Abstract
Immune thrombocytopenia (ITP) is an autoimmune bleeding disorder in which autoantibodies and/or autoreactive T cells destroy platelets and megakaryocytes in the spleen and bone marrow, respectively. Thrombopoietin receptor agonists (TPO-RA e.g. Romiplostim and Eltrombopag) have made a substantial contribution to the treatment of patients with ITP, which are refractory to first-line treatments and approximately 30% demonstrate sustained elevated platelet counts after drug tapering. How TPO-RA induce these sustained responses is not known. We analyzed the efficacy of a murine TPO-RA in a well-established murine model of active ITP. Treatment with TPO-RA (10 ug/kg, based on pilot dose escalation experiments) significantly raised the platelet counts in ITP-mice. Immunomodulation was assessed by measuring serum IgG anti-platelet antibody levels; TPO-RA-treated mice had significantly reduced IgG anti-platelet antibodies despite the increasing platelet counts. These results suggest that TPO-RA is not only an efficacious therapy but also reduces anti-platelet humoral immunity in ITP.
Introduction
Immune thrombocytopenia (ITP) is an autoimmune bleeding disorder where anti-platelet antibodies and/or T cells specifically target platelet/megakaryocyte antigens leading to peripheral platelet destruction and decreased bone marrow platelet production[Citation1]. This results in thrombocytopenia and clinical symptoms such as bruising and bleeding. The pathogenic mechanisms responsible for ITP are complex and multifactorial[Citation2]. Several treatment options for ITP are available such as first line-treatments like corticosteroids, intravenous immunoglobulin (IVIg) or anti-D and are generally aimed at decreasing autoantibody‐mediated platelet clearance[Citation1].Second-line therapies include immunosuppressive agents such as Rituximab (anti-CD20 antibody) and splenectomy. In case of failure of Rituximab-treatment or splenectomy, third-line treatments can be initiated and these include Thrombopoietin (TPO)-receptor agonists (RA) such has Romiplostim or Eltrombopag. These treatments induce platelet production by activating TPO receptors on megakaryocytes [Citation3,Citation4]. Additionally, Romiplostim was shown to improve Treg activity in patients with chronic ITP and to increase circulating transforming growth factor-β1 (TGF-β1) levels, which correlated with platelet count increments[Citation5]. Furthermore, in a prospective study of 21 corticosteroid-resistant/relapsed chronic ITP patients who received Eltrombopag therapy, Eltrombopag was shown to shift the monocyte-FcγR balance toward the inhibitory FcγRIIb[Citation6]. This was associated with decreased monocyte/macrophage-mediated phagocytosis in vitro, as well as with increased plasma levels of TGF-β1 in the ITP patients responding to Eltrombopag[Citation6]. Remarkably, it appears that approximately 30% of TPO-RA-treated patients remain in remission even after 24 weeks off TPO treatment[Citation7].
To gain more insights into this sustained response, we used a well-established murine model of active ITP, which demonstrates both antibody- and T cell-mediated thrombocytopenia [Citation8] and investigated murine TPO-RA efficacy with regards to its effects on platelet counts and anti-platelet antibody production. We show that murine TPO-RA significantly raises platelet counts not only in naïve mice but also in the mice suffering from ITP. Remarkably, the TPO-RA treatment also significantly lowered anti-platelet antibody levels while the platelet counts were being rescued possibly suggesting immunomodulation was taking place.
Methods
An initial pilot dose escalation study was performed by infusing naïve WT BALB/c mice with a single subcutaneous (sc) bolus of murine TPO-RA (AMP4, Amgen; 0.1, 1 and 10 ug/kg). We utilized our in vivo murine model of active ITP, which harbors both antibody- and T cell-mediated thrombocytopenia as described previously[Citation8]. In this model, platelet glycoprotein (GP) IIIa (CD61) knockout (KO) mice were immunized with CD61+ platelets and ITP was initiated by transfer of their splenocytes (4 x 104) into sub-lethally irradiated mice with severe combined immunodeficiency (SCID). The SCID mice were treated with TPO-RA (10ug/kg) weekly after transfer and platelet counts and serum anti-platelet antibodies were measured weekly. Blood was collected weekly via the saphenous vein into a microvette tube containing PBS:citrate-phosphate-dextrose-adenine (CPDA) solution and platelet counts were counted using a Beckman Coulter Counter-LH750 hematology analyzer (Brea, CA). Serum was produced by bleeding the mice weekly into preservative-free microvette tubes. Serum anti-platelet antibody levels were evaluated by titrating ITP mouse serum onto WT platelets followed by binding with a fluorescein-labelled goat anti-Mouse IgG and analyzed by flow cytometry. In selected mice, femurs were removed, cleaned of tissue and their ends were cut off and placed in fixative (B_ Fixative) for 12 hours. Decalcification was achieved with 10% nitric acid followed by embedding in wax and staining with hematoxylin and eosin (H&E).
Results
In a pilot dose escalation study, compared with a small dose of murine TPO-RA (0.1 ug/kg, )), a 1 ug/kg or 10 ug/kg infusion into naïve WT mice significantly increased platelet counts at 72 hours post-infusion ()). Compared with naïve mice ()), the increased platelet counts also correlated with increased megakaryocyte (MK) numbers in the bone marrow ()). Based on the maximum increase in platelet counts, a dose of 10 ug/kg of murine TPO-RA was used to treat SCID mice suffering from ITP. When naïve (non-immune) CD61-KO splenocytes were transferred into irradiated SCID mice, platelet counts normalized towards pre-transfer control levels after 2 weeks ()). In contrast, when immune CD61-KO splenocytes were transferred into irradiated SCID mice, the thrombocytopenia persisted from 184 ± 130 (SD) and 182 ± 80 after 2 and 3 weeks, respectively ()). When the ITP mice were treated with weekly injections of TPO-RA (10 ug/kg), platelet counts (x 109/L) increased to 376 ± 105 (SD) and 678 ± 122 after 2 and 3 weeks, respectively ()). Compared with non-treated ITP mice, serum IgG anti-platelet antibody production (percentage gated anti-platelet antibody positive cells) in the TPO-RA treated mice was significantly reduced after 1 and 2 weeks despite the increasing platelet counts ()). Similarly, TPO-RA treatment reduced platelet-associated IgG ()).
Figure 1. Murine TPO-RA significantly increases platelet counts in naive mice and in mice suffering from ITP. Naïve WT BALB/c mice were administered (A) 0.1 ug/kg, (B) 1 ug/kg or (C) 10 ug/kg murine TPO-RA subcutaneously (sc) and platelet counts were measured on days 0, 3, 7 and 14. Thrombocytopenia was assessed in irradiated SCID mice transferred with 4 × 104 splenocytes from naïve CD61-KO mice (D) or with splenocytes from CD61-KO mice immunized against WT BALB/c platelets (E) or with splenocytes from CD61-KO mice immunized against WT BALB/c platelets treated with 10 ug/kg TPO-RA sc (F). Platelet counts were evaluated on days 0, 7, 14 and 21 after splenocyte transfer. Representative bone marrow histology (H&E) of (G) a naïve mouse and (H) a TPO-RA treated mouse (10 ug/kg) at 72 hours post treatment. White solid circles in each panel mark megakaryocytes. Statistical comparisons were made using one-way ANOVA with Dunnett’s multiple comparison test comparing to day zero (panel A-C) or day seven (panel D-F). Only statistical significant comparisons of interest are shown; *: P < .05, **: P < .01, ***: P < .001, ****: P < .0001. Error bars represent standard deviations (SD).
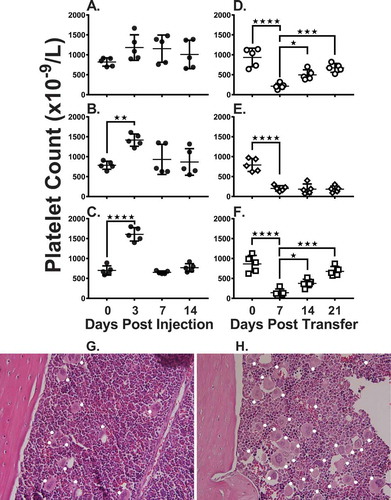
Figure 2. (A) Serum IgG anti-platelet antibody levels were assessed in irradiated SCID mice transferred with either 4 × 104 splenocytes from naïve CD61-KO mice (○), with splenocytes from CD61-KO mice immunized against WT BALB/c platelets (◊) or with splenocytes from CD61-KO mice immunized against WT BALB/c platelets treated with 10 ug/kg TPO-RA sc (□) on days 0, 7 and 14 (n = 5 mice per group). Sera were titrated onto WT platelets, stained with a FITC goat anti-mouse IgG antibody and the percent positive platelets are shown at a serum dilution of 1/100. (B) Representative flow cytometric histogram analysis of platelet-associated IgG (PAIgG) bound to platelets from a SCID mouse suffering from ITP at day 21 post transfer (thick line) or a SCID mouse treated with TPO-RA at day 21 post transfer (thin line). Platelets were produced from CPDA PRP, washed and stained with a FITC-conjugated goat anti-mouse IgG antibody for 30 minutes and acquired on the flow cytometer. Statistical comparisons were made using two-way ANOVA with Tukey’s multiple comparison test. The statistical comparison immune CD61-KO SC vs immune CD61-KO SC + TPO-RA is shown. In all panels, only statistical significant comparisons of interest are shown; **: P < .01, ****: P < .0001. Error bars represent SD.
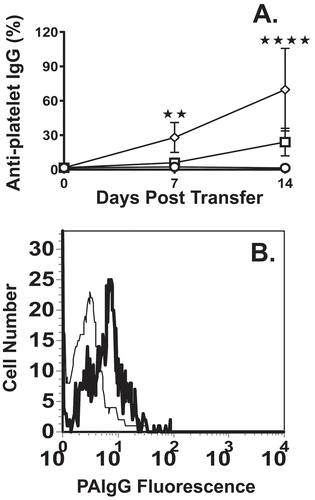
Discussion
TPO-RA effectively raised the platelet counts in a murine model of active ITP and inhibited serum IgG anti-platelet antibody production. These are the first results that we know of that have analyzed the murine equivalent of the human peptidobody, NPlate (Romiplostim, Amgen). The efficacious nature of this novel TPO-RA in murine ITP ()) is an advantage as it will allow for detailed studies on its ability to affect immunity in ITP. Related to this, while the platelet counts were normalizing from the TPO-RA therapy, the production of serum anti-platelet IgG antibodies was significantly reduced ()). This may be due to the nascent platelets acting as an immunological sink and adsorbing the anti-platelet antibodies, however, we could not detect IgG anti-platelet antibodies on the surface of the new platelets ()). Alternatively, the transplanted splenocytes may have reduced survival, which could account for the lowered antibody response, however, our previous results argue against this possibility. First, we have previously demonstrated that even when the transferred SCID mice are taken to 28 weeks post transfer, the strongest anti-platelet IgG antibody titers are observed [Citation8,Citation9]. This suggests that within the time frame of the current experiments, there appears to be no exhaustion of the transferred spleen cell’s ability to generate antibodies. Second, we have transferred splenocytes from the transferred SCID mice into naïve SCID mice at day 28 post-transfer and the splenocytes were able to transfer ITP suggesting they have the ability to mediate disease (unpublished data).
Taken together, our data suggest that the TPO-RA affects the production of anti-platelet antibodies but how this occurs is still unknown. One possibility may be related to the recent observation that TPO-stimulated MKs can process and present self-antigens and enhance platelet-specific CD8 + T cell reactivity in vitro and in vivo[Citation10]. Perhaps TPO-RA may affect immunity by altering antigen presentation and ultimately down-regulate platelet-specific immunity. In summary, these data support the notion that murine TPO-RA is not only an efficacious therapy for murine ITP but may also reduce anti-platelet immunity.
Declaration of interest statement
The authors report no potential conflicts of interest.
Author’s contributions
R.K. and R.A. designed and performed experiments, collected, analyzed, interpreted data, wrote and edited the paper; E.R.S performed experiments and collected data; J.M.R. collected and interpreted data; J.W.S. provided financial resources and edited the manuscript.
Acknowledgements
We thank Amgen for graciously supplying the murine TPO-RA (AMP4).
Additional information
Funding
References
- Zufferey A, Kapur R, Semple JW. Pathogenesis and therapeutic mechanisms in immune thrombocytopenia (ITP). J Clin Med 2017;6(2):16. doi:10.3390/jcm6020016
- McKenzie CG, Guo L, Freedman J, Semple JW. Cellular immune dysfunction in immune thrombocytopenia (ITP). Br J Haematol 2013;163(1):10–23. doi:10.1111/bjh.12480
- Erickson-Miller CL, DeLorme E, Tian SS, Hopson CB, Stark K, Giampa L, Valoret EI, Duffy KJ, Luengo JL, Rosen J, et al. Discovery and characterization of a selective, nonpeptidyl thrombopoietin receptor agonist. Exp Hematol 2005;33(1):85–93. doi:10.1016/j.exphem.2004.09.006
- Cohn CS, Bussel JB. Romiplostim: a second-generation thrombopoietin agonist. Drugs Today (Barc) 2009;45(3):175–188. doi:10.1358/dot.2009.45.3.1343793
- Bao W, Bussel JB, Heck S, He W, Karpoff M, Boulad N, Yazdanbakhsh K. Improved regulatory T-cell activity in patients with chronic immune thrombocytopenia treated with thrombopoietic agents. Blood 2010;116(22):4639–4645. doi:10.1182/blood-2010-04-281717
- Liu XG, Liu S, Feng Q, Liu X-N, Li G-S, Sheng Z, Chen P, Liu Y, Wei Y, Dong X-Y, et al. Thrombopoietin receptor agonists shift the balance of Fcgamma receptors toward inhibitory receptor IIb on monocytes in ITP. Blood 2016;128(6):852–861. doi:10.1182/blood-2016-01-690727
- Newland A, Godeau B, Priego V, Viallard J-F, López Fernández MF, Orejudos A, Eisen M. Remission and platelet responses with romiplostim in primary immune thrombocytopenia: final results from a phase 2 study. Br J Haematol 2016;172(2):262–273. doi:10.1111/bjh.13827
- Chow L, Aslam R, Speck ER, Kim M, Cridland N, Webster ML, Chen P, Sahib K, Ni H, Lazarus AH, et al. A murine model of severe immune thrombocytopenia is induced by antibody- and CD8+ T cell-mediated responses that are differentially sensitive to therapy. Blood 2010;115(6):1247–1253. doi:10.1182/blood-2009-09-244772
- Aslam R, Hu Y, Gebremeskel S, Segel GB, Speck ER, Guo L, Kim M, Ni H, Freedman J, Semple JW. Thymic Retention of CD4+CD25+FoxP3+ T regulatory cells is associated with their peripheral deficiency and thrombocytopenia in a murine model of immune thrombocytopenia (ITP). Blood 2012;120(10):2127–2132. doi:10.1182/blood-2012-02-413526
- Zufferey A, Speck ER, Aslam R, Aslam R, Guo L, McVey MJ, Kim M, Kapur R, Boilard E, Italiano JE, et al. Mature murine megakaryocytes present antigen-MHC class I molecules to T cells and transfer them to platelets. Blood Adv 2017;1(20):1773–1785. doi:10.1182/bloodadvances.2017007021