conducted a Principal Component Analysis (PCA) on the averaged numbers of occurrences of every behaviour and the number of grunts and deep grunts to classify the individual behaviours into meaningful categories. We excluded spawning itself from the PCA, because it is the result of courtship and should therefore not be analysed as a part of it. We weighed the observations by the time the male was in sight and active (10 min − Tout − of − sight −Tresting). After analysis of the scree plot, we conducted a principal component factor analysis with four factors and rotated the axes using Varimax rotation method. We considered all behaviours that contributed to a component with a factor loading of > 0.5 to be a meaningful part of that component. The PCA was conducted using JMP 12.2.0 (SAS Institute Inc., https://www.jmp.com).
Females
We did not conduct a similar behavioural analysis for females. Females usually did not spend extended periods of time at the nest, so standardised observations of individual females were impossible. Sometimes, up to 10 females and sneaker males grouped up around a nest. These mixed groups stayed around a nest for a longer time, sometimes even for hours (personal observation). In these cases, we had no reliable way of distinguishing between individual females or sneaker males. We therefore only present circumstantial descriptions of situations in which females grunted.
Clicks and plops
Given the difficulty in assigning an individual to every click and plop, we had to exclude clicks and plops from the statistical analysis for linking sound and behaviour. Instead, we present a description of the behavioural contexts of male and female clicks and plops from situations with clear visual confirmation of the sound production.
Results
Sounds produced by corkwing wrasses
We identified four types of sounds unequivocally produced by the corkwing wrasse. We named the sounds after the auditory associations we had when hearing them. We termed the sounds consisting of multiple repetitive element grunts and deep grunts, and the sounds consisting of single pulses clicks and plops.
Multiple element sounds
The selection of high-quality grunts resulted in the analysis of 172 male grunts from 28 videos of 17 males, 42 male deep grunts from 18 videos of 11 males and 35 female grunts from 12 videos at the nests of 9 males. Averaging the sound properties for each individual male resulted in a sample size of n = 17 for grunts and n = 11 for deep grunts.
Grunts and deep grunts are sounds consisting of multiple repeated elements with their main energy in a frequency range below 300 Hz (). Because of their different structure, we called the repetitive elements ‘pulses’ for grunts and ‘sound cycles’ for deep grunts. A typical male grunt is shown in , a typical male deep grunt in . A typical female grunt is shown in .
Table 2. Characteristics of grunts and deep grunts produced by territorial males and grunts produced by females of the corkwing wrasse. For males, number of sounds analysed is given as total number and the range of sounds analysed per individual in brackets. For males, number of nest sites equal the number of males recorded, for females, it represents the number of nests at which we observed them. Male sound properties were averaged on the individual level before calculating the presented data. Mean ± SD | Median is given for the number of pulses per sound, duration, pulse rate and dominant frequency
Figure 3. Multiple pulse sounds produced by the corkwing wrasse. Wave form (top) and corresponding spectrogram (below). (a) Grunt of a territorial male corkwing wrasse. (b) Deep grunt of a territorial male corkwing wrasse. (c) Grunt of a female corkwing wrasse. Spectrogram settings: 50 dB dynamic range, Hamming window (raised sine-squared), 0.008 s window length (male and female grunt), 0.08 s window length (male deep grunt)
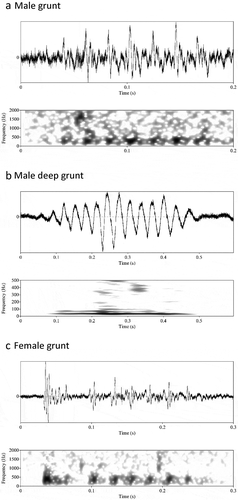
Because of the different structure of grunts and deep grunts, we only compared the sound properties statistically, which were the same in both types of grunt, i.e. dominant frequency and duration. The two male grunt types differ significantly from each other in both properties. Compared to deep grunts, male grunts are of a shorter duration (t-test: t14.92 = 5.44, P < 0.001, CI = −0.47–1.09). The dominant frequency is higher in male grunts than in male deep grunts (Mann Whitney U test: W = 0, N1 = 17, N2 = 11, P < 0.001). presents all measured sound properties for comparison.
Compared to male grunts, female grunts had a significantly lower pulse-rate (t-test: t38.12 = -6.71, P < 0.0001, CI = -21.19- -11.37). Grunts of males and females had a similar dominant frequency (t-test: t45.57 = 1.38, P = 0.17, CI = -0.05- 0.26), duration (t-test: t49.39 = 0.47, P = 0.64, CI = -0.19- 0.26), and number of pulses (Mann Whitney U test: W = 302, N1 = 17, N2 = 35, P = 0.94).
Single-pulse sounds
The selection of clearly assignable clicks resulted in the analysis of 102 male clicks from 29 videos of 19 individuals and 66 female clicks from 20 videos at the nests of 14 males. The selection of clearly assignable plops resulted in the analysis of 73 male plops from 28 videos of 18 videos and 66 female plops from 21 videos at the nests of 14 males. Averaging male sounds for each individual male resulted in a sample size of n = 18 for clicks, and n = 17 for plops.
Clicks are sharp, single-pulse broadband sounds with their main energy in a frequency range of several hundred Hz, while the sound pressure level difference across the harmonics are small (, ). The dominant frequency of clicks was significantly higher in males than in females (Mann Whitney U test: W = 409, N1 = 35, N2 = 66, P = 0.04). However, there were some female clicks for which the dominant frequency was much higher than in most clicks (up to 7200 Hz). We did not see any technical reason to exclude these outliers from the analysis. Clicks did not differ between males and females in the sound pressure level difference between dominant frequency and its 5th harmonic (t-test: t48.4 = 0.84, P = 0.40, CI = −2.17–5.31).
Table 3. Characteristics of clicks and plops produced by territorial males and by females of the corkwing wrasse. For males, number of sounds analysed is given as total number and the range of sounds analysed per individual in brackets. For males, number of nest sites equal the number of males recorded, for females, it represents the number of nests at which we observed them. Male sound properties were averaged on the individual level before calculating the presented data. Mean ± SD | Median is given for the dominant frequency and sound pressure level difference
Figure 4. Single pulse sounds produced by the corkwing wrasse. Wave form (top) and corresponding spectrogram (below). (a) Click produced by a territorial male corkwing wrasse. (b) A group of four plops produced by a territorial male corkwing wrasse. Spectrogram settings: 50 dB dynamic range, Hamming window (raised sine-squared), 0.008 s window length
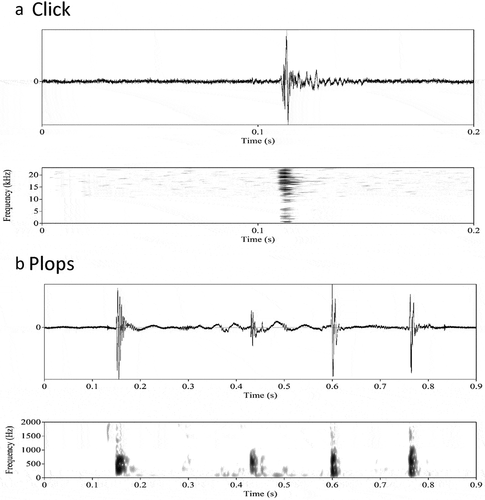
Plops are single-pulse broadband sounds with their main energy in a frequency range around 300 Hz and a steep decline of sound pressure level in the higher harmonics of the dominant frequency (, ). The dominant frequency of plops was significantly higher in females than in males (t-test: t48.52 = 2.00, P = 0.05, CI = −0.00–0.24). Plops did not differ between males and females in the sound pressure level difference between dominant frequency and its 5th harmonic (t-test: t41.99 = −0.97, P = 0.33, CI = −4.35–1.53).
Because males and females differed in the dominant frequency of both clicks and plops, we compared the two types of sounds separately for each sex. The results turned out similar for males and for females: Dominant frequency was significantly higher in clicks than it was for plops (Mann Whitney U test: males: W = 299, N1 = 18, N2 = 17, P < 0.0001; females: W = 3105, N1 = 66, N2 = 66, P > 0.0001). The sound pressure level difference between dominant frequency and its 5th harmonic was significantly larger in clicks than in plops (Mann Whitney U test: males: W = 630, N1 = 18, N2 = 17, P < 0.0001; females: W = 8778, N1 = 66, N2 = 66, P > 0.0001).
Linking sound to behaviour
Categories for visual and acoustic behaviours of territorial males
The Principal Component Analysis (PCA) of all recorded behaviours of territorial males recorded at 18 nest sites revealed four main components that all together explained 65.45% of the observed variance. Based on the combination of behaviours that were correlated to a component by a value of > 0.5, we assigned the components, respectively, to courtship, nest defence, nest maintenance and other behaviours ().
Table 4. Results of the PCA with varimax rotation method performed on behaviours of territorial males recorded at 18 nest sites and corresponding factor loadings of the rotated principal components. PC 1: Courtship (explained variance: 20.03%), PC 2: Nest maintenance (explained variance 18.53%), PC 3: Nest defence (explained variance: 15.63%), PC 4: Other behaviours (explained variance: 11.26%)
Courtship: includes behaviours directly linked to close contact to a female, like the components of ‘courtship swimming’ (Potts Citation1974), as well as ‘circling’, i.e. the behaviour which almost exclusively occurs before and in between spawning events. Additionally, both types of grunts are assigned to the courtship component. ‘Fanning’ is negatively correlated to the courtship component.
Nest maintenance: includes behaviours related to constructing and fixing the nest, as well as ‘feeding’ and ‘spitting sand’. These behaviours usually occur when there is no female around to court for. Accordingly, ‘paying attention’, which means that the male observes his surroundings, also contributes a lot to this factor.
Nest defence: consists of aggressive as well as defensive behaviours towards conspecifics or other animals taking place inside or close proximity to the nest. ‘Turning head towards female’ is part of courtship as well as nest defence, concurring with the description of Potts (Citation1974). Defensive and aggressive behaviours in the scenes we analysed took place almost exclusively between territorial males and females or sneaker males, never between two territorial males. Deep grunts also contribute to the nest-defence component to a lesser extent (factor loading = 0.44).
Other behaviours: includes the newly described behaviours ‘leaving female in nest’ and ‘fake spawn’, i.e. rare behaviours that could not be statistically assigned to one of the other components. Personal observations suggest that they are behaviours occurring during courtship. Behaviours from the nest maintenance component ‘picking up algae’ and ‘pushing algae in nest’ are negatively correlated to this component.
Male grunts and deep grunts
The PCA showed that grunts and deep grunts were only connected to courtship behaviour for males. Males produced grunts typically when they followed a female. The female then reacted by either swimming straight away and evading the male, or engaging with the male by swimming in zig-zag patterns or circles with him around the nest (‘courtship swimming’, Potts Citation1974). In the last case, we sometimes recorded males and females producing grunts alternatingly (Video S1). Grunts are accompanied by a sudden exaggerated swimming movement forward, from which we could infer which individual was the sound-producing one. A typical situation in which a male produced deep grunts was inside the nest closely circling with a female, right before or during the spawning bout of the female (Video S2). Deep grunts were accompanied by a quiver of the fish body.
Female grunts
We observed females to grunt and then dart away from a nest. This behaviour was often followed by an interaction with the nest-holding male. The male often swam after the female and engaged in quick zig-zag or circular swimming closely behind each other in the vicinity of the nest. Sometimes the male and female grunted alternatingly when they were following each other as described above (Video S1). The male then returned to the nest, and if the female followed him there, this usually lead to spawning. Other times after a female grunted and darted away, the male stayed inside the nest and no further interaction followed.
It is noteworthy that the described sequence of behaviours (female grunt – following – (alternating grunts) – spawning) only fully occurred if there was only a single female visiting the nest. If females and sneakers grouped around a nest, we could only observe fragments of the described sequence, e.g. a very short bit of following and a quick return of the male to the nest, or a female grunt and dart interrupted by a different female or sneaker distracting the nest holding male. In these group situations, a clear description of the situation in which the females produced grunts was much harder and we did not attempt to disentangle every behavioural sequence in these videos.
Clicks and plops
In those instances, in which we could clearly assign a click to an individual fish, we observed them rapidly shutting their mouth at the same time, crushing their jaws together. Corkwing wrasses produced very loud clicks when they were attacking or repelling other fish, which could sometimes be heard clearly above the water surface (Video S3). This holds true for both sexes – we observed these loud clicks in male-male, male-female, and female-female agonistic interactions. Additionally, we observed two scenes in which a territorial male attacked brown crabs (Cancer pagurus) coming close to a nest involving the production of clicks. We observed softer clicks in all sound files. Usually we were not able to identify the sound-producing individual for these soft clicks.
On video, we observed fish opening or closing their mouth rapidly synchronously with recorded plops. Territorial males typically produced plops in groups of three or four. When territorial males were producing plops, they were facing other fish with their fins erected, but without attempted physical contact (Video S4). Additionally, we heard soft plop sounds if a male picked up nesting material or food. We observed females producing plops combined with body twitches while swimming around the nests. We also noted female plops occurring as by-product of feeding. However, there were also a high number of unassignable plops in all sound files. In particular, plops that were recorded with low-amplitude were often not possible to assign to a fish that was visible on the video. These may have been produced by fish further away from the nest, or might be produced without externally visible signs of sound production, but we can provide no evidence for this.
Discussion
In this study, we provide evidence that the corkwing wrasse is a soniferous fish with a repertoire of four different calls, and that both males and females produce sound. We show that some of the produced sounds are part of the courtship in males and females and that they differ significantly between the sexes. Additionally, we describe sounds that co-occur with agonistic displays in both sexes.
Sound production in wrasses
The corkwing wrasse is to our knowledge the third wrasse species for which acoustic communication is described today. The local trivial name for it in the study area Austevoll, western Norway, is ‘Helle-klakk’. In Norwegian, ‘Helle’ stands for rocky shores and ‘klakk’ means to click or clap, probably referring to the remarkably powerful agonistic clicks. However, we are not aware of any scientific description of the sounds corkwing wrasses produce. In wrasses, acoustic communication has been described for two Hawaiian reef-inhabiting species, the bird wrasse and the saddle wrasse (Boyle and Cox Citation2009; Tricas and Boyle Citation2014). Like the corkwing wrasse, the bird – and saddle wrasses produce sounds in a courtship and spawning context, but neither female sound production, nor sound production in relation to agonistic behaviour is mentioned for these two species. Male bird wrasses as well as male saddle wrasses produce two types of pulse trains, which differ visually and acoustically from the types of sounds corkwing wrasses produce.
Male grunt sounds
Our study shows that male corkwing wrasses produce two kinds of grunts, which are different from each other, but both clearly associated with courtship. Males produce grunts often in interaction with females. Interestingly, we observed a pattern in these interactions: We observed females to grunt and then dart away from a nest. Then, the nest holding male followed the female and they engaged in courtship swimming. Sometimes the male and female grunted alternatingly while they were following each other (Video S1). We observed successful spawning after some of these interactions. It is likely that the alternating grunts during following occurred more often than we were able to record, because fish often moved outside the area we recorded during this behaviour. Reciprocal sound production of two individuals solidifies our assumption, that grunts are indeed part of acoustic communication. In various fish species, males use acoustic signalling in a courtship context, with purposes like assessment of mate quality, indication of spawning readiness or synchronisation of spawning (Amorim et al. Citation2015). Therefore, both sexes might signal their spawning readiness with the grunting. Grunts could also be an honest signal for quality of the potential mate. In many species, size relates to at least some sound characteristics. For example, male size has been correlated to fundamental frequency (de Jong et al. Citation2007), or number of pulses, fatigue and amplitude (Amorim et al. Citation2013). Amorim et al. (Citation2013) also showed the number of produced sounds can be an honest signal for body condition. Our methods did not allow a size or body condition analysis, we therefore cannot say anything about the information the grunts might carry. However, given the number of publications showing a relation between some aspect of sound production and male quality (reviewed in Amorim et al. Citation2013; Parmentier and Fine Citation2016), we hypothesise that with the appropriate study design one could reveal according relations of importance for mate choice in corkwing wrasses.
Deep grunts could act as a signal to initiate spawning inside the nest, as we heard most of them directly before the spawning bout of the female. Sound production for the synchronisation of spawning was suggested for Atlantic cod Gadus morhua (Rowe and Hutchings Citation2006), Mozambique tilapia Oreochromis mossambicus (Amorim et al. Citation2003), or haddock Melanogrammus aeglefinus (Hawkins and Amorim Citation2000). Another possibility is that deep grunts before spawning trigger females to lay more eggs. Some female fish have the ability to vary their clutch size according to mate quality (Côté and Hunte Citation1989; Spence and Smith Citation2006), or the current situation (e.g. sneaker-biased spawning, Smith and Reichard Citation2005). Assuming a relation of deep grunts to some male quality, they could act as an additional motivation for the female to increase her immediate reproductive investment. Deep grunts also contribute to a lesser extent to the nest defence component. This could be due to the dual function of some behaviours that can be used in courtship as well as nest defence (turning head towards female, stretching fins). If deep grunts contain information about male quality, it would also make evolutionary sense for them to be used as a signal in both courtship and nest defence situations.
Female grunts
Our analyses show that in the corkwing wrasse, females produce grunts in a courtship context. We recorded female grunts when a female approached a nest. The female grunt seems to function as a signal of interest by the female, or as stimulant for the male to start following the female, which can end in spawning. In some cases, however, the male remains in the nest after a female grunt. We also recorded some scenes in which the male and the female grunted alternatingly while they were following each other (see above). The production of female grunts or their properties could be related to properties like size, fecundity or hormonal status. If this is the case, a male could use the grunt to evaluate the quality of the potential mate and therefore decide whether it is worth to engage in the elaborate courtship ritual.
If females produce courtship sounds, there is also the possibility of sneaker males mimicking female sound, as was shown for the plainfin midshipman (Brantley and Bass Citation1994). We cannot completely rule out that some of the sounds we attributed to females were produced by sneaker males. In many situations, however, the behavioural context made it clear that the grunting fish was an actual female (e.g. spawning after grunting).
Female fish are rarely mentioned when it comes to sound production compared to their male counterparts (Ladich Citation2015a; Ladich and Maiditsch Citation2018). However, most female fish possess sound generating mechanisms, even if they are typically less pronounced than in male fish (Casaretto et al. Citation2016). Agonistic sounds that are produced by both sexes are typically rather similar in their characteristics (Myrberg et al. Citation1965; Hawkins and Rasmussen Citation1978; Ladich Citation1989, but see Kéver et al. Citation2012). Descriptions of female courtship sounds are scarce. Exceptions are e.g. seahorses, which exhibit reversed sex-roles (Anderson Citation2009; Chakraborty et al. Citation2014; Oliveira et al. Citation2014), the Japanese croaker (Ueng et al. Citation2007), and croaking gouramis (Ladich Citation2007). Female corkwing wrasses are thus an extension to growing list of female fish as courtship sound producers, which highlights the importance of considering both sexes when it comes to acoustic communication.
Clicks and plops
The clicks that can be heard if one fish attacks or repels another fish or even other animals like brown crabs are remarkably loud and can partly be heard even above water. Clicks have also been described to be part of defensive and territorial behaviour for the skunk loach Botia hovae (Valinski and Rigley Citation1981). If we assume that clicks are produced involving the jawbones, they might be an honest signal for strength in terms of biting force and therefore, if a male is the sound-producer, the ability to protect a nest. The latter aspect could eventually influence female mate choice. Unfortunately, measuring the absolute amplitude of sounds was not possible in this study, as we only had one hydrophone available for our set-up. Combined with the fish constantly changing position, there was no way to determine whether the amplitude of sounds actually differed, or if recorded differences were due to changing in the fish distances to the hydrophone. Agonistic clicks from females are most often heard in situations in which several females and/or sneaker males group around a nest. Females are then often chasing each other or even fighting (snapping and biting). These aggressive interactions are commonly accompanied by loud clicks.
Plops produced by males could be part of either courtship behaviour, agonistic behaviour or both. They often go along with fin-erection in territorial males, a behaviour that is part of courtship as well as nest defence. We would interpret the male behaviours we observed associated with plops as behaviours aiming to attract a female to the nest or chasing other fish away from it. In females we sometimes observed body twitches while producing plops around a nest. They could therefore also act as a combined acoustic and visual courtship signal.
Comparisons between male and female sounds
Our data show that female pulse rate is lower than male pulse rate in grunts. For clicks, dominant frequency is higher in males, whereas in plops, dominant frequency is higher in females. In clicks, this difference was influenced by some female clicks with a very high dominant frequency, which is likely outside of the range of perception for corkwing wrasses (Popper and Fay Citation2011). Differences in calls between males and females could be caused by size differences between the sexes. Female corkwing wrasses are smaller than territorial males on average (unpublished data from the population in Haukanesvågen bay: females 12.7 ± 2.6 cm, territorial males 14.4 ± 2.4 cm), which could explain the higher dominant frequency in plops. Grunt Pulse rate might not be dependent on body size, but rather on muscle contraction rate (assuming muscle contractions to be involved in the production of grunts), and therefore does not have to show the same difference between males and females as dominant frequency in plops (Skoglund Citation1961; Fine et al. Citation2001). An experimental set-up with individually identified and measured fish would be necessary to distinguish between size- and sex-related differences.
In the available literature, comparisons between male and female sound production show very different outcomes. The variability ranges from no measured difference at all (different catfish species, (Ladich Citation1997); haddock (Hawkins and Rasmussen Citation1978, but see Casaretto et al. Citation2016), over differences in calling rates (African jewelfish, Myrberg et al. Citation1965; river bullhead; Ladich Citation1989; croaking gouramis, 2007), to differences in some or all measured characteristics of the sound itself (Japanese croaker, Ueng et al. Citation2007; Zebra mbuna, Simões at al. Citation2008; longsnout seahorse, Oliveira et al. Citation2014; armoured catfish, Hadjiaghai and Ladich Citation2015). This shows that the potential to discover more differences in the communication of male and female corkwing wrasses is high and we encourage pursuing research using this highly interesting fish in terms of both visual and acoustic behaviour.
Conclusions
This study demonstrates that sound production is an integral component of courtship in both male and female corkwing wrasses. The vocal repertoire of the corkwing wrasse consists of four different types of sound. Our work therefore highlights the importance of sound production in this species and inside the family Labridae, which should encourage more research about vocalisation in this speciose genus. Both courtship and aggressive behaviour include acoustic elements, which makes the corkwing wrasse an interesting model species for the study of vocal communication and behaviour in fish. Further work should investigate the functions of the sounds produced by the corkwing wrasse, as questions about the information they carry remain unanswered, and more detailed behavioural analyses of female sound production will deepen the understanding of social behaviour of corkwing wrasses and related species.
Ethical note
The field observations of live fish in this study were in compliance with the Norwegian animal welfare Act (LOV 2009-06-19 nr 97: Lov om dyrevelferd) and did not require further permissions.
Supplemental Material
Download PDF (94.4 KB)Supplemental Material
Download Microsoft Video (AVI) (3.6 MB)Supplemental Material
Download Microsoft Video (AVI) (13.8 MB)Supplemental Material
Download Microsoft Video (AVI) (3.2 MB)Supplemental Material4
Download Microsoft Video (AVI) (6.7 MB)Acknowledgements
We would like to thank Austevoll Research Station and their research staff. In particular, we like to thank Anne Berit Skiftesvik, Reidun Bjelland and Kim Halvorsen for valuable advice and support. We also thank Katharina Foerster for support during the Master`s project this work originated from and Irene Adrian-Kalchhauser for insightful comments on a previous version of this manuscript.
Disclosure statement
The authors declare no competing interests.
Supplementary material
Supplemental data for this article can be accessed here.
Additional information
Funding
References
- Amorim MCP, Vasconcelos RO, Fonseca PJ. 2015. Fish sounds and mate choice. In: Ladich F, editor. Sound communication in fishes. Vienna: Springer Vienna; p. 1–33.
- Amorim MCP, Fonseca PJ, Almada VC. 2003. Sound production during courtship and spawning of Oreochromis mossambicus: male–female and male–male interactions. J Fish Biol. 62(3):658–672. doi:https://doi.org/10.1046/j.1095-8649.2003.00054.x.
- Amorim MCP, Pedroso SS, Bolgan M, Jordão JM, Caiano M, Fonseca PJ. 2013. Painted gobies sing their quality out loud: acoustic rather than visual signals advertise male quality and contribute to mating success. Funct Ecol. 27(2):289–298.
- Amundsen T, Forsgren E. 2001. Male mate choice selects for female coloration in a fish. PNAS. 98(23):13155–13160.
- Anderson PA 2009. The functions of sound production in the lined seahorse, Hippocampus erectus, and effects of loud ambient noise on its behavior and physiology in captive environments. Ph.D. thesis, University of Florida.
- Andersson MB. 1994. Sexual selection. Princeton, NJ: Princeton University Press.
- Blanco Gonzalez E, de Boer F. 2017. The development of the Norwegian wrasse fishery and the use of wrasses as cleaner fish in the salmon aquaculture industry. Fish Sci. 83(5):661–670. doi:https://doi.org/10.1007/s12562-017-1110-4.
- Boyle KS, Cox TE. 2009. Courtship and spawning sounds in bird wrasse Gomphosus varius and saddle wrasse Thalassoma duperrey. J Fish Biol. 75(10):2670–2681.
- Brantley RK, Bass AH. 1994. Alternative male spawning tactics and acoustic signals in the plainfin midshipman fish Porichthys notatus Girard (Teleostei, Batrachoididae). Ethology. 96(3):213–232. doi:https://doi.org/10.1111/j.1439-0310.1994.tb01011.x.
- Casaretto L, Picciulin M, Hawkins AD. 2016. Differences between male, female and juvenile haddock (Melanogrammus aeglefinus L.) sounds. Bioacoustics. 25(2):111–125. doi:https://doi.org/10.1080/09524622.2015.1130647.
- Chakraborty B, Saran A, Kuncolienker DS, Sreepada R, Haris K, Fernandes W. 2014. Characterization of yellow seahorse Hippocampus kuda feeding click sound signals in a laboratory environment: an application of probability density function and power spectral density analyses. Bioacoustics. 23(1):1–14. doi:https://doi.org/10.1080/09524622.2013.796571.
- Côté I, Hunte W. 1989. Male and female mate choice in the redlip blenny: why bigger is better. Anim Behav. 38(1):78–88.
- Cruz A, Lombarte A. 2004. Otolith size and its relationship with colour patterns and sound production. J Fish Biol. 65(6):1512–1525. doi:https://doi.org/10.1111/j.0022-1112.2004.00558.x.
- Darwall WRT, Costello MJ, Donnelly R, Lysaght S. 1992. Implications of life‐history strategies for a new wrasse fishery. J Fish Biol. 41:111–123. doi:https://doi.org/10.1111/j.1095-8649.1992.tb03873.x.
- Darwin C. 1872. The descent of man, and selection in relation to sex. Vol. 2. D.New York: Appleton.
- de Jong K, Bouton N, Slabbekoorn H. 2007. Azorean rock-pool blennies produce size-dependent calls in a courtship context. Anim Behav. 74(5):1285–1292. doi:https://doi.org/10.1016/j.anbehav.2007.02.023.
- Drosopoulos S, Claridge MF. 2005. Insect sounds and communication: physiology, behaviour, ecology, and evolution. Boca Raton, FL: CRC press.
- Emerson SB, Boyd SK. 1999. Mating vocalizations of female frogs: control and evolutionary mechanisms. Brain Behav Evol. 53(4):187–197. doi:https://doi.org/10.1159/000006594.
- Feighny JA, Williamson KE, Clarke JA. 2006. North American elk bugle vocalizations: Male and female bugle call structure and context. J Mammal. 87(6):1072–1077. doi:https://doi.org/10.1644/06-MAMM-A-079R2.1.
- Fine ML, Malloy KL, King C, Mitchell SL, Cameron TM. 2001. Movement and sound generation by the toadfish swimbladder. J Comp Physiol A. 187(5):371–379. doi:https://doi.org/10.1007/s003590100209.
- Forsgren E, Amundsen T, Borg ÅA, Bjelvenmark J. 2004. Unusually dynamic sex roles in a fish. Nature. 429(6991):551–554. doi:https://doi.org/10.1038/nature02562.
- Geissmann T. 2002. Duet-splitting and the evolution of gibbon songs. Biol Rev. 77(1):57–76. doi:https://doi.org/10.1017/S1464793101005826.
- Gerhardt HC, Huber F. 2002. Acoustic communication in insects and anurans: common problems and diverse solutions. Chicago, IL: University of Chicago Press.
- Hadjiaghai O, Ladich F. 2015. Sex-specific differences in agonistic behaviour, sound production and auditory sensitivity in the callichthyid armoured catfish Megalechis thoracata. Plos One. 10(3):e0121219. doi:https://doi.org/10.1371/journal.pone.0121219.
- Halvorsen KT, Sørdalen TK, Olsen EM, Knutsen H, Skiftesvik AB, Durif C, Bjelland RM, Vøllestad LA, Rustand TE. 2016. Male-biased sexual size dimorphism in the nest building corkwing wrasse (Symphodus melops): implications for a size regulated fishery. ICES J Mar Sci. 73(10):2586–2594. doi:https://doi.org/10.1093/icesjms/fsw135.
- Hawkins AD, Amorim MCP. 2000. Spawning sounds of the male haddock, Melanogrammus aeglefinus. Environ Biol Fishes. 59(1):29–41. English. doi:https://doi.org/10.1023/A:1007615517287.
- Hawkins AD, Rasmussen KJ. 1978. The calls of gadoid fish. J Mar Biol Assoc UK. 58(4):891–911. doi:https://doi.org/10.1017/S0025315400056848.
- Heubel KU, Lindström K, Kokko H. 2008. Females increase current reproductive effort when future access to males is uncertain. Biol Lett. 4(2):224–227. doi:https://doi.org/10.1098/rsbl.2007.0630.
- Hoffmann S, Trost L, Voigt C, Leitner S, Lemazina A, Sagunsky H, Abels M, Kollmansperger S, Maat AT, Gahr M. 2019. Duets recorded in the wild reveal that interindividually coordinated motor control enables cooperative behavior. Nat Commun. 10(1):2577. doi:https://doi.org/10.1038/s41467-019-10593-3.
- Kasumyan A. 2008. Sounds and sound production in fishes. J Ichthyol/Vopr Ikhtiol. 48(11):981–1030.
- Kéver L, Boyle KS, Dragičević B, Dulčić J, Casadevall M, Parmentier E. 2012. Sexual dimorphism of sonic apparatus and extreme intersexual variation of sounds in Ophidion rochei (Ophidiidae): first evidence of a tight relationship between morphology and sound characteristics in Ophidiidae. Front Zool. 9(1):34. doi:https://doi.org/10.1186/1742-9994-9-34.
- Knutsen H, Jorde PE, Gonzalez EB, Robalo J, Albretsen J, Almada V. 2013. Climate change and genetic structure of leading edge and rear end populations in a northwards shifting marine fish species, the corkwing wrasse (Symphodus melops). PloS One. 8(6):e67492. doi:https://doi.org/10.1371/journal.pone.0067492.
- Kvarnemo C, Ahnesjo I. 1996. The dynamics of operational sex ratios and competition for mates. Trends Ecol Evol. 11(10):404–408. doi:https://doi.org/10.1016/0169-5347(96)10056-2.
- Ladich F. 1989. Sound production by the river bullhead, Cottus gobio L. (Cottidae, Teleostei). J Fish Biol. 35(4):531–538. doi:https://doi.org/10.1111/j.1095-8649.1989.tb03004.x.
- Ladich F. 1997. Agonistic behaviour and significance of sounds in vocalizing fish. Mar Freshwater Behav Phy. 29(1–4):87–108.
- Ladich F, Myrberg A. 2006. Agonistic behavior and acoustic communication. In: Ladich F, Collin SP, Moller P, editors. Communication in fishes. Enfield: NH: Science Publishers; p. 121–148.
- Ladich F. 2007. Females whisper briefly during sex: context-and sex-specific differences in sounds made by croaking gouramis. Anim Behav. 73(2):379–387. doi:https://doi.org/10.1016/j.anbehav.2006.04.014.
- Ladich F. 2015. Sound communication in fishes. Vol. 4. Vienna: Springer.
- Ladich F. 2015a. Acoustic signalling in female fish. In: Ladich F, editor. Sound communication in fishes. Vienna: Springer Vienna; p. 149–173.
- Ladich F, Maiditsch IP. 2018. Acoustic signalling in female fish: factors influencing sound characteristics in croaking gouramis. Bioacoustics. 27(4):377–390. English. doi:https://doi.org/10.1080/09524622.2017.1359669.
- Ladich F, Schleinzer G. 2020. Sound production in female Trichopsis schalleri (Labyrinth fishes): comparison to males and evolutionary considerations. Bioacoustics. 29(2):123–139. doi:https://doi.org/10.1080/09524622.2018.1555773.
- Langmore NE. 1998. Functions of duet and solo songs of female birds. Trends Ecol Evol. 13(4):136–140. doi:https://doi.org/10.1016/S0169-5347(97)01241-X.
- Liesch N, Ladich F. 2020. Both sexes produce sounds in vocal fish species: testing the hypothesis in the pygmy gourami (labyrinth fishes). J Exp Biol. 223(10):jeb223750. doi:https://doi.org/10.1242/jeb.223750.
- Luo C, Wei C. 2015. Stridulatory sound-production and its function in females of the cicada Subpsaltria yangi. Plos One. 10(2):e0118667. doi:https://doi.org/10.1371/journal.pone.0118667.
- McCauley D, Joyce FJ, Lowenstein J. 2008. Effects of the aquarium fish industry in Costa Rica on populations of the Cortez rainbow wrasse Thalassoma lucasanum. Ciencias Marinas. 34(4):445–451. doi:https://doi.org/10.7773/cm.v34i4.1361.
- McIlwain JL, Jones GP. 1997. Prey selection by an obligate coral-feeding wrasse and its response to small-scale disturbance. Mar Ecol Prog Ser. 155:189–198. doi:https://doi.org/10.3354/meps155189.
- Myrberg A, Lugli M. 2006. Reproductive behavior and acoustical interactions. In: Ladich F, Collin SP, Moller P, editors. Communication in fishes. Enfield: NH: Science Publishers; p. 149–176.
- Myrberg AA, Kramer E, Heinecke P. 1965. Sound production by cichlid fishes. Science. 149(3683):555–558. doi:https://doi.org/10.1126/science.149.3683.555.
- Myrberg AA, Spires JY. 1980. Hearing in damselfishes: an analysis of signal detection among closely related species. J Comp Physiol. 140(2):135–144. doi:https://doi.org/10.1007/BF00606305.
- Oliveira T, Ladich F, Abed‐Navandi D, Souto A, Rosa I. 2014. Sounds produced by the longsnout seahorse: a study of their structure and functions. J Zool. 294(2):114–121.
- Parenti P, Randall JE. 2011. Checklist of the species of the families Labridae and Scaridae: an update. Smithiana Bull. 13:29–44.
- Parmentier E, Fine ML. 2016. Fish sound production: Insights. In: Suthers RA, Fitch WT, Fay RR et al., editors. Vertebrate Sound Production and Acoustic Communication. Springer International Publishing, Cham, Switzerland, p. 19–49.
- Paxton JR, Collin SP, Marshall NJ. 2000. Fish otoliths: do sizes correlate with taxonomic group, habitat and/or luminescence? Philos Trans R Soc Lond B Biol Sci. 355(1401):1299–1303. doi:https://doi.org/10.1098/rstb.2000.0688.
- Popper AN, Fay RR. 2011. Rethinking sound detection by fishes. Hearing Res. 273(1–2):25–36. doi:https://doi.org/10.1016/j.heares.2009.12.023.
- Potts GW. 1974. The colouration and its behavioural significance in the corkwing wrasse, Crenilabrus melops. J Mar Biol Assoc UK. 54(4):925–938. doi:https://doi.org/10.1017/S0025315400057659.
- Potts GW. 1985. The nest structure of the corkwing wrasse, Crenilabrus melops (Labridae: teleostei). J Mar Biol Assoc UK. 65(2):531–546. doi:https://doi.org/10.1017/S002531540005058X.
- Prakash S, Ajith Kumar TT, Raghavan R, Rhyne A, Tlusty MF, Subramoniam T. 2017. Marine aquarium trade in India: challenges and opportunities for conservation and policy. Mar Policy. 77:120–129. doi:https://doi.org/10.1016/j.marpol.2016.12.020.
- Quignard J, Pras A. 1986. Labridae. In: Whitehead PJP, Bauchot M-L, Hureau J-C, editors. Fishes of the North-eastern Atlantic and the Mediterranean. Paris: UNESCO; p. 919–942.
- Riebel K, Odom KJ, Langmore NE, Hall ML. 2019. New insights from female bird song: towards an integrated approach to studying male and female communication roles. Biol Lett. 15(4):20190059. doi:https://doi.org/10.1098/rsbl.2019.0059.
- Rodrigues D, Horta E Costa B, Cabral HN, Gonçalves EJ. 2015. Habitat associations and behavioural patterns of Symphodus spp. (Pisces: labridae). Acta Ethol. 18(3):269–282. doi:https://doi.org/10.1007/s10211-015-0212-1.
- Rowe S, Hutchings JA. 2006. Sound production by Atlantic cod during spawning. Trans Am Fish Soc. 135(2):529–538. doi:https://doi.org/10.1577/T04-061.1.
- Roy D. 1997. Communication signals and sexual selection in amphibians. Curr Sci. 72(12):923–927.
- Sargent RC, Gross MR, Van Den Berghe EP. 1986. Male mate choice in fishes. Anim Behav. 34(2):545–550. doi:https://doi.org/10.1016/S0003-3472(86)80123-3.
- Sekulic R. 1983. The effect of female call on male howling in red howler monkeys (Alouatta seniculus). Int J Primatol. 4(3):291–305. doi:https://doi.org/10.1007/BF02735551.
- Simões JM, Duarte IG, Fonseca PJ, Turner GF, Amorim MC. 2008. Courtship and agonistic sounds by the cichlid fish Pseudotropheus zebra. J Acoust Soc Am. 124(2):1332–1338. doi:https://doi.org/10.1121/1.2945712.
- Skiftesvik AB, Durif CM, Bjelland RM, Browman HI. 2014. Distribution and habitat preferences of five species of wrasse (Family Labridae) in a Norwegian fjord. ICES J Mar Sci. 72(3):890–899. doi:https://doi.org/10.1093/icesjms/fsu211.
- Skoglund CR. 1961. Functional analysis of swim-bladder muscles engaged in sound production in toadfish. J Biophys Biochem Cytol. 10(4):187–200. doi:https://doi.org/10.1083/jcb.10.4.187.
- Slater PJB, Mann NI. 2004. Why do the females of many bird species sing in the tropics? J Avian Biol. 35(4):289–294.
- Slobodkin LB, Fishelson L. 1974. The effect of the cleaner-fish Labroides dimidiatus on the point diversity of fishes on the reef front at eilat. Am Nat. 108(961):369–376. doi:https://doi.org/10.1086/282914.
- Smith C, Reichard M. 2005. Females solicit sneakers to improve fertilization success in the bitterling fish (Rhodeus sericeus). Proc R Soc Lond Ser B. 272(1573):1683–1688.
- Spence R, Smith C. 2006. Mating preference of female zebrafish, Danio rerio, in relation to male dominance. Behav Ecol. 17(5):779–783. doi:https://doi.org/10.1093/beheco/arl016.
- Townsend SW, Deschner T, Zuberbühler K. 2008. Female chimpanzees use copulation calls flexibly to prevent social competition. Plos One. 3(6):e2431. doi:https://doi.org/10.1371/journal.pone.0002431.
- Tricas TC, Boyle KS. 2014. Acoustic behaviors in Hawaiian coral reef fish communities. Mar Ecol Prog Ser. 511:1–16. doi:https://doi.org/10.3354/meps10930.
- Ueng J-P, Huang B-Q, Mok H-K. 2007. Sexual differences in the spawning sounds of Japanese croaker, Argyrosomus japonicus (Sciaenidae). Zool Stud. 46(1):103.
- Uglem I, Rosenqvist G. 2002. Nest building and mating in relation to male size in corkwing wrasse, Symphodus melops. Environ Biol Fishes. 63(1):17–25. doi:https://doi.org/10.1023/A:1013886112191.
- Valinski W, Rigley L. 1981. Function of sound production by the skunk loach Botia horae (Pisces, Cobitidae). Zeitschrift für Tierpsychologie. 55(2):161–172. doi:https://doi.org/10.1111/j.1439-0310.1981.tb01266.x.
- Van der Sluijs I, Gray SM, Amorim MCP, Barber I, Candolin U, Hendry AP, Krahe R, Maan ME, Utne-Palm AC, Wagner H-J. 2011. Communication in troubled waters: responses of fish communication systems to changing environments. Evol Ecol. 25(3):623–640. doi:https://doi.org/10.1007/s10682-010-9450-x.
- Wabnitz C. 2003. From ocean to aquarium: the global trade in marine ornamental species. Stevenage, UK: UNEP/Earthprint; p. 17.
- Westneat MW, Alfaro ME. 2005. Phylogenetic relationships and evolutionary history of the reef fish family Labridae. Mol Phylogen Evol. 36(2):370–390. doi:https://doi.org/10.1016/j.ympev.2005.02.001.