Abstract
Recent years have witnessed the introduction of ex vivo expanded dermal fibroblasts for several cell therapy and tissue-engineering applications, including the treatment of facial scars and burns, representing a promising cell type for regenerative medicine. We tested different in-house produced human platelet lysate (HPL) solutions against fetal bovine serum as supplements for in vitro fibroblast expansion by comparing cell yield, molecular marker expression, extracellular matrix (ECM) generation, genomic stability and global gene expression. Our in-house produced HPL supported fibroblast growth at levels similar to those for FBS and commercial HPL products and was superior to AB human serum. Cells grown in HPL maintained a fibroblast phenotype (VIM+, CD44+, CD13+, CD90+), ECM generation capacity (FN+, COL1+) and a normal karyotype, although gene expression profiling revealed changes related to cell metabolism, adhesion and cellular senescence. The HPL manufacturing process was validated within a GMP compliant system and the solution was stable at −80ºC and −20ºC for 2 years. Dermal fibroblasts expanded in vitro with HPL maintain a normal karyotype and expression of fibroblast markers, with only minor changes in their global gene expression profile. Our in-house produced GMP-HPL is an efficient, safe and economical cell culture supplement that can help increase the healthcare activity of blood transfusion centers through the re-use of transfusional plasma and platelets approaching their expiration date. Currently, our HPL solution is approved by the Spanish Agency of Medicines and Medical Devices and is being used in the manufacture of cell therapy products.Abbreviations: AB plasma: plasma group AB; ABHS: AB Human Serum; ABHS+GF: AB Human Serum supplemented with growth factors; ANOVA: Analysis of variance; ATMPs: Advanced Therapies for Medicinal Products; CPE: cytopathic effect; DEGs: Differentially expressed genes; DMEM: Dulbecco’s modified Eagle’s Medium; ECM: Extracellular matrix; ELISA: enzyme-linked immunosorbent assay; FBS: Fetal bovine serum; FDR: False discovery rate; FGF: Fibroblast growth factor; GMP: Good manufacturing practice; HPL: Human platelet lysate; HPL-CM: commercial human platelet lysate; MSCs: mesenchymal stem cells; NEAA: non-essential amino acids; P/S: penicillin/streptomycin; PBS: phosphate buffered saline; PC: leukodepleted platelet concentrate; PCR: polymerase chain reaction; PDGF: Platelet-derived growth factor; PDGFRA: Platelet-derived growth factor receptor alpha; qPCR: quantitative polymerase chain reaction; RNA: Ribonucleic acid; RT: Room temperature; TAC: Transcriptome analysis console; TGF-β: Transforming growth factor beta
Introduction
Fibroblasts are cells of mesenchymal origin with key roles in both pathological and regenerative responses [Citation1]. They are considered tissue filler cells based on their capacity to synthesize extracellular matrix in connective tissues, providing a physical framework for other cells [Citation2]. Fibroblasts have recently come under the spotlight as therapeutics in regenerative medicine owing to their regenerative capacity together with their multilineage differentiation potential and immunosuppressive properties [Citation3–5].
Although mesenchymal stem cells (MSCs) are commonly regarded as the ideal source for the development of cell-based therapeutics, they are associated with several limitations and fibroblasts might be a more appropriate choice for some cell therapies. The most commonly used MSCs source, the bone marrow, provides relatively little starting material for cellular expansion and requires invasive extraction procedures, whereas fibroblasts are easily harvested in large numbers from various biological waste products (e.g., foreskin, leftover skin from abdominoplasties and mammoplasties, etc.) [Citation4]. MSCs are relatively rare cells in tissue, with bone marrow containing 1 MSCs per 10,000 to 100,000 nucleated cells [Citation6]. In order to generate therapeutically relevant doses (1–2 million/kg body weight), MSCs need to undergo massive in vitro cell expansion, which increases both the risk of mutagenesis and loss of activity. Tissue sources such as the skin, in which larger numbers of fibroblasts can be initially extracted, might serve as an attractive alternative to bone marrow-derived MSCs [Citation4]. Indeed, fibroblasts and MSCs share surface markers and a similar ability to differentiate and immunmodulate [Citation1,Citation5,Citation7]. Ex vivo expanded fibroblasts are used extensively for the production of artificial skin for the treatment of burns and for other cell and tissue engineering applications including facial scars, diabetic foot ulcers, urinary incontinence, gingival repair [Citation8,Citation9], degenerative disc disease [Citation10], epidermolysis bullosa, and cardiac or tendon regeneration [Citation11], to mention only a few.
Fetal bovine serum (FBS) is the current gold standard for the expansion of human cell therapy products, including fibroblast-based products. However, FBS suffers from significant drawbacks, including its ill-defined nature, batch-to-batch variability, xenogeneic origin and consequent safety and animal welfare concerns, as well as other economic, sourcing, and supply issues [Citation12]. Regulatory agencies and the scientific community [Citation13–15] have encouraged the development of methods for cell expansion that do not use animal products, especially ruminant material, which can potentially transmit prions and adventitious viruses.
Human platelet lysate (HPL), produced in most cases from expired platelets, has been used as a xeno-free culture supplement to expand different cell types for the last 40 years [Citation16], but its use has recently grown explosively due to the need of safely replacing FBS for clinical scale manufacturing. Accordingly, numerous studies have evaluated the effect of HPL products on MSCs biology, but studies on the use of HPL for fibroblast expansion are limited. Although different HPL production protocols have been described in the literature (reviewed in [Citation13]), there is no clear consensus on the optimal HPL manufacturing process, hindering standardization and characterization [Citation12].
In the last decade, public blood transfusion centers have progressively increased their healthcare activity and integrated cutting-edge technologies and developments in line with scientific advances. However, according to data from the National System for Transfusional Security in Spain, in 2015, a surplus of 373,055 liters of unused plasma was channeled into biopharmaceutical companies [Citation17]. Likewise, the number of expired or discarded platelet units was close to 40,000. The use of routinely discarded platelets to produce HPL could herald the development of new therapeutic applications and cost savings within the public health system setting.
In the present study, we collected platelets approaching expiry from the blood bank of our healthcare system and produced HPL products using several different protocols. We then tested these products as culture supplements for dermal fibroblasts and developed a GMP-grade HPL. Fibroblasts cultured with our proprietary HPL solution maintained their extracellular matrix (ECM) generation capacity and expression of fibroblast markers, and showed a normal karyotype with only minor gene expression changes, indicating that FBS can be safely replaced by the HPL solution. Our in-house produced GMP HPL is an efficient, safe and economical cell culture supplement that can help enhance the healthcare activity of blood transfusion centers through the re-use of plasma and platelets approaching expiry.
Methods
Fibroblast Isolation
Skin biopsies were obtained from three deceased donors using 4-mm2 punches (Stiefel; Watford, London, UK) and were maintained in BASE.128 decontamination medium (Alchimia srl; Padua, Italy) until processing. Biopsies were washed with phosphate buffered saline (PBS; Sigma-Aldrich, St. Louis, MO) supplemented with 1% penicillin/streptomycin (P/S; Sigma-Aldrich) and cut into small pieces (approximately 1 × 1 mm) using a sterile scalpel. Tissue explants were directly plated onto the bottom of cell culture-treated 6-well plates. The ethics committee of the University Hospital Virgen del Rocío approved the study and samples were obtained after informed written consent according to Spanish law (RD 1301/2006).
Fibroblast Culture
Adult dermal fibroblasts from the three independent donors were cultured in Dulbecco’s modified Eagle’s medium (DMEM; Sigma-Aldrich) containing 1% non-esential amino acids 100× (NEAA; Sigma-Aldrich), 20 µg/mL gentamycin (Normon, Madrid, Spain), 1% GlutaMaxTM (Life Technologies, Invitrogen, Gaithesburg, MO), and supplemented with 10% FBS (Sigma-Aldrich), 10% commercial HPL (StemulateTM, Cook Medical, Bloomington, IN), 10% AB human serum (ABHS) from two different manufacturers (ABHS1 from Sigma-Aldrich and ABHS2 from Invitrogen, Carlsbad, CA) or 5% in-house produced solutions. ABHS1 was also tested at 10% supplemented with 2 µg/L transforming growth factor-beta 1 (TGF-β1; Gibco, Fisher Scientific, Madrid, Spain), 10 µg/L fibroblast growth factor (FGF; Thermo Scientific, Waltham, MA) and 100 nM hydrocortisone (Sigma-Aldrich). Heparin (2 U/mL, Rovi, Madrid, Spain) was added to the cell culture media supplemented with HPL solutions to prevent undesired clotting.
In-house Produced Human Platelet Lysate
Six bags (30 donors in total) of group 0 leukodepleted platelet concentrates from buffy coats of healthy donors were used just before their expiry date (day 5 after isolation) to manufacture different HPL solutions (HPL1- HPL4) according to the scheme described in . First, we pooled the volume of the bags into a single container and then distributed the same volume into four bottles to obtain the four different starting solutions. HPL1 was produced by direct freezing/thawing (two cycles) of the starting solution. For the remainder of the solutions, the platelets of the starting solution were concentrated, counted and resuspended to a final concentration of 109 platelets/mL. Platelets were centrifuged (2500 × g 10 min at room temperature) and the pellet was resuspended in a solution consisting of 60% CompoSol® (Fresenius-kabi AG, Bad Homburg, Germany) and 40% AB plasma (for HPL2 and HPL3) or in a solution consisting of 80% PBS (Gibco, Fisher Scientific, Madrid, Spain) and 20% AB plasma (for HPL4). Subsequently, all HPL solutions were subjected to two freeze/thaw cycles and platelet cell debris was removed by two centrifugation cycles. The supernatants were divided into several bottles and frozen at −80ºC and −20ºC for testing. For the HPL3 solution, a final filtration step (Corning Costar, Celbio, Milan, Italy) was performed after the centrifugation cycles. All donors tested negative for HIV, hepatitis B and C and Treponema pallidum (syphilis) according to Spanish law (RD 9/2014). Samples were provided by the Centro de Transfusiones, Tejidos y Células of Seville. Informed written consent was obtained from all donors according to Spanish law (RD 1088/2005).
Figure 1. Production of in-house human platelet lysate solutions. A) Production of human platelet lysate (HPL) following different protocols. B) Population doublings per day of fibroblasts grown with 10% FBS, 5% HPL-CM, 5% HPL1, 5% HPL2, 5% HPL3 or 5% HPL4 solutions for 3 passages. Data represent mean ± S.E.M from fibroblasts of 3 different donors. Statistical significance was determined by one-way ANOVA followed by Tukey’s multiple comparison test, *, p ≤ 0.05 and **, p ≤ 0.01. C) All cells expressed fibronectin and collagen type I after culture for 5 passages with 10% FBS, 5% HPL2 or 5% HPL-CM. No significant differences were observed between conditions. Representative confocal images (maximum projections) are shown. Scale bar, 50 µm.
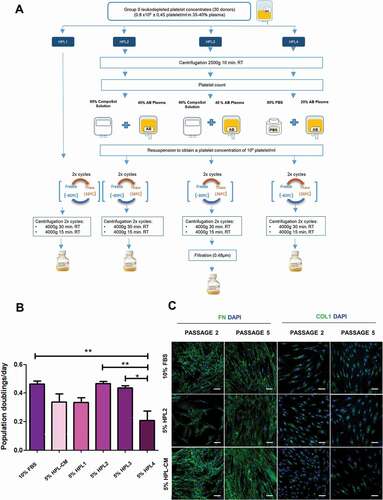
Gene Expression Analysis
Fibroblasts (n = 3) were cultured for 8 passages in DMEM supplemented with 1% NEAA, 20 µg/mL gentamycin, 1% Glutamax, 2 U/mL heparin (when using HPL), and with 10% FBS, or 5% or 15% in-house HPL2 solution. RNA was extracted with the RNeasy® Mini kit (Qiagen, Hilden, Germany) and RNA quality was analyzed on the Bioanalyzer 2100 platform (Agilent Technologies Inc., Santa Clara, CA). All samples had an RNA Integrity Number greater than 8. These samples were used for cDNA synthesis, which was followed by biotin labeling and hybridization to independent Human Clarion-STM Microarrays (Affymetrix, Santa Clara, CA). Microarrays were scanned with the AffymetrixGeneChip Scanner 7 G, and data were analyzed with Affymetrix® GeneChip® Command Console® v2.0 software. Microarray analysis was carried out in the Genomics Unit of the Centro Andaluz de Biología Molecular y Medicina Regenerativa (Seville, Spain). The microarray expression dataset is publicly available at the GEO repository (https://www.ncbi.nlm.nih.gov/geo/) under the identifier GSE147146. Further analyses were performed using Transcriptome Analysis Console (TAC, Affymetrix) v4.0 software and R version 3.6.1. Differentially expressed genes (DEGs) were obtained applying a threshold of ±2-fold change and a false discovery rate (FDR) p-value less than or equal to 0.05 (FDR ≤0.05). Functional enrichment analysis was performed using the bioinformatics tool EnrichR (https://amp.pharm.mssm.edu/Enrichr/) [Citation18,Citation19].
Statistics
Data are presented as mean ± SEM from three different donors. The statistical tests applied are indicated in the figure legends. Differences were considered to be statically significant at a p-value of 0.05. All statistical analyses were performed using GraphPad Prism 5, version 5.01 (GraphPad Software Inc., San Diego, CA). Additional methods are detailed in supplementary methods.
Results
Manufacture and Characterization of Different In-house Produced HPL Solutions
To evaluate a suitable cell culture supplement for the expansion of human dermal fibroblasts in a clinical context, we first compared commercial allogeneic ABHS with FBS. A priori, ABHS had some advantages over FBS (e.g., xeno-free supplement), but we found that the cell proliferation rate was significantly lower in fibroblasts cultured in 10% ABHS than in the same cells cultured in 10% FBS, and with some cell batches acquiring a senescent-like morphology (Figure S1A) with loss of cell division. We then compared fibroblast proliferation in the presence of different supplements suitable for clinical use: 10% ABHS supplemented with FGF, hydrocortisone and TGF-β1 (ABHS1+ GF), 10% commercial HPL (HPL-CM) and 10% FBS as a control. Fibroblasts showed the highest proliferation rate when cultured with 10% ABHS1+ GF, followed by 10% HPL-CM, which also performed well (Figure S1B). No morphological changes were observed between conditions (Figure S1C). Taking into account the cost of the culture supplements (for each 100 mL: 202 Euro for ABHS, 375 Euro for ABHS+GF, 220 Euro HPL-CM and 160 Euro for FBS), we decided to develop an in-house produced HPL using platelets approaching expiry from the transfusion center of our public healthcare system.
We designed four different protocols to develop in-house HPL products using platelets from group 0 donors and AB plasma to avoid potential problems with antigens and isoagglutinins. The four HPL solutions mainly differed in the additives used to resuspend the platelets, the percentage of plasma in the final product, the starting concentration of platelets, and the addition of a final filtration step (see details in ). We then tested and compared the different HPL products on fibroblast proliferation (measured as population doublings per day). Fibroblasts cultured with 5% HPL2 showed the highest proliferation rate, which was similar to that of cultures supplemented with 10% FBS, whereas 5% HPL4 was poorest for proliferation (). Fibroblasts cultured with 5% HPL3, 5% HPL1 or 5% HPL-CM showed intermediate rates of proliferation. HPL2 contained 40% plasma, suggesting that plasma-derived growth factors are important to promote fibroblast proliferation. Although not significant, the proliferation rate obtained with HPL3 (filtered solution) was slightly lower than that with HPL2, indicating that some growth factors were likely removed by the filtration step. Also, we noted that cells cultured with HPL2 and HPL3 were smaller in size to those cultured with FBS (Figure S2). Fibroblasts cultured with 5% HPL2, 10% FBS, and 5% HPL-CM displayed a similar capacity to generate ECM components (Fibronectin and Collagen type 1) along passages, as observed by immunocytochemistry (). Given these results, we chose HPL2 for further characterization.
We first compared different concentrations of HPL2 as a supplement for culture media (5, 10 and 15%). As shown in , we observed significant differences between 5% and 15% both in doubling time and total cell yield (p < .05), and between 10% and 15% in doubling time (p < .05). There were no evident changes in the protein expression of phenotypic markers including CD13, Vimentin, Collagen type 1, Fibronectin () and CD44, CD90, CD13 () between conditions. Importantly, fibroblasts cultured with 5% or 15% HPL2 at passage 12 (approximately 22 accumulated population doublings) maintained a normal karyotype (Figure S3).
Figure 2. Characterization of fibroblasts grown with different human platelet lysate concentrations. A) Population doubling time and B) number of fibroblasts obtained when cells were grown in 5%, 10% or 15% of HPL2 for at least 3 passages. Data are mean ± S.E.M from fibroblasts of 3 different donors. Statistical significance was determinate by one-way ANOVA test followed by Tukey’s multiple momparison test *, p ≤ 0.05. (C) Immunocytochemsitry analysis for CD13, vimentin (VIM), collagen type I (COL) and fibronectin (FN). Representative confocal images (maximum projections) are shown. Scale bar, 50 µm. D) Flow cytometry analysis showing positivity for CD44, CD90, CD13 and negativity for HLA-DR and CD324 of fibroblasts grown in 5% or 15% HPL2. No significant differences were observed between both supplements (5% and 15%) or with the control group (10% FBS). E) Exponential growth kinetics of fibroblasts (n = 3) grown with different concentrations of HPL2 (5%, 10% and 15%) and 10% FBS. Statistical significance was determinate by two-way ANOVA. No significant differences were observed.
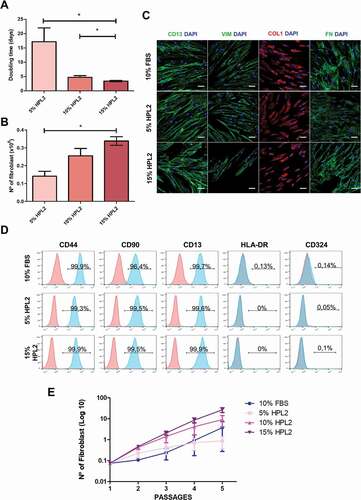
We also studied the stability of the medium supplemented with HPL2 at 4ºC. To this end, we cultured cells for four weeks with medium supplemented with 5%, 10% and 15% HPL2 solution that were stored at 4ºC. As shown in , no significant differences were observed regarding the proliferation capacity of fibroblasts cultured with the different concentrations of HPL2 for at least 40 days, indicating that HPL can be stored at 4ºC for at least four weeks. However, it would be preferable to use media supplemented with 5% HPL2 within 2 weeks to obtain similar cell yields to those of 10% FBS.
Fibroblast Gene Expression Analysis in Response to Different Supplements
To assess whether the HPL2 supplement affected the transcriptome of the cultured fibroblasts, which might compromise their use in a clinical setting, we next performed microarray analysis. Thus, we analyzed the gene expression profiles of fibroblasts from the 3 different donors cultured with our in-house manufactured HPL2 at two different percentages (5% and 15%) and with 10% FBS (control group). Using an FDR p-value ≤0.05 and ±2-fold change, we found a total of 129 DEGs when comparing 5% HPL2 versus 10% FBS, of which 38 were upregulated and 89 downregulated (). The same analysis comparing 15% HPL2 versus 10% FBS resulted in only 29 DEGs, of which 5 were upregulated and 24 downregulated (). Overall, 20 DEGs were shared between the two comparison groups, with significant downregulation of 17 genes and significant upregulation of 3 genes in the HPL groups when compared with the control group (). Supporting our earlier observations (), genes related to the ECM (VIN, COL1A2, FN1), cell adhesion and cell communication (CD90, CD13, CD44) were unchanged between groups (). Also, HLA-DR and tissue factor (TF) expression was not significantly different between the 3 conditions (). We then performed an enrichment analysis on the DEGs to investigate possible molecular interactions, metabolic pathways and/or biological processes altered between the growth conditions. In the fibroblasts cultured with HPL2 we identified upregulated KEGG pathways related to tyrosine metabolism (ADH1B, AOX1), cytokine-cytokine receptor interaction (BMP4, BMP6, IL20RB, ACKR3), cell adhesion molecules (NEGR1, CNTN1, NRCAM) and TGF-β signaling (BMP4, BMP6); and downregulated KEGG pathways related to glycolysis/gluconeogenesis (ALDH1B1, LDHA, PKM, PFKP), fluid shear stress and atherosclerosis (NQO1, MGST3, HMOX1, SDC1, CYBA), regulation of actin cytoskeleton (SCIN, CFL1, ITGA6, ITGA5, PFN1, MYL9), ECM-receptor interaction (SDC1, ITGA6, ITGA5), cellular senescence (MAP2K3, CCNA2, CCNE2,,CDK2), GAP function (TUBB6, TUBB4B, TUBA4A), focal adhesion (SHC1, ITGA6, ITGA5, MYL9) and cell cycle (CCNA2, CCNE2, CDK2) (). We validated the expression of some of these genes using RT-qPCR, which corroborated our findings in the microarray analysis (Figure S4).
Figure 3. Gene expression profiling of fibroblasts grown with human platelet lysate concentrations. A) Venn diagrams show upregulated (upper panel) and downregulated (bottom panel) differentially expressed genes (DEGs) in fibroblasts cultured with 5% HPL2 versus 10% FBS and with 15% HPL2 versus 10% FBS. B, C) Volcano plots of DEGs at fold change ±2 and FDR p-value ≤0.05. Common DEGs found in both comparison groups (3 upregulated, 17 downregulated) are highlighted in bold. D) Expression levels of characteristic fibroblast markers and other relevant genes (HLA-DR and TF). E) KEGG enriched biological processes of up- and down-regulated DEGs in both comparison groups.
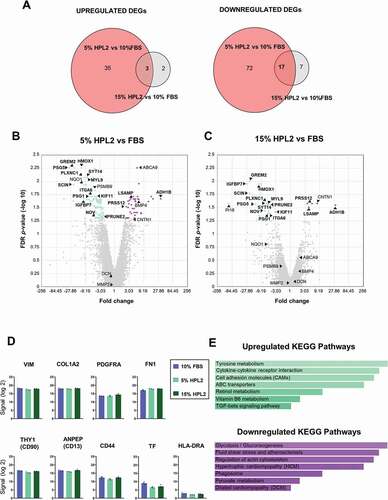
Validation of Human Platelet Lysate Manufacturing under GMP Conditions
In the context of human clinical use, any cell or gene therapy medicinal product must be manufactured with special quality requirements to ensure safety. To this end, once we had verified the efficacy and safety of our in-house produced platelet lysate, we developed a full GMP-compliant manufacturing process for the generation of clinical-grade HPL2. The process was performed in a clean room that complied with GMP and European Medicines Agency pharmaceutical standards [Citation20–23]. The facility has the certificate of GMP compliance from the Spanish Agency of Medicines and Medical Devices (in Spanish, AEMPS) for the generation of autologous and allogeneic skin substitutes. We created a documentation system to cover the procedures and equipment to comply with GMP regulation throughout the full HPL manufacturing process. We established the necessary in-process controls and the final quality controls for HPL to be performed before the release of the finished product: mycoplasma, sterility, endotoxin level, activity test, pH, PDGF-AB levels according to the European Pharmacopoeia, Chapter 2.6 Biological tests. The validation of the HPL production process was performed by manufacturing 3 batches of HPL2 following the protocol indicated in . The three batches passed all the quality controls, demonstrating the robustness and reproducibility of the process (). Once validated, we presented all the documentation and received the GMP certificate from AEMPS for producing fibroblasts using clinical-grade HPL2 as a cell culture supplement (N/REF: DCIM/INSP/CFT-MM). We then manufactured 7 different batches to test the replicability of the process. In all cases, fibroblasts showed similar or higher proliferation rates with 5% GMP-HPL2 than with 10% FBS (). Similarly, cells displayed a normal fibroblastic morphology () and maintained the expression of the fibroblast markers CD13, Fibronectin, Vimentin (), CD44, CD13 and Collagen type 1 (). All microbiological controls met the acceptance criteria (). PDGF-AB was tested to verify that platelet alpha granules were released (). We did not observe any significant differences in the promotion of fibroblast proliferation, pH, PDGF-AB, total proteins or albumin levels when we compared the HPL immediately after manufacturing with HPL that had been stored at −80°C or −20°C for 24 months (Figure S5).
Table I. Quality Controls performed in each batch. The acceptation limit for each release test is indicated in brackets. For the activity test FBS was used as control
Figure 4. Human platelet lysate GMP validation. A) Scheme of the GMP compliant manufacturing process (production + quality controls) of the HPL2 product. B) Activity test (doubling time of fibroblasts grown in 5% HPL2 versus 10% FBS). Statistical significance was determinated by two-tailed Student´s t-test. No significant differences were observed. C) Morphology of cells grown in the presence of a representative batch of GMP-HPL2. Scale bar, 200 µm. Inmunophenotypic characterization of fibroblasts cultured in 5% HPL2 over 5 passages by immunocytochemistry (D) and flow cytometry (E). All batches were positive for CD13, fibronectin (FN), vimentin (VIM), CD44, collagen type I (COL) and negative for HLA-DR. Data are mean ± S.E.M of 7 batches. Scale bar: 50 µm.
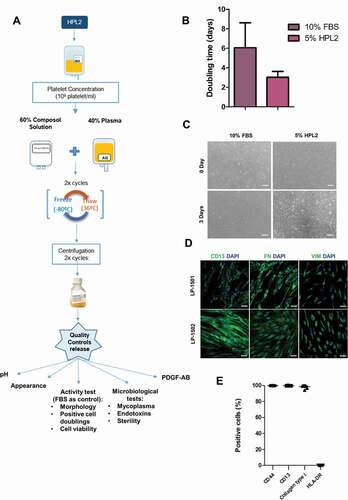
Discussion
In the present study, we assessed different culture medium supplements to obtain an optimal starting material for the manufacturing of fibroblast-based cell therapy products. Our findings indicate that HPL is a good candidate to replace FBS as growth supplement for fibroblast culture in a clinical context, as reported in other studies examining MSCs [Citation13,Citation24–26]. However, the price of commercial HPL is high, which significantly increases cell manufacturing costs. Therefore, we developed a propietary HPL that allows the use of expired platelets, optimizing the resources from our facilities both economically and technically. Different preparation methods are known to exert significant impacts on the final quality of HPL solutions [Citation27,Citation28] and, consequently, may also alter the cell product, thus influencing the potency and therapeutic benefit, and ultimately patient outcomes [Citation12]. Our study shows that a medium supplemented with our in-house HPL2 solution is an efficient, safe and economical solution from a clinical use perspective. Our HPL2 solution promoted fibroblast expansion and the maintenance of a normal phenotype and karyotype to the same level as that of FBS and commercial HPL. HPL2 is a human platelet lysate supplemented with 40% AB plasma in CompoSol®, an isotonic solution commonly used to store blood derivates. It has been previously described that HPL supplementation with plasma proteins is required to achieve good cell expansion [Citation29,Citation30]. Plasma contains high concentrations of chemokine, attachment factors and mitogens [Citation31] that likely play important roles in cell proliferation, chemotaxis, cell differentiation, regeneration and angiogenesis [Citation32]. It has been demonstrated that HPL supplemented with plasma is more efficient for cell expansion than unsupplemented HPL [Citation29]. Although clots and aggregates formed during freezing/thawing cycles are not eliminated, non-filtered platelet lysate seems to be better than a filtered solution, perhaps due to the removal of some important components for cell growth during the filtering process. As reported in other studies [Citation13,Citation24–26], the additive solution is also important to achieve a higher quality of the product [Citation33,Citation34].
Data regarding the stability of HPL solutions are limited. We show here that our solution remains stable in the supplemented medium for 40 days at 4ºC without significant loss of activity, which is similar to results from the Reems laboratory [Citation35]. Also in agrement with our results, the same laboratory reported that HPL can be stored at −80ºC for 24 months [Citation36]. We further show that the HPL remains stable for two years at −20ºC, maintaining its growth promotion activity in fibroblasts.
Several studies have evaluated the effect of HPL on MSCs expansion [Citation37–46], including gene expression profiling [Citation42–45]. However, there remains a paucity of studies on the use of HPL on fibroblasts. Our results confirm that only a handful of genes are modified by culture with HPL and none of them are related to the acquisition of a tumorigenic or senescent phenotype. Among the few changes we detected, we found upregulated DEGs in HPL2 (versus 10% FBS) involved in several metabolic pathways (ABCA9, ABCC9, ADH1B, AOX1, CBS, NFIB, MKX, BMP4) and cell adhesion processes (NEGR1, CNTN1, NRCAM, CDH18) [Citation18,Citation19,Citation47–49], whereas genes associated with cellular senescence such as MAP2K3 [Citation50], CCNA2 [Citation51], CCNE2, CDK2, and IGFBP7 [Citation52], were downregulated. This might be a reason for the higher yields observed in the characterization studies. Similarly, scinderin (SCIN), a gene involved in cell proliferation inhibition [Citation53], was downregulated in HPL2-treated cells. In line with these findings, fibroblasts cultured with 10% FBS showed an upregulation of genes associated with stress responses, such as NQO1, HMOX1 and CYBA [Citation54]. Overall, fibroblasts cultured with our HPL2 product seem to have a genetic profile biased slightly toward cell proliferation. Interestingly, the expression of HLA-DR and TF, the principal component eliciting the instant blood-mediated inflammatory reaction, was not affected by culture with HPL, suggesting that its use as a supplement is a safe option for the production of allogeneic fibroblast-based cell products. However, hemocompatibiliy tests should be performed before the systemic application of fibroblasts, as has been indicated for intravascular delivery of other cell types [Citation55,Citation56].
We also validated the production of an HPL product under GMP conditions, which included an inspection by the AEMPS, and we obtained a GMP certificate for producing fibroblasts using clinical-grade HPL2 as a cell culture supplement. Accordingly, our HPL product can be used for the expansion of cells for clinical purposes or as a medicinal product itself. However, the pooling of a large number of platelet units has been recently under analysis by the regulatory authorities, citing concerns of viral contamination and suggesting limiting pool sizes unless methods for virus inactivation are applied [Citation57]. Technologies for pathogen reduction treatment (PRT) that can effectively decrease the potential viral and bacterial content of blood products have indeed been developed (reviewed in [Citation12]). This is now a relevant issue as international travel and emerging pathogens such us SARS-CoV2 or Zika virus may entail challenges in manufacturing safe blood products. Therefore, we are currently developing a viral inactivated HPL. However, although PRT would be desirable, GMP-HPL2 batches have been tested for adventitious viruses through the cytopathic effect test. This test allows for the detection of many viruses including SARS-CoV2 [Citation58,Citation59].
In conclusion, our data show that the GMP-HPL2 product is an attractive alternative to FBS and other commercial blood derivates for fibroblast cell culture in a clinical context. Furthermore, it provides a route for blood banks and transfusion centers to share and optimize available resources within a public health system setting.
Acknowledgements
We acknowledge members of the Unidad de Terapia Celular, Reina Sofía University Hospital, Córdoba, Spain and Unidad de Terapia Celular, Virgen de las Nieves Hospital, Granada for PDGF-AB assay and sterility testing, respectively, and all the members of Centro Transfusional, Células y Tejidos of Seville for their support. We thank Dr. Sanchez Pernaute for valuable advice and assistance during the planning and execution of this work and Dr. Cristina Rosell-Valle for assistance with statistical analysis.
Author contributions
Conception and design of the study: MSG. Acquisition of data: ILR, RCC, MGB, LNN, MMA, BAA, SNM, MSG. Analysis and interpretation of data: MSG, LNN, BFM, GCS Statistical analysis: MSG, LNN, BFM. Study supervision: MSG. Drafting of figures and manuscript: LNN, BFM, MSG. All authors have approved the final article.
Declaration of Competing interest
MSG, BFM and ILR are authors of a patent application for the use different media based on HPL (nº application Spanish Patent Office: P201730713). The authors have no other commercial, proprietary, or financial interest in the products or companies described in this article.
Additional information
Funding
References
- Haniffa MA, Collin MP, Buckley CD, Dazzi F. Mesenchymal stem cells: the fibroblasts’ new clothes? Haematologica 2009;94(2).258–263. doi:https://doi.org/10.3324/haematol.13699.
- Alberts B, Johnson A, Lewis J, Raff M, Roberts K, Walter P. Molecular biology of the cell. 4th ed. New York; Garland Science; 2002. 1616 p.
- Junker JPE, Sommar P, Skog M, Johnson H, Kratz G, et al. Adipogenic, chondrogenic and osteogenic differentiation of clonally derived human dermal fibroblasts. Cells Tissues Organs 2010;191(2).105–118. doi:https://doi.org/10.1159/000232157.
- Ichim TE, O’Heeron P, Kesari S. Fibroblasts as a practical alternative to mesenchymal stem cells. J Transl Med 2018;16(1):212. doi:https://doi.org/10.1186/s12967-018-1536-1.
- Wada N, Bartold PM, Gronthos S. Human foreskin fibroblasts exert immunomodulatory properties by a different mechanism to bone marrow stromal/stem cells. Stem Cells Dev 2011;20(4):647–659. doi:https://doi.org/10.1089/scd.2010.0246.
- Pittenger MF, Martin BJ. Mesenchymal stem cells and their potential as cardiac therapeutics. Circ Res 2004;95(1):9–20. doi:https://doi.org/10.1161/01.RES.0000135902.99383.6f.
- Cappellesso-Fleury S, Puissant-Lubrano B, Apoil P-A, Titeux M, Winterton P, Casteilla L, Bourin P, Blancher A. Human fibroblasts share immunosuppressive properties with bone marrow mesenchymal stem cells. J Clin Immunol 2010;30(4).607–619. doi:https://doi.org/10.1007/s10875-010-9415-4.
- Dominiak M, Saczko J, Gerber H, Rybak Z, Gredes T. Use of primary culture of human fibroblasts in gingiva augmentation procedure. Biomed Tech (Berl) 2010;55(6).331–334. doi:https://doi.org/10.1515/bmt.2010.052.
- Milinkovic I, Aleksic Z, Jankovic S, Popovic O, Bajic M, Cakic S, Lekovic V. Clinical application of autologous fibroblast cell culture in gingival recession treatment. J Periodontal Res 2015;50(3).363–370. doi:https://doi.org/10.1111/jre.12215.
- Ural IH, Alptekin K, Ketenci A, Solakoglu S, Alpak H, Özyalçın S. Fibroblast transplantation results to the degenerated rabbit lumbar intervertebral discs. Open Orthop J 2017;11(1).404–416. doi:https://doi.org/10.2174/1874325001711010404.
- Costa-Almeida R, Soares R, Granja PL. Fibroblasts as maestros orchestrating tissue regeneration. J Tissue Eng Regen Med 2018;12(1).240-251.doi:https://doi.org/10.1002/term.2405.
- Bieback K, Fernandez-Munoz B, Pati S, Schäfer R. Gaps in the knowledge of human platelet lysate as a cell culture supplement for cell therapy: a joint publication from the AABB and the international society for cell & gene therapy. Cytotherapy 2019;21(9).911–924. doi:https://doi.org/10.1016/j.jcyt.2019.06.006.
- Burnouf T, Strunk D, Koh MBC, Schallmoser K. Human platelet lysate: replacing fetal bovine serum as a gold standard for human cell propagation? Biomaterials 2016;76:371–387. doi:https://doi.org/10.1016/j.biomaterials.2015.10.065.
- Agency EM. Guideline on the quality, non-clinical and clinical aspects of gene therapy medicinal products. Table of contents 2018;44.
- European Medicines Agency. Guideline on the use of bovine serum in the manufacture of human biological medicinal products. EMA/CHMP/BWP/457920/2012 rev.1. 2012.
- Choi YC, Morris GM, Sokoloff L. Effect of platelet lysate on growth and sulfated glycosaminoglycan synthesis in articular chondrocyte cultures. Arthritis Rheum 1980;23(2):220–224. doi:https://doi.org/10.1002/art.1780230213.
- Ministry of Health Social Services and Equality (Currently Ministry of Health Consumer Affairs and Social Welfare). Annual report on the national health system of Spain 2016. 2018.
- Kuleshov MV, Jones MR, Rouillard AD, Fernandez NF, Duan Q, Wang Z, Koplev S, Jenkins SL, Jagodnik KM, Lachmann A. Enrichr: a comprehensive gene set enrichment analysis web server 2016 update. Nucleic Acids Res 2016;44(W1):W90–W97. doi:https://doi.org/10.1093/nar/gkw377.
- Chen EY, Tan CM, Kou Y, Duan Q, Wang Z, Meirelles GV, Clark NR, Ma’ayan A. Enrichr: interactive and collaborative HTML5 gene list enrichment analysis tool. J Eng Res 2013;14.128.
- Commission Directive 2003/94/EC of 8 October 2003. Laying down the principles and guidelines of good manufacturing practice in respect of medicinal products for human use and investigational medicinal products for human use. Off J Eur Union 2003;L 262:22–26.
- Commission Directive 2006/17/EC of 8 February 2006. Implementing Directive 2004/23/EC of the European Parliament and of the Council as regards certain technical requirements for the donation, procurement and testing of human tissues and cells. Off J Eur Union 2006;L 38:40–52.
- Directive 2004/23/EC of the European Parliament and of The Council of 31 March 2004 on setting standards of quality and safety for the donation, procurement, testing, processing, preservation, storage and distribution of human tissues and cells. Off J Eur Union 2004;L 102:48–58.
- Directive 2002/98/EC of the European Parliament and of The Council of 27 January 2003 setting standards of quality and safety for the collection, testing, processing, storage and distribution of human blood and blood componentsand amending Directive 2001. Off J Eur Union 2003;L 33:30–40.
- Bieback K. Platelet lysate as replacement for fetal bovine serum in mesenchymal stromal cell cultures. Transfus Med Hemother 2013;40(5):326–335. doi:https://doi.org/10.1159/000354061.
- Kocaoemer A, Kern S, Kluter H, Bieback K. Human AB serum and thrombin-activated platelet-rich plasma are suitable alternatives to fetal calf serum for the expansion of mesenchymal stem cells from adipose tissue. Stem Cell 2007;25(5).1270–1278. doi:https://doi.org/10.1634/stemcells.2006-0627.
- Huang C-J, Sun Y-C, Christopher K, Pai AS-I, Lu C-J, Hu F-R, Lin S-Y, Chen W-L. Comparison of corneal epitheliotrophic capacities among human platelet lysates and other blood derivatives. PLoS One 2017;12(2).e0171008. doi:https://doi.org/10.1371/journal.pone.0171008.
- Pawitan JA. Platelet rich plasma in xeno-free stem cell culture: the impact of platelet count and processing method. Curr Stem Cell Res Ther 2012;7(5):329–335. doi:https://doi.org/10.2174/157488812802481508.
- European Directorate for the Quality of Medicines & HealthCare (EDQM). Guide to the preparation, use and quality assurance of blood components. 19th ed. Strasbourg; Council of Europe; 2017.
- Horn P, Bokermann G, Cholewa D, Bork S, Walenda T, Koch C, Drescher W, Hutschenreuther G, Zenke M, Ho AD, et al. Impact of individual platelet lysates on isolation and growth of human mesenchymal stromal cells. Cytotherapy 2010;12(7).888–898. doi:https://doi.org/10.3109/14653249.2010.501788.
- Everts PAM, Knape JTA, Weibrich G, Schonberger JPAM, Hoffmann J, Overdevest EP, Box HAM, van Zundert A. Platelet-rich plasma and platelet gel: a review. J Extra Corpor Technol 2006;38:174–187.
- Blair P, Flaumenhaft R. Platelet alpha-granules: basic biology and clinical correlates. Blood Rev 2009;23(4):177–189. doi:https://doi.org/10.1016/j.blre.2009.04.001.
- Werner S, Grose R. Regulation of wound healing by growth factors and cytokines. Physiol Rev 2003;83(3):835–870. doi:https://doi.org/10.1152/physrev.2003.83.3.835.
- Azuma H, Hirayama J, Akino M, Ikeda H. Platelet additive solution - electrolytes. Transfus Apher Sci 2011;44(3).277–281. doi:https://doi.org/10.1016/j.transci.2011.03.002.
- Eriksson L, Hogman CF. Platelet concentrates in an additive solution prepared from pooled buffy coats. 1. In vitro studies. Vox Sang 1990;59:140–145.
- Mojica-Henshaw MP, Jacobson P, Morris J, Kelley L, Pierce J, Boyer M, Reems J-A. Serum-converted platelet lysate can substitute for fetal bovine serum in human mesenchymal stromal cell cultures. Cytotherapy 2013;15(12).1458–1468. doi:https://doi.org/10.1016/j.jcyt.2013.06.014.
- Pierce J, Benedetti E, Preslar A, Jacobson P, Jin P, Stroncek DF, Reems J-A. Comparative analyses of industrial-scale human platelet lysate preparations. Transfusion 2017;57(12).2858–2869. doi:https://doi.org/10.1111/trf.14324.
- Trojahn Kolle S-F, Oliveri RS, Glovinski PV, Kirchhoff M, Mathiasen AB, Elberg JJ, Andersen PS, Drzewiecki KT, Fischer-Nielsen A. Pooled human platelet lysate versus fetal bovine serum-investigating the proliferation rate, chromosome stability and angiogenic potential of human adipose tissue-derived stem cells intended for clinical use. Cytotherapy 2013;15(9).1086–1097. doi:https://doi.org/10.1016/j.jcyt.2013.01.217.
- Azouna N, Jenhani F, Regaya Z, Berraeis L, Othman T, Ducrocq E, Domenech J. Phenotypical and functional characteristics of mesenchymal stem cells from bone marrow: comparison of culture using different media supplemented with human platelet lysate or fetal bovine serum. Stem Cell Res Ther 2012;3(1).6. doi:https://doi.org/10.1186/scrt97.
- Bernardi M, Agostini F, Chieregato K, Amati E, Durante C, Rassu M, Ruggeri M, Sella S, Lombardi E, Mazzucato M, et al. The production method affects the efficacy of platelet derivatives to expand mesenchymal stromal cells in vitro. J Transl Med 2017;15(1).1–9. doi:https://doi.org/10.1186/s12967-017-1185-9.
- Abdelrazik H, Spaggiari GM, Chiossone L, Moretta L. Mesenchymal stem cells expanded in human platelet lysate display a decreased inhibitory capacity on T- and NK-cell proliferation and function. Eur J Immunol 2011;41(11).3281–3290. doi:https://doi.org/10.1002/eji.201141542.
- Schallmoser K, Bartmann C, Rohde E, Reinisch A, Kashofer K, Stadelmeyer E, Drexler C, Lanzer G, Linkesch W, Strunk D, et al. Human platelet lysate can replace fetal bovine serum for clinical-scale expansion of functional mesenchymal stromal cells. Transfusion 2007;47(8).1436–1446. doi:https://doi.org/10.1111/j.1537-2995.2007.01220.x.
- Fernandez-Rebollo E, Mentrup B, Ebert R, Franzen J, Abagnale G, Sieben T, Ostrowska A, Hoffmann P, Roux P-F, Rath B, et al. Human platelet lysate versus fetal calf serum: these supplements do not select for different mesenchymal stromal cells. Sci Rep 2017;7(1).5132. doi:https://doi.org/10.1038/s41598-017-05207-1.
- Schallmoser K, Bartmann C, Rohde E, Bork S, Guelly C, Obenauf AC, Reinisch A, Horn P, Ho AD, Strunk D, et al. Replicative senescence-associated gene expression changes in mesenchymal stromal cells are similar under different culture conditions. Haematologica 2010;95(6).867–874. doi:https://doi.org/10.3324/haematol.2009.011692.
- Dessels C, Ambele MA, Pepper MS. The effect of medium supplementation and serial passaging on the transcriptome of human adipose-derived stromal cells expanded in vitro. Stem Cell Res Ther 2019;10(1):253. doi:https://doi.org/10.1186/s13287-019-1370-2.
- Torensma R, Prins H-J, Schrama E, Verwiel ETP, Martens ACM, Roelofs H, Jansen BJH. The impact of cell source, culture methodology, culture location, and individual donors on gene expression profiles of bone marrow-derived and adipose-derived stromal cells. Stem Cells Dev 2013;22(7).1086–1096. doi:https://doi.org/10.1089/scd.2012.0384.
- Sondergaard RH, Follin B, Lund LD, Juhl M, Ekblond A, Kastrup J, Haack-Sørensen M. Senescence and quiescence in adipose-derived stromal cells: effects of human platelet lysate, fetal bovine serum and hypoxia. Cytotherapy 2017;19(1).95–106. doi:https://doi.org/10.1016/j.jcyt.2016.09.006.
- Kanehisa M, Goto S. KEGG: kyoto encyclopedia of genes and genomes. Nucleic Acids Res 2000;28(1):27–30. doi:https://doi.org/10.1093/nar/28.1.27.
- Kanehisa M, Sato Y, Furumichi M, Morishima K, Tanabe M. New approach for understanding genome variations in KEGG. Nucleic Acids Res 2019;47(D1).D590–D595. doi:https://doi.org/10.1093/nar/gky962.
- The Gene Ontology Consortium. The gene ontology resource: 20 years and still GOing strong. Nucleic Acids Res 2019;47(D1):D330–D338. doi:https://doi.org/10.1093/nar/gky1055.
- Jia M, Souchelnytskyi N, Hellman U, O’Hare M, Jat PS, Souchelnytskyi S. Proteome profiling of immortalization-to-senescence transition of human breast epithelial cells identified MAP2K3 as a senescence-promoting protein which is downregulated in human breast cancer. Proteomics Clin Appl 2010;4(10–11).816–828. doi:https://doi.org/10.1002/prca.201000006.
- Purcell M, Kruger A, Tainsky MA. Gene expression profiling of replicative and induced senescence. Cell Cycle 2014;13(24):3927–3937. doi:https://doi.org/10.4161/15384101.2014.973327.
- Benatar T, Yang W, Amemiya Y, Evdokimova V, Kahn H, Holloway C, Seth A. IGFBP7 reduces breast tumor growth by induction of senescence and apoptosis pathways. Breast Cancer Res Treat 2012;133(2).563–573. doi:https://doi.org/10.1007/s10549-011-1816-4.
- Zunino R, Li Q, Rose SD, Romero-Benítez MMI, Lejen T, Brandan NC, Trifaró J-M. Expression of scinderin in megakaryoblastic leukemia cells induces differentiation, maturation, and apoptosis with release of plateletlike particles and inhibits proliferation and tumorigenesis. Blood 2001;98(7).2210–2219. doi:https://doi.org/10.1182/blood.V98.7.2210.
- Bhakkiyalakshmi E, Sireesh D, Rajaguru P, Paulmurugan R, Ramkumar KM. The emerging role of redox-sensitive Nrf2-Keap1 pathway in diabetes. Pharmacol Res 2015;91:104–114. doi:https://doi.org/10.1016/j.phrs.2014.10.004.
- George MJ, Prabhakara K, Toledano-Furman NE, Wang Y-W, Gill BS, Wade CE, Olson SD, Cox CS. Clinical cellular therapeutics accelerate clot formation. Stem Cells Transl Med 2018;7(10):731–739. doi:https://doi.org/10.1002/sctm.18-0015.
- Moll G, Ankrum JA, Kamhieh-Milz J, Bieback K, Ringdén O, Volk H-D, Geissler S, Reinke P. Intravascular mesenchymal stromal/stem cell therapy product diversification: time for new clinical guidelines. Trends Mol Med 2019;25(2).149–163. doi:https://doi.org/10.1016/j.molmed.2018.12.006.
- European Directorate for the Quality of Medicines & HealthCare (EDQM). General chapter 5. 2.12. Raw materials for the production of cell-based and gene therapy products. European Pharmacopoeia; Strasbourg; Council of Europe; 2013.
- Park WB, Kwon NJ, Choi SJ, Kang CK, Choe PG, Kim JY, Yun J, Lee G-W, Seong M-W, Kim NJ, et al. Virus Isolation from the first patient with SARS-CoV-2 in Korea. J Korean Med Sci 2020;35(7).e84. doi:https://doi.org/10.3346/jkms.2020.35.e84.
- Zhu N, Zhang D, Wang W, Li X, Yang B, Song J, Zhao X, Huang B, Shi W, Lu R, et al. A novel coronavirus from patients with Pneumonia in China, 2019. N Engl J Med 2020;382(8).727–733. doi:https://doi.org/10.1056/NEJMoa2001017.