Abstract
Succinobucol is a phenolic antioxidant with anti-inflammatory and antiplatelet effects. Given the importance of oxidant stress in modulating platelet–platelet and platelet–vessel wall interactions, the aim of this study was to establish if antioxidant activity was responsible for the antiplatelet activity of succinobucol. Platelet aggregation in response to collagen and adenosine diphosphate (ADP) was studied in rabbit whole blood and platelet-rich plasma using impedance aggregometry. The effect of oxidant stress on aggregation, platelet lipid peroxides, and vascular tone was studied by incubating platelets, washed platelets or preconstricted rabbit iliac artery rings respectively with a combination of xanthine and xanthine oxidase (X/XO). To study the effect of succinobucol in vivo, anaesthetized rats were injected with up to 150 mg/kg succinobucol and aggregation measured in blood removed 15 mins later. Succinobucol (10−5–10−4 M) significantly attenuated platelet aggregation to collagen and ADP in whole blood and platelet-rich plasma. X/XO significantly increased aggregation to collagen and platelet lipid peroxides and this was reversed by succinobucol. Addition of X/XO to denuded rabbit iliac arteries caused a dose-dependent relaxation which was significantly inhibited by succinobucol. In vivo administration up to 150 mg/kg had no effect on heart rate or mean arterial blood pressure but significantly inhibited platelet aggregation to collagen ex vivo. In conclusion, succinobucol displays anti-platelet activity in rabbit and rat blood and reverses the increase in platelet aggregation in response to oxidant stress.
Introduction
Succinobucol [mono[4-[[1-[[3,5-bis(1,1-dimethylethyl)-4-hydroxyphenyl]thio]-1-methylethyl]thio]-2,6-bis(1,1-dimethylethyl)phenyl] ester] (butanedioc acid) is a phenolic antioxidant derivative of probucol, which has consistently demonstrated improved pharmacokinetics, antiproliferative, and anti-inflammatory effects compared with probucol [Citation1–Citation3]. Succinobucol inhibits cytokine release by monocytes and expression of proinflammatory cell adhesion molecules by endothelial cells (ECs) [Citation4]. However, it has not fulfilled early clinical promise due to deleterious changes in lipid profiles and increased incidence of atrial fibrillation [Citation5]. Our own studies using the pig model of coronary stenting have demonstrated that a 1% succinobucol-eluting stent actually worsened the extent of neointima formation and vascular inflammation, possibly due to toxic effects on smooth muscle and ECs observed in vitro [Citation6]. Despite disappointing clinical results, experimental studies using derivatized compounds with succinobucol as the base molecule are still ongoing with efforts directed at improving its therapeutic effects [Citation7,Citation8].
Interestingly, succinobucol has demonstrable antiplatelet activity and in healthy volunteers can reduce aggregation of platelets to ADP and expression of activation-dependent biomarkers such as the PAR-1 thrombin receptor [Citation9,Citation10]. In volunteers with multiple risk factors for coronary artery disease (CAD) administration of 300 mg of succinobucol daily for 12 weeks was sufficient to reduce generation of thromboxane B2 by platelets without affecting EC prostaglandin production [Citation11]. Since the endothelium is a key component in preventing platelet aggregation and adhesion, a lack of effect of succinobucol on the vasodilator and antiaggregatory capacity of the endothelium may be a distinct advantage.
Oxidative stress is widely recognized as contributing to cardiovascular disease progression [Citation12] and can play an important role in triggering platelet/EC activation. This may be either a direct effect of oxidant stress on the platelet [Citation13] or an indirect effect through destruction of labile endothelium-derived vasodilators such as nitric oxide [Citation14]. However, the direct effects of reactive oxygen species (ROS) on platelets are inconsistent with previous studies reporting both pro- and anti-aggregatory effects when platelets are exposed to exogenous ROS [Citation15]. Oxidant stress generated either by platelets themselves via several intracellular sources [Citation16] or by the artery wall can also modulate vascular tone [Citation17] and this has implications for blood flow and thrombus formation within blood vessels.
Given the importance of oxidative stress in modulating platelet–platelet and platelet–vessel wall interactions, we hypothesized that the antiplatelet effects of succinobucol may be mediated through free-radical scavenging or protecting the platelet against the effects of oxidant stress. In the present study, we aimed to study the mechanism underlying the antiplatelet effects of succinobucol in vitro and ex vivo.
Materials and methods
Animals
Male New Zealand White (NZW) rabbits and Sprague–Dawley (SD) rats used in this study were housed at the University of Glasgow and maintained on 12 hour cycles of light and dark and at ambient temperature. All procedures conformed to the Guide for the Care and Use of Laboratory Animals published by the US National Institutes of Health (NIH Publication No. 85–23, revised 1996) and Directive 2010/63/EU of the European Parliament. Rabbits (2.5–3 kg) were euthanized by injection of sodium pentobarbital (200 mg/kg) via the marginal ear vein. Blood was removed from the pulmonary artery into syringes containing 3.8% sodium citrate (1:10 ratio) for whole blood aggregometry and platelet-rich plasma (PRP) preparation. Iliac arteries were removed and prepared for isometric tension studies. Rats were used to study the effects of succinobucol on platelet aggregation ex vivo.
Platelet aggregometry
Impedance aggregometry was studied using a Chrono-log, Model 590 aggregometer and responses recorded on a dual-channel chart recorder. Blood (500 µL) was diluted 50:50 in 0.9% saline and warmed for 5 minutes at 37°C with stirring. A baseline was established and aggregation initiated by the addition of collagen (1–10 µg/mL) or adenosine diphosphate (ADP; 3 × 10−6 M–10−5 M) and followed for approximately 7 minutes. Where succinobucol was used, this was added to blood 5 minutes before collagen or ADP. To prepare PRP, blood was centrifuged at 1200 rpm for 10 minutes and the supernatant collected. Aggregation of PRP was induced using 20 µg/mL collagen in the presence or absence of succinobucol. In some experiments, whole blood was exposed to the superoxide anion generating system xanthine (added at 10−4 M) and xanthine oxidase (6 mU/mL). This was incubated with the blood for 10 minutes at 37°C before addition of succinobucol and, 5 minutes later, 5 µg/mL collagen. The incubation periods and the doses of succinobucol, xanthine, and xanthine oxidase used were based on our previous studies [Citation6,Citation18].
Platelet lipid peroxidation levels (TBARS)
Washed platelets were prepared from rabbit PRP by adding 5 mL of PRP to 7 mL of washing buffer (NaCl 140 mM, KCl 0.5 mM, trisodium citrate 12 mM, glucose 10 mM, and saccharose 12.5 mM; pH 6) and centrifuging at 1500 g for 10 minutes. The platelet pellet was subjected to two repeated washes and resuspended in 2 mL Krebs’ solution. Protein concentration in the samples was determined against a bovine serum albumin (BSA) standard curve using the Bradford method. The suspension was divided into two with one tube treated with xanthine (10−4 M) and xanthine oxidase (6 mU/mL), and the other with X/XO plus 10−5 M succinobucol. After incubation at 37°C for 15 minutes, samples were centrifuged at 2000 g for 10 minutes and the supernatant discarded. Platelet pellet proteins were precipitated with 10% (v/v) tricholoroacetic acid (TCA) at 37°C for 15 minutes and the supernatant was analyzed for the presence of thiobarbituric acid (TBA) reactive substances (TBARS). Briefly, the supernatant was mixed with 200 μl of TBA (0.67% w/v) and incubated at 100°C for 10 minutes then cooled, TBARS were quantified against a malondialdehyde (MDA) standard curve (0–20 µM). Each standard was reacted with 200 µl of TBA, followed by incubation at 100°C for 10 minutes. The absorbance of standards and samples was measured concurrently at a wavelength of 544 nm in a FLUOstar OPTIMA microplate reader (BMG Labtech, Germany) and results were quantified as nmol MDA per mg of platelet protein.
Wire myography
Rabbit iliac artery rings from NZW rabbits were cleaned of surrounding connective tissue and the endothelium was removed using forceps. Rings of 2–3 mm were mounted in a small artery wire myograph (Danish Myotec) in Krebs’ solution containing (in mM): 118 NaCl, 25 NaHCO3, 4.7 KCl, 1.2 KH2PO4, 1 MgSO4, 2.5 CaCl2, 11 glucose at 37°C, and continuously gassed with 95%O2 5% CO2. An optimum resting tension of 3 g was applied and rings were equilibrated and sensitized to two additions of 40 mM KCl solution. All rings then received an excess of xanthine (10−4 M) and either vehicle or succinobucol (10−4 M or 10−5 M). Ten minutes later rings were contracted by the addition of 1 µM phenylephrine, and once the level of artery tone had stabilized, XO was added at 3, 6, and 9 mU/mL with 10 minutes between additions. Successful endothelial denudation was measured as a lack of relaxation (<10% of PE-induced tone) to addition of 10−6 M ACh.
Effects on platelet aggregation ex vivo
Male SD (250–310 g) rats were induced with 3% isoflurane in oxygen and maintained throughout the procedure on 1–1.5% isoflurane via a face mask. Level of anesthesia was monitored and once the limb withdrawal reflex was lost, a mid-line incision was made over the neck and the carotid artery and jugular vein were isolated and cannulated with polyethylene cannulae (Harvard Apparatus, Kent, UK) filled with heparinized saline. The carotid cannula was connected to a pressure transducer and data acquisition system (Bio-Pac Student Lab Pro, BioPac Systems, Norfolk, UK). The right jugular vein was used for administration of succinobucol. Succinobucol was prepared in ethanol at a concentration of 10−2 M and diluted in 0.9% saline. Rats received injections of 50, 100, and 150 mg/kg of succinobucol at 15-minute intervals. From the experimental recordings, mean arterial blood pressure (MAP) and heart rate was calculated 1, 10, and 15 minutes after each dose of drug. At the end of the experiment, blood was collected from the cannula and ex vivo platelet aggregation in response to collagen was measured by impedance aggregometry. Control animals received injection of equivalent volumes of ethanol/saline vehicle and blood was removed at the same point after the experiment to measure platelet aggregation.
Materials
Collagen and ADP were from Chronolog, Labmedics Ltd, Manchester, UK. ADP was dissolved in distilled water to prepare a stock solution of 10−3 M. All other chemicals were from Sigma-Aldrich, Poole, Dorset. Succinobucol (previously AGI-1067), the monosuccinic acid ester of probucol, was synthesized by esterification of probucol. The identity of the product was confirmed by NMR spectroscopy and purity was in excess of 99% [Citation6]. Succinobucol is metabolically stable and no significant active metabolites are formed in vivo [Citation19]. Xanthine was dissolved in 1 M NaOH solution and sonicated until clear.
Statistical analysis
Data were tested for normality using Kolmogorov–Smirnov test and all variables were normally distributed. Data are expressed as mean ± SEM, where n is the number of animals used to supply blood or arteries used in the experiments. All statistical analyses were performed using GraphPad Prism 5.0 (La Jolla, CA, USA). Differences in platelet aggregation in whole blood and PRP and platelet lipid peroxidation levels were analyzed using a Student’s paired t-test. Myography data, in vivo measurements of HR and MAP, and ex vivo measurements of platelet aggregation were analyzed by one-way ANOVA with Dunnett’s post hoc test. The value of p < 0.05 was considered statistically significant in all cases.
Results
Effect of succinobucol on platelet aggregation
In whole blood, both collagen and ADP caused a dose-dependent increase in impedance although collagen caused more platelet aggregation within the dose range studied (data not shown). Based on these preliminary experiments, 5 µg/mL collagen and 5 × 10−6 M ADP were selected for all further experiments. All three concentrations of succinobucol caused a significant inhibition of platelet aggregation in response to collagen (). Baseline impedance in response to collagen and the value used to calculate percentage reductions was 15.4 ± 2.1 Ω (n = 12). Succinobucol also caused a significant reduction in aggregation in response to ADP (). Baseline impedance in response to ADP and the value used to calculate percentage reductions was 5.2 ± 0.4 Ω (n = 10). In PRP, addition of collagen at concentrations up to 10 µg/mL resulted in very low changes in impedance (2.4 ± 0.5 Ω with collagen 5 µg/mL and 3.8 ± 0.3 Ω with collagen 10 µg/mL; n = 4) which were insufficient to study an inhibitory effect of succinobucol on platelet aggregation. Consequently, a higher concentration of collagen was used in PRP but succinobucol maintained its ability to significantly reduce aggregation at all three concentrations studied ().
Figure 1. (A) Succinobucol caused a dose-dependent reduction in collagen-induced platelet aggregation in rabbit whole blood. Aggregation was measured by impedance aggregometry and values in the presence of succinobucol are expressed as a percentage of the average value of at least three determinations of impedance in the presence of collagen alone (n = 6; p < 0.05 vs. control). (B) Succinobucol also caused a significant reduction in whole blood aggregation in response to ADP (n = 10; p < 0.05 vs. control). (C) In rabbit platelet-rich plasma, succinobucol also caused a dose-dependent reduction in aggregation compared to collagen alone (n = 5; p < 0.05 vs. control).
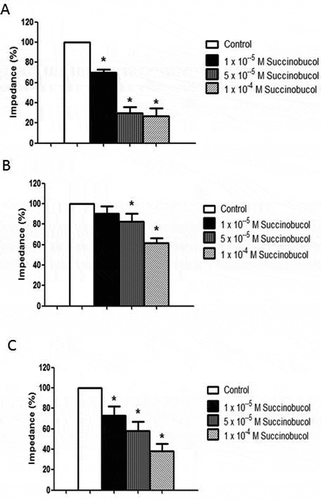
Exposure of rabbit blood to superoxide generated by the combination of X/XO led to an increase in collagen-induced aggregation compared to control (). Preincubating the blood with succinobucol caused a dose-dependent reversal of the effects of X/XO and an overall reduction in aggregation compared to collagen alone ().
Figure 2. Addition of the superoxide generating system xanthine and xanthine oxidase (X/XO) caused a significant increase in collagen-induced aggregation of rabbit whole blood. Succinobucol reversed this increase and in fact lowered aggregation below the value observed with collagen alone (n = 5; p < 0.05 vs. control).
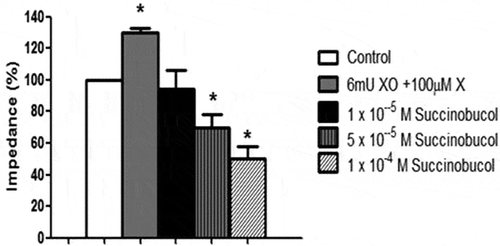
In washed platelets under control conditions, TBARS measured as nmol of MDA per mg of platelet protein were below the level of detection. Incubation with a combination of X/XO (10−4 M xanthine and 6 mU/mL xanthine oxidase) for 15 minutes resulted in an increase in TBARS (21.163.13; n = 4) and there was a non-significant trend towards a reduction in TBARS with the addition of 10−5 M succinobucol (12.15 ± 3.26; n = 4; p > 0.05 vs. X/XO alone).
Wire myography
In denuded rabbit iliac artery rings incubated with xanthine and preconstricted with phenylephrine, addition of three separate concentrations of XO caused a dose-dependent relaxation which peaked at around 45% loss of the induced tone (). Both concentrations of succinobucol significantly attenuated the relaxation to X/XO.
Effect on platelet aggregation ex vivo
Intravenous injection of succinobucol at 50, 100, and 150 mg/kg had no significant effect on either heart rate or MAP ( and ). No other unexpected effects were seen and all 10 rats (5 control animals and 5 experimental animals) survived until the end of the procedure. Blood which was removed 15 minutes after the final injection of succinobucol showed significantly less aggregation in response to collagen at both 5 µg/mL and 20 µg/mL ().
Figure 4. In anaesthetized rats, i.v. injection of three concentrations of succinobucol (50, 100, and 150 mg/kg) with 15 minutes between additions had no significant effect on either heart rate (A) or MAP (B). However, succinobucol did significantly attenuate ex vivo aggregation of platelets in response to two concentrations of collagen (C). n = 5; p < 0.05 vs. vehicle-treated animals.

Discussion
In this study, we have demonstrated that succinobucol exerts an antiplatelet effect in rabbit blood against two different aggregating agents and that it can counteract the activity of ROS in promoting aggregation and modulating vascular tone. In addition, in vivo administration of succinobucol had an anti-aggregatory effect on platelets ex vivo without any effects on MAP or heart rate. We suggest that antioxidant or free-radical scavenging activity may underlie the antiplatelet activity of succinobucol.
A previous study in human blood found an inhibitory effect of succinobucol on platelet aggregation in PRP in response to ADP but not collagen [Citation9] at concentrations between 15 and 90 µg/ml. This equates to a molarity of between 24 and 145 µM which is consistent with our results using between 10 and 100 µM. The study by Serebruany measured platelet activation markers by flow cytometry and reported that reductions in PAR-1 receptors and GP IIb/IIIa may underlie the antiplatelet effects in their study. The parent compound probucol also exhibits antioxidant activity [Citation20] and there is a widespread literature which suggests that such activity can mediate antiplatelet effects [Citation21–Citation23]. Since succinobucol is also an antioxidant, we speculated whether this activity could underlie its anti-aggregatory effect.
In order to study this further, we exposed whole blood to the superoxide generating system xanthine and xanthine oxidase (X/XO). The concentrations of X/XO used in the current study are consistent with similar studies [Citation24] and there are many reports in the literature which demonstrate that ROS derived either from platelets [Citation25], from damaged red blood cells [Citation26] or from addition of free-radical generating systems [Citation27], can augment platelet aggregation in response to stimulating agents such as collagen and ADP. In this study, we found that whole blood aggregated more readily in response to collagen, suggesting that other cell types present in whole blood may contribute to platelet aggregation. Other studies have indicated that the hematocrit can influence aggregation [Citation28] and alcohol can induce release of ADP from RBCs which can increase platelet aggregation in whole blood [Citation29]. Interestingly, many of the agents used to activate platelets such as collagen can themselves induce ROS generation through arachidonic acid (AA) metabolism [Citation30] and ascorbate, a scavenger of superoxide, inhibits aggregation and AA release. We found a significant increase in the aggregation response to collagen when rabbit platelets were pretreated for 10 minutes with X/XO and this was reversed in a dose-dependent manner by succinobucol, suggesting that free-radical scavenging was responsible. In a previous study, we used chemiluminescence (CL) to establish that a combination of 100 µM xanthine and varying concentrations of XO generated SOD-inhabitable CL [Citation18] and here we further verified the effect of X/XO on platelet function by studying lipid peroxidation [Citation23]. In washed rabbit platelets, we found that X/XO significantly increased TBARS above baseline where the level was below detection. Addition of succinobucol showed a trend towards lowered TBARS but this was not significant. However, it provides further evidence that succinobucol protects the platelets against oxidant stress.
We also sought to establish the effect of succinobucol on the artery wall since adhesion of aggregating platelets to areas of damaged endothelium is critical in vivo in mediating not only pathological thrombus formation but also in repair and prevention of blood loss. In preconstricted artery rings, addition of increasing concentrations of the superoxide generating system X/XO caused an endothelium-independent relaxation. In the presence of an intact endothelium, superoxide will destroy nitric oxide and induce constriction [Citation31]. However, some studies have shown that activation of arterial superoxide generating systems such as NADPH oxidase through addition of the substrate (NADH) causes relaxation at low concentrations [Citation32]. In our study, we did not attempt to prevent the conversion of superoxide into hydrogen peroxide, and, since hydrogen peroxide can induce vasodilation in denuded arteries [Citation33,Citation34], it may be that the vasodilation we observed was due to hydrogen peroxide rather than superoxide itself. Nevertheless, the significant inhibition of the vasodilator response caused by succinobucol provides further evidence that scavenging of free radicals may be an important effect of succinobucol in vitro. The significance of this finding is difficult to extrapolate in vivo but it would be interesting in future to study the effects of platelets on vascular tone under control conditions and following treatment with succinobucol.
We also studied the effects of in vivo administration of succinobucol on basic cardiovascular parameters and platelet aggregation ex vivo. Doses of succinobucol up to 150 mg/kg had no effect on MAP or HR in anaesthetized rats. These doses are in line with previous in vivo studies in animals [Citation2], albeit that treatment was chronic in this study. A previous study reported raised systolic blood pressure in humans taking succinobucol chronically [Citation5] but we observed no effect after acute administration in rats, suggesting that effects on blood pressure develop over time. Interestingly, acute administration of succinobucol to rats (up to 150 mg/kg) was sufficient to significantly inhibit platelet aggregation to collagen when blood was removed 15 minutes later. This implies that enough succinobucol is retained within the blood to exert an antiplatelet effect ex vivo or perhaps that the lipophilic drug enters the platelet or modifies its membrane expression of activation markers to reduce aggregation. Although pharmacokinetic studies have established that succinobucol is not a pro-drug of probucol [Citation1], it would be important to establish plasma concentrations of succinobucol in these animals and perhaps also use flow cytometry to measure platelet biomarkers. However, in a study by Serebruany et al. [Citation9], a daily dose of 300 mg/day was reported to result in an average steady-state concentration of 30 ± 5 µg/mL in human blood. In all the in vitro experiments performed here, concentrations between 10−5 and 10−4 M succinobucol were used. When these are converted to µg/ml, based on a formula weight of approximately 617, it gives a concentration of between 6.17 and 61.7 µg/mL, a concentration range similar to that found in human subjects.
Overall, our data suggest that at clinically relevant concentrations succinobucol has an antiplatelet effect in vitro and ex vivo. This is likely mediated through free-radical scavenging or other means which protect the platelet from oxidant species.
Conclusions
In conclusion, we have demonstrated an antiplatelet effect of succinobucol in rabbit whole blood and plasma and that succinobucol is able to reverse the effects of oxidant stress on platelet aggregation and vascular tone. We also show that acute in vivo administration produces an antiplatelet effect ex vivo. Succinobucol was originally synthesized as an antiatherogenic medication and has been studied in phase III trials. However, its potential has not been realized and although experimental studies, particularly on its antioxidant and antihyperglycaemic effects continue, it is unlikely to make an impact as a medicine in humans (reviewed in [Citation19,Citation35]). New derivatives of succinobucol have been developed and these may offer advantages over the parent drug [Citation8]. It would be important to establish the antiplatelet activity of these compounds during development.
Conflict of interest
The authors declare no potential conflict of interests.
References
- Meng CQ, Somers PK, Rachita CL, Holt LA, Hoong LK, Zheng XS, Simpson JE, Hill RR, Olliff LK, Kunsch C. Novel phenolic antioxidants as multifunctional inhibitors of inducible VCAM-1 expression for use in atherosclerosis. Bioorg Med Chem Lett 2002;12(18):2545–2548.
- Sundell CL, Somers PK, Meng CQ, Hoong LK, Suen K-L, Hill RR, Landers LK, Chapman A, Butteiger D, Jones M. AGI-1067: a multifunctional phenolic antioxidant, lipid modulator, anti-inflammatory and antiatherosclerotic agent. J Pharmacol Exp Ther 2003;305(3):1116–1123.
- Murata S, Sundell CL, Lijkwan MA, Balsam LB, Hämmäinen P, Coleman C, York C, Luchoomun J, Suen K-L, Howard R. Effects of AGI-1096, a novel antioxidant compound with anti-inflammatory and antiproliferative properties, on rodent allograft arteriosclerosis. Transplantation 2004;77(10):1494–1500.
- Kunsch C, Luchoomun J, Grey JY, Olliff LK, Saint LB, Arrendale RF, Wasserman MA, Saxena U, Medford RM. Selective inhibition of endothelial and monocyte redox-sensitive genes by AGI-1067: a novel antioxidant and anti-inflammatory agent. J Pharmacol Exp Ther 2004;308(3):820–829.
- Tardif J-C, McMurray JJ, Klug E, Small R, Schumi J, Choi J, Cooper J, Scott R, Lewis EF, L’Allier PL. Effects of succinobucol (AGI-1067) after an acute coronary syndrome: a randomised, double-blind, placebo-controlled trial. Lancet 2008;371(9626):1761–1768.
- Watt J, Kennedy S, McCormick C, Agbani EO, McPhaden A, Mullen A, Czudaj P, Behnisch B, Wadsworth RM, Oldroyd KG. Succinobucol‐eluting stents increase neointimal thickening and peri‐strut inflammation in a porcine coronary model. Catheterization Cardiovasc Interventions 2013;81(4):698–708.
- Jurček O, Ikonen S, Buřičová L, Wimmerová M, Wimmer Z, Drašar P, Horníček J, Galandáková A, Ulrichová J, Kolehmainen ET. Succinobucol’s New Coat—Conjugation with Steroids to Alter Its Drug Effect and Bioavailability. Molecules 2011;16(11):9404–9420.
- Ladopoulou E, Matralis AN, Kourounakis AP. New multifunctional Di-tert-butylphenoloctahydro (pyrido/benz) oxazine derivatives with antioxidant, antihyperlipidemic, and antidiabetic action. J Med Chem 2013;56(8):3330–3338.
- Serebruany V, Malinin A, Scott R. The in vitro effects of a novel vascular protectant, AGI-1067, on platelet aggregation and major receptor expression in subjects with multiple risk factors for vascular disease. J Cardiovasc Pharmacol Ther 2006;11(3):191–196.
- Serebruany VL, Malinin A, Eisert C, Ong S. AGI-1067, a novel vascular protectant, anti-inflammatory drug and mild antiplatelet agent for treatment of atherosclerosis. Expert Rev Cardiovasc Ther 2007;5(4):635–641.
- Serebruany V, Malinin A, Qiu F-H, Xu X-C, Kunsch C, Scott R, Group AS. Selective thromboxane inhibition after vascular protectant AGI-1067: results of assessment of lipoprotein profiles (ALPS) biomarkers in vitro and in vivo substudy. J Thrombosis Thrombolysis 2009;27(4):438–446.
- Delles C, Zimmerli LU, McGrane DJ, Koh-Tan CH, Pathi VL, McKay AJ, Steedman T, Dargie HJ, Hamilton CA, Dominiczak AF. Vascular stiffness is related to superoxide generation in the vessel wall. J Hypertens 2008;26(5):946–955.
- Jang JY, Min JH, Wang SB, Chae YH, Baek JY, Kim M, Ryu J-S, Chang T-S. Resveratrol inhibits collagen-induced platelet stimulation through suppressing NADPH oxidase and oxidative inactivation of SH2 domain-containing protein tyrosine phosphatase-2. Free Radical Biol Med 2015;89:842–851.
- de Meirelles LR, Resende AdC, Matsuura C, Salgado Â, Pereira NR, Cascarelli PG, Mendes‐Ribeiro AC, Brunini T. Platelet activation, oxidative stress and overexpression of inducible nitric oxide synthase in moderate heart failure. Clin Exp Pharmacol Physiol 2011;38(10):705–710.
- Krötz F, Sohn H-Y, Pohl U. Reactive oxygen species players in the platelet game. Arteriosclerosis Thrombosis Vasc Biol 2004;24(11):1988–1996.
- Wachowicz B, Olas B, Zbikowska H, Buczyński A. Generation of reactive oxygen species in blood platelets. Platelets 2002;13(3):175–182.
- Li H, Horke S, Förstermann U. Vascular oxidative stress, nitric oxide and atherosclerosis. Atherosclerosis 2014;237(1):208–219.
- Watt J, Ewart M-A, Greig FH, Oldroyd KG, Wadsworth RM, Kennedy S. The effect of reactive oxygen species on whole blood aggregation and the endothelial cell-platelet interaction in patients with coronary heart disease. Thrombosis Res 2012;130(2):210–215.
- Muldrew KM, Franks AM. Succinobucol: review of the metabolic, antiplatelet and cardiovascular effects. Exp Opin Invest Drugs 2009;18(4):531–539.
- Nomura S, Takahashi N, Inami N, Kajiura T, Yamada K, Nakamori H, Tsuda N. Probucol and ticlopidine: effect on platelet and monocyte activation markers in hyperlipidemic patients with and without type 2 diabetes. Atherosclerosis 2004;174(2):329–335.
- Almeida Rezende B, Carvalho Pereira A, F Cortes S, Soares Lemos V. Vascular effects of flavonoids. Curr Med Chem 2016;23(1):87–102.
- Kwon S-U, Lee H-Y, Xin M, Ji S-J, Cho H-K, Kim D-S, Kim D-K, Lee Y-M. Antithrombotic activity of Vitis labrusca extract on rat platelet aggregation. Blood Coagulation Fibrinolysis Int J Haemostasis Thrombosis 2015;27(2):141–146.
- Olas B, Hamed AI, Oleszek W, Stochmal A. Comparison of biological activity of phenolic fraction from roots of Alhagi maurorum with properties of commercial phenolic extracts and resveratrol. Platelets 2015;26(8):788–794.
- Sill JC, Proper JA, Johnson ME, Uhl CB, Katusic ZS. Reactive oxygen species and human platelet GP IIb/IIIa receptor activation. Platelets 2007;18(8):613–619.
- Jeng J-H, Chen S-Y, Liao C-H, Tung Y-Y, Lin B-R, Hahn L-J, Chang M-C. Modulation of platelet aggregation by areca nut and betel leaf ingredients: roles of reactive oxygen species and cyclooxygenase. Free Radical Biol Med 2002;32(9):860–871.
- Iuliano L, Violi F, Pedersen JZ, Praticò D, Rotilio G, Balsano F. Free radical-mediated platelet activation by hemoglobin released from red blood cells. Arch Biochem Biophys 1992;299(2):220–224.
- Ikeda H, Koga Y, Oda T, Kuwano K, Nakayama H, Ueno T, Toshima H, Michael LH, Entman ML. Free oxygen radicals contribute to platelet aggregation and cyclic flow variations in stenosed and endothelium-injured canine coronary arteries. J Am Coll Cardiol 1994;24(7):1749–1756.
- Goldsmith H, Kaufer E, McIntosh F. Effect of hematocrit on adenosine diphosphate-induced aggregation of human platelets in tube flow. Biorheology 1995;32(5):537–552.
- Abi-Younes SA, Ayers ML, Myers AK. Mechanism of ethanol-induced aggregation in whole blood. Thrombosis Res 1991;63(5):481–489.
- Ghiselli A, Pignatelli P, Sanguigni V, Violi F. Superoxide anion and hydroxyl radical release by collagen-induced platelet aggregation-role of arachidonic acid metabolism. Thromb Haemost 2000;83:485–90.
- Katusic ZS, Schugel J, Cosentino F, Vanhoutte PM. Endothelium-dependent contractions to oxygen-derived free radicals in the canine basilar artery. Am J Physiol-Heart Circulatory Physiol 1993;264(3):H859–H864.
- Didion SP, Faraci FM. Effects of NADH and NADPH on superoxide levels and cerebral vascular tone. Am J Physiol-Heart Circulatory Physiol 2002;282(2):H688–H695.
- Nacitarhan C, Bayram Z, Eksert B, Usta C, Golbasi I, Ozdem SS. The effect of hydrogen peroxide in human internal thoracic arteries: role of potassium channels, nitric oxide and cyclooxygenase products. Cardiovasc Drugs Ther 2007;21(4):257–262.
- Zhang DX, Borbouse L, Gebremedhin D, Mendoza SA, Zinkevich NS, Li R, Gutterman DD. H2O2-induced dilation in human coronary arterioles: role of protein kinase G dimerization and large-conductance Ca2+-activated K+ channel activation. Circ Res 2012;110(3):471–480.
- Lo MC, Lansang MC. Recent and emerging therapeutic medications in type 2 diabetes mellitus: incretin-based, pramlintide, colesevelam, SGLT2 inhibitors, tagatose, succinobucol. Am J Ther 2013;20(6):638–653.