ABSTRACT
There is increasing clinical interest in extracellular vesicles (EV) for diagnostic and treatment purposes. This review provides an overview of bulk immunoassays to analyse EV. Western blot and enzyme-linked immunosorbent assay are still the two predominant bulk immunoassays. Recently, new assays have become available that can detect exposure to EV concentrations that are up to 10,000-fold lower. This is advantageous for applications that detect rare EV. Other important parameters are the detectable concentration range, the required sample volume, whether simultaneous presence of different antigens on a single EV can be detected, size selectivity of each assay and practical considerations. In this review, we will explain the working principles of the traditional and novel assays together with their performance parameters. The most sensitive assays are micro-nuclear magnetic resonance, surface plasmon resonance, and time-resolved fluorescent immunoassay.
Introduction
There is increasing clinical interest in extracellular vesicles (EV), such as “microparticles” and “exosomes”, for diagnostic and treatment purposes. To study EV, bulk assays are widely used because they are affordable, widely available, and applicable to EV research. We define bulk assay as any assay that performs a biochemical analysis on an ensemble of EV. In practice, bulk assays determine one or more biochemical properties from thousands to trillions of EV simultaneously. Because bulk assays analyze an ensemble of EV, bulk assays are generally more sensitive and faster than single EV techniques. A typical disadvantage of bulk assays is that information on the EV concentration, biochemical heterogeneity, and polydispersity is lost [Citation1, Citation2]. We will limit the scope of this review by exclusion of proteomics, lipidomics, nucleotide sequencing assays, and polymerase chain reaction [Citation3-Citation8], enabling us to focus on bulk immunoassays (BIA). An example of a mechanism employed in BIA is the use of a fluorescent antibody conjugate, where the amount of fluorescence is proportional to the number of antigens. Besides fluorescence, other reporters include enzymes, proximity sensors, radioactive isotopes, and metal nanoparticles. In this review, we will discuss the use of BIA for the detection of EV from any origin, including platelet-derived EV.
For this review, a literature study was performed in Scopus (Elsevier, Amsterdam, The Netherlands; May 2016). Included articles (1) contain at least one BIA in the abstract, (2) apply the BIA to study EV, and (3) were published since January 2010. The search resulted in nine different BIA, which include Western blot (WB) and enzyme-linked immunosorbent assay (ELISA), as well as seven newly developed BIA.
Classification and assay description
We will classify the nine BIA by whether they (A) are performed after gel electrophoresis, (B) are performed in suspension, or (C) are immunosorbent assays. See for an overview of the principles of operation of all described assays.
Figure 1. Schematic overview of bulk immunoassays for EV characterization. Western Blot (WB) performs EV lysis, gel electrophoresis (EF) of all protein content, and transfer EF to a membrane for subsequent immuno-labeling. Micro-Nuclear Magnetic Resonance (µNMR) selects EV sizes by filtration, and labels EV with immunomagnetic particles for subsequent detection of the magnetic susceptibility of the sample. Amplified Luminescent Proximity Homogeneous Assay (ALPHA) detects transfer of singlet oxygen from the donor to the acceptor if they are in close proximity, i.e. bound to an EV. Surface Plasmon Resonance (SPR) captures EV on an immuno-functionalized surface, and detects the resulting change in refractive index. The other immunosorbent assays are all variations of the sandwich Enzyme Linked Immuno Sorbent Assay (ELISA), where capture of EV on a functionalized surface is complemented by detection of a different antigen, or epitope. The reporter molecules can be enzymes (ELISA), fluorescent molecules (FLISA, Fluorescent Immuno Sorbent Assay), or phosphorescent molecules (TRFIA, Time-Resolved Fluorescent ImmunoAssay). To overcome the mass transport limitations near a surface, electrohydrodynamic-induced nanoshearing (ac-EHD) generates additional flow near the surface, while the Integrated Microfluidic Exosome Analysis Platform (IMEAP) mobilizes the surface itself by means of immunomagnetic beads.
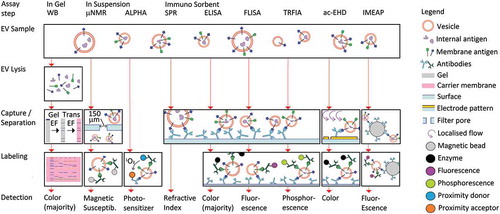
A. Gel electrophoresis-based assays
A.1. Western blot (WB)
WB provides information about the presence and molecular weight of an antigen of interest by gel electrophoresis and subsequent labeling of the target antigen [Citation9].
A commonly applied WB procedure starts with EV lysis. Subsequently, all proteins are separated by gel electrophoresis based on differences in their molecular-weight and charge [Citation10-Citation12]. Thereafter, the antigens are transferred or “blotted” by electrophoresis to a carrier membrane, such as nitrocellulose or polyvinylidene fluoride. Subsequently, the sample is incubated with an antibody against the antigen of interest, followed by a second antibody targeting the first antibody. The second antibody is conjugated to horseradish peroxidase or alkaline phosphatase, which catalyzes a colorimetric reaction in a substrate. Alternatively, either enzyme can be utilized to perform conversion of a substrate into chemiluminescence or fluorescence. Readout of the blot is done through digitization of the blot, or directly by eye.
B Assays in suspension
B.1. Micro-nuclear magnetic resonance (µNMR)
µNMR measures the degree of sample magnetization in response to an applied magnetic field.
In the µNMR protocol, EV are labeled with 7 nm magnetic beads in a two-step labeling protocol inside a microfluidic chip. A 150 nm filter at the entrance port limits the maximum EV size, and a 50 nm pore size filter is used to retain the sample inside the chamber during washing of unbound labels. Subsequently labeled EV are detected with an embedded magnetic coil. Biological samples have negligible magnetic susceptibility, allowing detection of immunomagnetic nanoparticles bound to membrane antigens on EV [Citation13]. This results in a ~1,000-fold more sensitivity improvement over ELISA [Citation13]. Selection of different filters allows changes in the size range.
B.2. Amplified luminescent proximity homogeneous assay (ALPHA)
A proximity assay measures the emitted signal as a function of the proximity of two components of the detection system by means of some energy transfer process.
In the ALPHA system two different types of beads are used. A donor bead releases singlet oxygen (1O2) upon excitation, and an acceptor bead emits light upon uptake of 1O2. Due to the rapid decay time of 1O2 to the stable triplet oxygen (3O2), these beads need to be within 200 nm of each other. Therefore, a signal is only found if both donor and acceptor are held near to each other, for example on two antigens of an EV of interest [Citation14]. The emitted light intensity of these “bead-EV-bead” complexes correlates linearly with the number of EV. The assay can be performed in a 96 well plate format and requires a plate reader dedicated to the proximity luminescent assay.
C. Immunosorbent assays
C.1. Surface plasmon resonance (SPR)
SPR detects the refractive index change due to immune mediated adhesion of EV at a detection surface.
In the basic protocol, the sensor surface is coated with antibody, followed by washing the sensor and blocking any exposed sensor surface by incubation with a protein like bovine serum albumin. Then, the sample is loaded into the sensor, and left to incubate. EV are captured by antibodies at the surface. This capture results in a refractive index change which can be monitored in time. The time data allow the study of kinetics of interaction between EV and the surface. Because the refractive index contrast between buffer and EV is sufficiently large, no reporter is needed for readout [Citation15-Citation18], which is why SPR is sometimes referred to as a label-free technique. The change in refractive index provides information on the amount of captured material [Citation19]. Different commercial systems exist, and each detection system requires dedicated sensor chips. The sensor design allows a single, or up to 96 parallel antigen measurements.
C.2. Enzyme linked immunosorbent assay (ELISA)
The sandwich ELISA performs immune mediated EV capture at a surface and reads out the captured quantity through a secondary labeling procedure. All the immunosorbent assays discussed in the rest of this section are variations of the sandwich ELISA assay.
A sandwich ELISA begins with the coating of a capture antibody to the well(s) of a microplate. EV samples, control samples and, if available, a reference standard containing protein of interest, are all pipetted into different wells. After incubation and washing, a second antibody targeting the captured EV is added. After again incubation and washing, a third antibody targeting the second antibody is added. This third antibody is linked to an enzyme, for example horse radish peroxidase. The amount of enzyme can be measured analogous to WB. Although colorimetric quantification is encountered most frequently, chemiluminescence may be more sensitive [Citation20].
The ELISA method is a benchmark for quantitation of antigens in solution. This quantitation relies on calibration with a standard containing a known concentration of protein of interest, for example a recombinant antigen. However, for intact EV this calibration is of limited value because the diffusion of EV toward the capture surface is 10-100-fold slower than the protein solution used for calibration.
C.3 Fluorescent immunosorbent assay (FLISA)
FLISA is an ELISA-based assay where the captured quantity is determined through a fluorescent antibody conjugate.
A recent example applied to EV is the “EV array” [Citation21, Citation22]. The EV array utilizes slides printed with an array of antibodies to capture EV and then determines the amount of captured EV by labeling with a cocktail of biotinylated CD9, CD63 and CD81 and the use of streptavidin-Cy5 as a reporter molecule. Advantages of the use of a fluorescent reporter molecule instead of an enzyme may be an increase in sensitivity and a less time sensitive protocol. However, the auto fluorescence of the plastic of standard 96 well plates limits the detection of low density antigens. To mitigate this auto fluorescence, the EV array is performed on glass microscopy slides with an “ArrayIt” system to temporarily generate 96 wells.
C.4. Time-resolved fluorescent immunoassay (TRFIA)
TRFIA is an ELISA-based assay where the captured quantity is determined through a phosphorescent antibody conjugate
This exploits the difference in time delay in emission of phosphorescent molecules relative to auto fluorescence of the sample and sample holder. For example, the amount of bound antibody labeled with europium is quantified using a dedicated time delay plate reader [Citation23]. The time delay measurement results in an ~10-fold improvement in sensitivity over ELISA and FLISA due to elimination of the background signals of the sample and the 96-well plate.
C.5. Alternating current electrohydrodynamics-induced nanoshearing (ac-EHD)
ac-EHD is an ELISA-based assay in a microfluidic chip, in which capture of EV is enhanced 3-fold by a local flow induced by an electric field [Citation24].
The electric field induced local flow also causes an increase of shear at the capture surface, which enhances the release of weakly bound EV, thus reducing non-specific signal. Enzyme-based detection has been demonstrated [Citation24], but there is no fundamental limitation to the application of fluorescence or phosphorescence.
C. 6. Immunomagnetic beads in a microchip ELISA (IMEAP)
IMEAP is an ELISA-based assay, where the capture surface has been mobilized onto immunomagnetic beads.
In the microchip, EV-bead complexes are captured through the application of a magnetic field for all washing steps and for sample enrichment. This has been demonstrated in the “Integrated microfluidic exosome analysis platform” (IMEAP) [Citation20]. In IMEAP, chemiluminescent detection is performed by means of a sandwich assay similar to ELISA. If desired, the captured EV can be lysed in a follow up analysis, and the released proteins are recaptured, but now with magnetic beads conjugated to the antibody of interest prior to detection.
The main advantage is that the capture surface is made mobile, thus potentially increasing the contact between capture surface and sample, and thus reducing the limitations imposed in ELISA by the relatively slow diffusion of EV. However, it is unknown what the gain is, and we encountered only one immunomagnetic bead capture assay.
Comparison of BIA performance
To determine the usefulness of BIA in a clinical setting, we have compared the described methods with regard to their limit of detection, the concentration range that can be handled, the required sample volume, and the ability to perform multiple experiments in parallel. The results of this comparison are shown in and discussed below.
Table 1. Comparison of bulk immunoassays for extracellular vesicles (EV).
Limit of detection
For BIA, we define the limit of detection as the minimal required EV concentration for the signal to reliably exceed the noise. Although the limit of detection is a critical parameter there is no uniform method to define nor determine the limit of detection.
In the literature, the limit of detection is expressed in µg antigen, µg antigen/mL, total number of EV, number of EV/mL, or compared to another assay, such as ELISA. The variation in definitions is most extreme in the case of SPR, where authors have determined the detection limit as number of EV/mL [Citation16], µg EV/mL [Citation17], or number of EV/cm2 [Citation18]. While each of the units for limit of detection are sensible for some of the discussed assays, we selected number of EV/mL because it is definable for all assays. The alternative µg antigen/mL is not definable for all assays, and µg antigen or EV alone can be misleading for methods that do not see the whole sample volume.
To determine the limit of detection of a method, NTA is frequently used to determine the concentration of EV in a reference sample. NTA does not detect the concentration of EV, but the concentration of all particles inside the detection size range (typically 80-500 nm [Citation25]). Furthermore, a wide array of reference samples is used, including EV derived from different cell cultures [Citation13, Citation14, Citation16, Citation17, Citation20, Citation21, Citation23, Citation26] and/or plasma [Citation21, Citation27] were used. All these factors lead to considerable uncertainty in the limit of detection. Therefore, we report the order of magnitude of the limit of detection. The actual limit may still prove to be 1-2 orders of magnitude different if all BIA were to be compared using a uniform definition for limit of detection, and with a single culture cell line and a single antibody clone. The latter two factors are important to ensure that the EV antigen exposure level and the affinity of the antibody do not influence the comparison. Comparison of new BIA assays would greatly be facilitated by standardization of the method to determine the limit of detection.
The reported or calculated detection limits range between 107 and 1012 EV/mL. WB and ELISA are both relatively insensitive, and require - on average - 104-fold higher concentrations of EV than SPR and µNMR to allow detection [Citation13, Citation16].
Dynamic range
The highest detectable EV concentration is the concentration at which the signal is nearest to saturation. At the saturation level, an increase in EV concentration no longer results in an increase in signal. The lowest detectable concentration of EV is the limit of detection, earlier defined as the EV concentration at which the signal is just discernible from noise. The dynamic range is the ratio between the highest and lowest detectable concentration. This parameter is important for quantitative measurements, because with a low dynamic range samples need to be analysed at multiple dilutions, adding time and cost to the assay. Because the dynamic range was not stated in any publication, we derived a lower boundary for dynamic range from the maximum measured signal relative to the limit of detection. The order of magnitude is given, with an uncertainty less than one order of magnitude.
WB has the lowest dynamic range, approximately 100:1. Partially because of this low dynamic range, WB is typically not used quantitatively. TRFIA and SPR have the highest dynamic ranges of 105 and 106, respectively.
Sample volume
Assays with high minimum EV concentration may need to concentrate their samples prior to measurement. Therefore, we define the sample volume as the total (initial) volume required for analysis per measurement. This sample volume varies between 1 and 500 µL for the different BIA and therefore is no limitation for conditioned cell media and commonly used body fluids in EV research, such as blood plasma or urine. However, a sample volume of 500 µL may pose a problem for murine experiments or measurements on neonate blood samples.
Number of targets
This parameter describes the capability to detect a combination of one, two or more antigens on the same EV. Typical single antigen assays are WB, SPR, and µNMR. These assays may be capable of measuring multiple targets in parallel experiments, but this will not prove that EV in the sample expose all targets simultaneously. The sandwich ELISA and the fluorescent and phosphorescent analogues thereof, as well as ALPHA may detect two antigens on the same EV by targeting a different antigen with the primary and secondary antibody. No BIA was found that could measure more than two targets on the same EV.
Parallel experiments
Parallel experiments allow analysis of multiple samples and/or targets, and therefore are advantageous for analysis of biorepositories. For parallel samples, the 96 well plate compatible ELISA and fluorescent/phosphorescent analogues together with the proximity assay Alpha have the best possibilities. For parallel targets, the imaging version of SPR can measure up to 96 different antibodies on a single sample.
Analysis duration
The overall analysis duration is estimated from the described methods in literature and represents an estimate of the time required to prepare and perform an experiment. It includes the time required for sample preparation, surface or bead modifications, sample incubation and the actual detection. Performing a set of parallel experiments does not change this duration for any BIA if the increase in time due to pipetting actions is neglected.
Most BIA require two to four hours to prepare and perform an experiment, but FLISA and TRFIA require 24 and 8 hours, respectively, due to longer sample incubation steps [Citation21, Citation23]. Longer incubation allows more contact between sample and capture surface, and thus longer incubation may benefit the limit of detection for ELISA, SPR, and ac-EHD. However, the sample integrity may be compromised if the time to result becomes too long.
EV size
All discussed BIA have some EV size dependence in their detected signal. For example, in all surface-based immunosorbent assays the transport of EV to the surface is limited by diffusion [Citation17]. Diffusion scales with the inverse of the diameter, so a 50 nm EV will have a 20-fold higher diffusion constant as a 1,000 nm EV. This means that while surface-based immunosorbent assays may detect EV of all sizes, they will detect a lower proportion of larger EV. On the other hand, it is easier to lyse a large EV [Citation28], which means that assays that require lysis of EV may measure proportionally more large EV. For ALPHA, which uses two antigen targets, a larger EV presents a higher chance that a singlet oxygen is absorbed by an acceptor bead. µNMR limits the size of EV in the sample to 50-150 nm by means of filtration [Citation13]. Note that this latter size range is inferred from the filter pore diameters, and has not been verified. In fact, for none of the assays described in this review size selectivity has been thoroughly studied, although a theoretical basis has been described for SPR [Citation17]. Moreover, in the case of ELISA and its analogues, calibration is needed prior to quantification, in part to correct for the sample volume that comes in contact with the capture surface. The typical reference is a concentration series of recombinant antigen in solution, but this does not account for the 10-100-fold difference in diffusion constant between EV and antigens in suspension.
Differentiation between intravesicular and extravesicular domains
If the assay requires lysis of EV, it is not possible to differentiate between whether the target antigen was found on the outer surface of the EV, or intravesicular. The only assay that requires lysis is WB. For detection of intravesicular antigens, all other assays allow but do not require lysis.
Popularity
Western blot is widely used [Citation9-Citation12, Citation29-Citation73] to confirm the presence of EV, through their proteins, but rarely the concentration of EV. ELISA is the most widely used immunosorbent assay [Citation9, Citation56-Citation76], and detects the concentration of target antigens in a sample. Most other assays discussed in this review were discussed by fewer than three laboratories per assay. SPR has been applied at least five laboratories.
Conclusion
BIA have become an important tool in EV studies. WB and ELISA are established in the field and at least seven more BIA are currently being tested and may become useful for detection and/or biomolecular characterization of EV. Because BIA are mainly used to detect or quantify the presence of specific markers on EV, we expect that the most sensitive assays, µNMR, TRFIA, and SPR, will empower progression of the EV field.
Funding
This work was supported by the Dutch technology foundation STW [Perspectief program Cancer-ID - 14198, VENI - 13681].
Additional information
Funding
References
- Oksvold MP, Kullmann A, Forfang L, Kierulf B, Li M, Brech A, Vlassov AV, Smeland EB, Neurauter A, Pedersen KW. Expression of B-Cell surface antigens in subpopulations of exosomes released from B-cell lymphoma cells. Clinical Therapeutics 2014;36(6):847–862.
- Torregrosa Paredes P, Gutzeit C, Johansson S, Admyre C, Stenius F, Alm J, Scheynius A, Gabrielsson S. Differences in exosome populations in human breast milk in relation to allergic sensitization and lifestyle. Allergy: European Journal of Allergy and Clinical Immunology 2014;69(4):463–471.
- Osteikoetxea X, Balogh A, Szabó-Taylor K, Németh A, Szabó TG, Pálóczi K, Sódar B, Kittel Á, György B, Pállinger É, et al. Improved characterization of EV preparations based on protein to lipid ratio and lipid properties. PLoS ONE 2015;10(3):1–16.
- Pocsfalvi G, Stanly C, Vilasi A, Fiume I, Capasso G, Turiák L, Buzas EI, Vékey K. Mass spectrometry of extracellular vesicles. Mass Spectrometry Reviews 2016;35(1):3–21.
- van Blitterswijk WJ, de Veer G, Krol JH, Emmelot P. Comparative lipid analysis of purified plasma membranes and shed extracellular membrane vesicles from normal murine thymocytes and leukemic GRSL cells. BBA - Biomembranes 1982;688(2):495–504.
- Jia S, Zocco D, Samuels ML, Chou MF, Chammas R, Skog J, Zarovni N, Momen-Heravi F, Kuo WP. Emerging technologies in extracellular vesicle-based molecular diagnostics. Expert Rev Mol Diagn 2014;14(3):307–21.
- Koppers-Lalic D, Hackenberg M, Menezes R, Misovic B, Wachalska M, Geldof A, Zini N, Reijke T, Wurdinger T, Vis A, et al. Noninvasive prostate cancer detection by measuring miRNA variants (isomiRs) in urine extracellular vesicles. Oncotarget 2016;7(16):22566–22578.
- Lazaro-Ibanez E, Sanz-Garcia A, Visakorpi T, Escobedo-Lucea C, Siljander P, Ayuso-Sacido A, Yliperttula M. Different gDNA content in the subpopulations of prostate cancer extracellular vesicles: apoptotic bodies, microvesicles, and exosomes. Prostate 2014;74(14):1379–90.
- Harshman SW, Canella A, Ciarlariello PD, Agarwal K, Branson OE, Rocci A, Cordero H, Phelps MA, Hade EM, Dubovsky JA, et al. Proteomic characterization of circulating extracellular vesicles identifies novel serum myeloma associated markers. Journal of Proteomics 2016;136:89–98.
- Eldh M, Olofsson Bagge R, Lässer C, Svanvik J, Sjöstrand M, Mattsson J, Lindnér P, Choi DS, Gho YS, Lötvall J. MicroRNA in exosomes isolated directly from the liver circulation in patients with metastatic uveal melanoma. BMC Cancer 2014;14(962):1–10.
- Figliolini F, Cantaluppi V, De Lena M, Beltramo S, Romagnoli R, Salizzoni M, Melzi R, Nano R, Piemonti L, Tetta C, et al. Isolation, characterization and potential role in beta cell-endothelium cross-talk of extracellular vesicles released from human pancreatic islets. PLoS ONE 2014;9(7):1–14.
- Svennerholm K, Rodsand P, Hellman U, Lundholm M, Waldenström A, Biber B, Ronquist G, Haney M. Myocardial ischemic preconditioning in a porcine model leads to rapid changes in cardiac extracellular vesicle messenger RNA content. IJC Heart and Vasculature 2015;8:62–67.
- Shao H, Chung J, Balaj L, Charest A, Bigner DD, Carter BS, Hochberg FH, Breakefield XO, Weissleder R, Lee H. Protein typing of circulating microvesicles allows real-time monitoring of glioblastoma therapy. Nat Med 2012;18(12):1835–40.
- Yoshioka Y, Kosaka N, Konishi Y, Ohta H, Okamoto H, Sonoda H, Nonaka R, Yamamoto H, Ishii H, Mori M, et al. Ultra-sensitive liquid biopsy of circulating extracellular vesicles using ExoScreen. Nature communications 2014;5:3591.
- Grasso L, Wyss R, Weidenauer L, Thampi A, Demurtas D, Prudent M, Lion N, Vogel H. Molecular screening of cancer-derived exosomes by surface plasmon resonance spectroscopy. Anal Bioanal Chem 2015;407(18):5425–32.
- Im H, Shao HL, Park YI, Peterson VM, Castro CM, Weissleder R, Lee H. Label-free detection and molecular profiling of exosomes with a nano-plasmonic sensor. Nature Biotechnology 2014;32(5):490–U219.
- Rupert DLM, Lässer C, Eldh M, Block S, Zhdanov VP, Lotvall JO, Bally M, Höök F. Determination of exosome concentration in solution using surface plasmon resonance spectroscopy. Analytical Chemistry 2014;86(12):5929–5936.
- Zhu L, Wang K, Cui J, Liu H, Bu X, Ma H, Wang W, Gong H, Lausted C, Hood L, et al. Label-free quantitative detection of tumor-derived exosomes through surface plasmon resonance imaging. Analytical Chemistry 2014;86(17):8857–8864.
- Schasfoort RBM, Tudos AJ. Handbook of surface plasmon resonance. Cambridge :: Royal Society of Chemistry; 2008.
- Zarovni N, Corrado A, Guazzi P, Zocco D, Lari E, Radano G, Muhhina J, Fondelli C, Gavrilova J, Chiesi A. Integrated isolation and quantitative analysis of exosome shuttled proteins and nucleic acids using immunocapture approaches. Methods 2015;87:46–58.
- Jorgensen M, Baek R, Pedersen S, Sondergaard EK, Kristensen SR, Varming K. Extracellular Vesicle (EV) Array: microarray capturing of exosomes and other extracellular vesicles for multiplexed phenotyping. J Extracell Vesicles 2013;2:1–9.
- Jorgensen MM, Baek R, Varming K. Potentials and capabilities of the Extracellular Vesicle (EV) Array. J Extracell Vesicles 2015;4:26048.
- Duijvesz D, Versluis CYL, Van Der Fels CAM, Vredenbregt-Van Den Berg MS, Leivo J, Peltola MT, Bangma CH, Pettersson KSI, Jenster G. Immuno-based detection of extracellular vesicles in urine as diagnostic marker for prostate cancer. International Journal of Cancer 2016;137(12):2869–2878.
- Vaidyanathan R, Naghibosadat M, Rauf S, Korbie D, Carrascosa LG, Shiddiky MJ, Trau M. Detecting exosomes specifically: a multiplexed device based on alternating current electrohydrodynamic induced nanoshearing. Anal Chem 2014;86(22):11125–32.
- van der Pol E, Coumans FA, Grootemaat AE, Gardiner C, Sargent IL, Harrison P, Sturk A, van Leeuwen TG, Nieuwland R. Particle size distribution of exosomes and microvesicles determined by transmission electron microscopy, flow cytometry, nanoparticle tracking analysis, and resistive pulse sensing. J Thromb Haemost 2014;12(7):1182–92.
- He M, Crow J, Roth M, Zeng Y, Godwin AK. Integrated immunoisolation and protein analysis of circulating exosomes using microfluidic technology. Lab Chip 2014;14(19):3773–80.
- Boing AN, van der Pol E, Grootemaat AE, Coumans FA, Sturk A, Nieuwland R. Single-step isolation of extracellular vesicles by size-exclusion chromatography. J Extracell Vesicles 2014;3(23430):1–11.
- Osteikoetxea X, Sodar B, Nemeth A, Szabo-Taylor K, Paloczi K, Vukman KV, Tamasi V, Balogh A, Kittel A, Pallinger E, et al. Differential detergent sensitivity of extracellular vesicle subpopulations. Org Biomol Chem 2015;13(38):9775–82.
- Andrews AM, Lutton EM, Merkel SF, Razmpour R, Ramirez SH. Mechanical injury induces brain endothelial-derived microvesicle release: Implications for cerebral vascular injury during traumatic brain injury. Frontiers in Cellular Neuroscience 2016;10(43):1–13.
- Atienzar-Aroca S, Flores-Bellver M, Serrano-Heras G, Martinez-Gil N, Barcia JM, Aparicio S, Perez-Cremades D, Garcia-Verdugo JM, Diaz-Llopis M, Romero FJ, et al. Oxidative stress in retinal pigment epithelium cells increases exosome secretion and promotes angiogenesis in endothelial cells. Journal of Cellular and Molecular Medicine 2016;20(8):1457–1466.
- Ban JJ, Lee M, Im W, Kim M. Low pH increases the yield of exosome isolation. Biochemical and Biophysical Research Communications 2015;461(1):76–79.
- Burnett LA, Light MM, Mehrotra P, Nowak RA. Stimulation of GPR30 increases release of EMMPRIN-containing microvesicles in human uterine epithelial cells. Journal of Clinical Endocrinology and Metabolism 2012;97(12):4613–4622.
- Burns G, Brooks K, Wildung M, Navakanitworakul R, Christenson LK, Spencer TE. Extracellular vesicles in luminal fluid of the ovine uterus. PLoS ONE 2014;9(3):1–11.
- Carver KA, Yang D. N-acetylcysteine amide protects against oxidative stress–induced microparticle release from human retinal pigment epithelial cells. Investigative Ophthalmology and Visual Science 2016;57(2):360–371.
- Chaudhary SC, Kuzynski M, Bottini M, Beniash E, Dokland T, Mobley CG, Yadav MC, Poliard A, Kellerman O, Millán JL, et al. Phosphate induces formation of matrix vesicles during odontoblast-initiated mineralization in vitro. Matrix Biology 2015;52-54:284–300.
- Giricz Z, Varga ZV, Baranyai T, Sipos P, Pálóczi K, Kittel T, Buzás EI, Ferdinandy P. Cardioprotection by remote ischemic preconditioning of the rat heart is mediated by extracellular vesicles. Journal of Molecular and Cellular Cardiology 2014;68:75–78.
- Hong SW, Choi EB, Min TK, Kim JH, Kim MH, Jeon SG, Lee BJ, Gho YS, Jee YK, Pyun BY, et al. An important role of α-hemolysin in extracellular vesicles on the development of atopic dermatitis induced by Staphylococcus aureus. PLoS ONE 2014;9(7):1–10.
- Hubert A, Subra C, Jenabian MA, Labrecque PFT, Tremblay C, Laffont B, Provost P, Routy JP, Gilbert C. Elevated abundance, size, and MicroRNA content of plasma extracellular vesicles in viremic HIV-1+ patients: Correlations with known markers of disease progression. Journal of Acquired Immune Deficiency Syndromes 2015;70(3):219–227.
- González DA, Egido P, Balcarcel NB, Hattab C, Barbieri van Haaster MM, Pelletier J, Sévigny J, Ostuni MA. Rat submandibular glands secrete nanovesicles with NTPDase and 5′-nucleotidase activities. Purinergic Signalling 2015;11(1):107–116.
- Kranendonk MEG, Visseren FLJ, Van Balkom BWM, Nolte-'T Hoen ENM, Van Herwaarden JA, De Jager W, Schipper HS, Brenkman AB, Verhaar MC, Wauben MHM, et al. Human adipocyte extracellular vesicles in reciprocal signaling between adipocytes and macrophages. Obesity 2014;22(5):1296–1308.
- Javeed N, Sagar G, Dutta SK, Smyrk TC, Lau JS, Bhattacharya S, Truty M, Petersen GM, Kaufman RJ, Chari ST, et al. Pancreatic cancer-derived exosomes cause paraneoplastic β-cell dysfunction. Clinical Cancer Research 2015;21(7):1722–1733.
- Klein-Scory S, Kübler S, Diehl H, Eilert-Micus C, Reinacher-Schick A, Stühler K, Warscheid B, Meyer HE, Schmiegel W, Schwarte-Waldhoff I. Immunoscreening of the extracellular proteome of colorectal cancer cells. BMC Cancer 2010;10(70):1–19.
- Hung WT, Hong X, Christenson LK, McGinnis LK. Extracellular vesicles from bovine follicular fluid support cumulus expansion. Biology of Reproduction 2015;93(5:117):1–9.
- Lopes-Rodrigues V, Di Luca A, Sousa D, Seca H, Meleady P, Henry M, Lima RT, O'Connor R, Vasconcelos MH. Multidrug resistant tumour cells shed more microvesicle-like EVs and less exosomes than their drug-sensitive counterpart cells. Biochimica et Biophysica Acta - General Subjects 2016;1860(3):618–627.
- Li H, Han L, Yang Z, Huang W, Zhang X, Gu Y, Li Y, Liu X, Zhou L, Hu J, et al. Differential Proteomic Analysis of Syncytiotrophoblast Extracellular Vesicles from Early-Onset Severe Preeclampsia, using 8-Plex iTRAQ Labeling Coupled with 2D Nano LC-MS/MS. Cellular Physiology and Biochemistry 2015;36(3):1116–1130.
- Marie G, Dunning CJ, Gaspar R, Grey C, Brundin P, Sparr E, Linse S. Acceleration of α-synuclein aggregation by exosomes. Journal of Biological Chemistry 2015;290(5):2969–2982.
- Momen-Heravi F, Saha B, Kodys K, Catalano D, Satishchandran A, Szabo G. Increased number of circulating exosomes and their microRNA cargos are potential novel biomarkers in alcoholic hepatitis. Journal of Translational Medicine 2015;13(261):1–13.
- Ohshima K, Kanto K, Hatakeyama K, Ide T, Wakabayashi-Nakao K, Watanabe Y, Sakura N, Terashima M, Yamaguchi K, Mochizuki T. Exosome-mediated extracellular release of polyadenylate-binding protein 1 in human metastatic duodenal cancer cells. Proteomics 2014;14(20):2297–2306.
- Pienimaeki-Roemer A, Kuhlmann K, Böttcher A, Konovalova T, Black A, Orsõ E, Liebisch G, Ahrens M, Eisenacher M, Meyer HE, et al. Lipidomic and proteomic characterization of platelet extracellular vesicle subfractions from senescent platelets. Transfusion 2015;55(3):507–521.
- Malik ZA, Kott KS, Poe AJ, Kuo T, Chen L, Ferrara KW, Knowlton AA. Cardiac myocyte exosomes: Stability, HSP60, and proteomics. American Journal of Physiology - Heart and Circulatory Physiology 2013;304(7):H954–H965.
- Ramos TL, Sánchez-Abarca LI, Muntión S, Preciado S, Puig N, López-Ruano G, Hernández-Hernández Á, Redondo A, Ortega R, Rodríguez C, et al. MSC surface markers (CD44, CD73, and CD90) can identify human MSC-derived extracellular vesicles by conventional flow cytometry. Cell Communication and Signaling 2016;14(2):1–14.
- Ramos TL, Sánchez-Abarca LI, López-Ruano G, Muntión S, Preciado S, Hernández-Ruano M, Rosado B, de las Heras N, Chillón MC, Hernández-Hernández T, et al. Do endothelial cells belong to the primitive stem leukemic clone in CML? Role of extracellular vesicles. Leukemia Research 2015;39(8):921–924.
- Schiller M, Heyder P, Ziegler S, Niessen A, Claßen L, Lauffer A, Lorenz HM. During apoptosis HMGB1 is translocated into apoptotic cell-derived membraneous vesicles. Autoimmunity 2013;46(5):342–346.
- Sáenz-Cuesta M, Arbelaiz A, Oregi A, Irizar H, Osorio-Querejeta I, Muñoz-Culla M, Banales JM, Falcón-Pérez JM, Olascoaga J, Otaegui D. Methods for extracellular vesicles isolation in a hospital setting. Frontiers in Immunology 2015;6:1–12.
- Saari H, Lázaro-Ibáñez E, Viitala T, Vuorimaa-Laukkanen E, Siljander P, Yliperttula M. Microvesicle- and exosome-mediated drug delivery enhances the cytotoxicity of Paclitaxel in autologous prostate cancer cells. Journal of Controlled Release 2015;220:727–737.
- da Silva Nardi F, Slowik R, Michelon T, dos Santos Manvailer LF, Wagner B, Neumann J, Horn P, da Graça Bicalho M, Rebmann V. High Amounts of Total and Extracellular Vesicle-Derived Soluble HLA-G are Associated with HLA-G 14-bp Deletion Variant in Women with Embryo Implantation Failure. American Journal of Reproductive Immunology 2016;75(6):661–671.
- Benito-Martin A, Ucero AC, Zubiri I, Posada-Ayala M, Fernandez-Fernandez B, Cannata-Ortiz P, Sanchez-Nino MD, Ruiz-Ortega M, Egido J, Alvarez-Llamas G, et al. Osteoprotegerin in Exosome-Like Vesicles from Human Cultured Tubular Cells and Urine. PLoS ONE 2013;8(8):1–13.
- Dubois L, Stridsberg M, Kharaziha P, Chioureas D, Meersman N, Panaretakis T, Ronquist KG. Malignant cell-derived extracellular vesicles express different chromogranin epitopes compared to prostasomes. Prostate 2015;75(10):1063–1073.
- Franquesa M, Hoogduijn MJ, Ripoll E, Luk F, Salih M, Betjes MGH, Torras J, Baan CC, Grinyó JM, Merino AM. Update on controls for isolation and quantification methodology of extracellular vesicles derived from adipose tissue mesenchymal stem cells. Frontiers in Immunology 2014;5(525):1–10.
- Gehrmann U, Qazi KR, Johansson C, Hultenby K, Karlsson M, Lundeberg L, Gabrielsson S, Scheynius A. Nanovesicles from malassezia sympodialis and host exosomes induce cytokine responses - novel mechanisms for host-microbe interactions in atopic eczema. PLoS ONE 2011;6(7):1–10.
- Grange C, Tapparo M, Tritta S, Deregibus MC, Battaglia A, Gontero P, Frea B, Camussi G. Role of HLA-G and extracellular vesicles in renal cancer stem cell-induced inhibition of dendritic cell differentiation. BMC Cancer 2015;15(1009):1–11.
- Guescini M, Leo G, Genedani S, Carone C, Pederzoli F, Ciruela F, Guidolin D, Stocchi V, Mantuano M, Borroto-Escuela DO, et al. Microvesicle and tunneling nanotube mediated intercellular transfer of g-protein coupled receptors in cell cultures. Experimental Cell Research 2012;318(5):603–613.
- Hong SW, Kim MR, Lee EY, Kim JH, Kim YS, Jeon SG, Yang JM, Lee BJ, Pyun BY, Gho YS, et al. Extracellular vesicles derived from Staphylococcus aureus induce atopic dermatitis-like skin inflammation. Allergy: European Journal of Allergy and Clinical Immunology 2011;66(3):351–359.
- Kobayashi M, Salomon C, Tapia J, Illanes SE, Mitchell MD, Rice GE. Ovarian cancer cell invasiveness is associated with discordant exosomal sequestration of Let-7 miRNA and miR-200. Journal of Translational Medicine 2014;12(1):1–12.
- Nardi FDS, Michelon TF, Neumann J, Manvailer LFS, Wagner B, Horn PA, Bicalho MDG, Rebmann V. High levels of circulating extracellular vesicles with altered expression and function during pregnancy. Immunobiology 2016;221(7):753–760.
- Pisetsky DS. The expression of HMGB1 on microparticles released during cell activation and cell death in vitro and in vivo. Molecular Medicine 2014;20(1):158–163.
- Properzi F, Logozzi M, Abdel-Haq H, Federici C, Lugini L, Azzarito T, Cristofaro I, di Sevo D, Ferroni E, Cardone F, et al. Detection of exosomal prions in blood by immunochemistry techniques. J Gen Virol 2015;96(Pt 7):1969–74.
- Rivera J, Cordero RJB, Nakouzi AS, Frases S, Nicola A, Casadevall A. Bacillus anthracis produces membrane-derived vesicles containing biologically active toxins. Proceedings of the National Academy of Sciences of the United States of America 2010;107(44):19002–19007.
- Roca E, Lacroix R, Judicone C, Laroumagne S, Robert S, Cointe S, Muller A, Kaspi E, Roll P, Brisson AR, et al. Detection of EpCAM-positive microparticles in pleural fluid: A new approach to mini-invasively identify patients with malignant pleural effusions. Oncotarget 2016;7(3):3357–3366.
- Spencer DM, Mobarrez F, Wallén H, Pisetsky DS. The Expression of HMGB1 on Microparticles from Jurkat and HL-60 Cells Undergoing Apoptosis in vitro. Scandinavian Journal of Immunology 2014;80(2):101–110.
- Vallejo MC, Matsuo AL, Ganiko L, Medeiros LCS, Miranda K, Silva LS, Freymüller-Haapalainen E, Sinigaglia-Coimbra R, Almeida IC, Puccia R. The pathogenic fungus Paracoccidioides brasiliensis exports extracellular vesicles containing highly Immunogenic α-galactosyl epitopes. Eukaryotic Cell 2011;10(3):343–351.
- Vargas A, Zhou S, Et́hier-Chiasson M, Flipo D, Lafond J, Gilbert C, Barbeau B. Syncytin proteins incorporated in placenta exosomes are important for cell uptake and show variation in abundance in serum exosomes from patients with preeclampsia. FASEB Journal 2014;28(8):3703–3719.
- Zlotogorski-Hurvitz A, Dayan D, Chaushu G, Korvala J, Salo T, Sormunen R, Vered M. Human Saliva-Derived Exosomes: Comparing Methods of Isolation. Journal of Histochemistry and Cytochemistry 2015;63(3):181–189.
- Chaparro A, Gaedechens D, Ramírez V, Zuñiga E, Kusanovic JP, Inostroza C, Varas-Godoy M, Silva K, Salomon C, Rice G, et al. Placental biomarkers and angiogenic factors in oral fluids of patients with preeclampsia. Prenatal Diagnosis 2016;36(5):476–482.
- Moon PG, Lee JE, Cho YE, Lee SJ, Jung JH, Chae YS, Bae HI, Kim YB, Kim IS, Park HY, et al. Identification of developmental endothelial locus-1 on circulating extracellular vesicles as a novel biomarker for early breast cancer detection. Clinical Cancer Research 2016;22(7):1757–1766.
- Tan KH, Tan SS, Sze SK, Lee WKR, Ng MJ, Lim SK. Plasma biomarker discovery in preeclampsia using a novel differential isolation technology for circulating extracellular vesicles. American Journal of Obstetrics and Gynecology 2014;211(4):380.e1–380.e13.