Abstract
Platelets are transfused to patients to prevent bleeding. Since both preparation and storage can impact the hemostatic functions of platelets, we studied platelet concentrates (PCs) with different initial composition in regard to platelet fragmentation and its impact on storage-induced changes in activation potential. Ten whole blood derived PCs were assessed over 7 storage days. Using flow cytometry, platelet (CD41+) subpopulations were characterized for activation potential using activation markers (PAC-1, P-selectin, and LAMP-1), phosphatidylserine (Annexin V), and mitochondrial integrity (DiIC1(5)). Aggregation response, coagulation, and soluble activation markers (cytokines and sGPVI) were also measured. Of the CD41+ events, the PCs contained a median of 82% normal-sized platelets, 10% small platelets, and 8% fragments. The small platelets exhibited procoagulant hallmarks (increased P-selectin and Annexin V and reduced DiIC1(5)). Normal-sized platelets responded to activation, whereas activation potential was decreased for small and abolished for fragments. Five PCs contained a high proportion of small platelets and fragments (median of 28% of CD41+ events), which was significantly higher than the other five PCs (median of 9%). A high proportion of small platelets and fragments was associated with procoagulant hallmarks and decreased activation potential, but, although diminished, they still retained some activation potential throughout 7 days storage.
Plain Language Summary
What is the context?
● Platelets are necessary to prevent and stop bleeding.
● Conditions associated with a low platelet count in the circulation, such as during chemotherapy treatment for hematologic cancer, can result in life-threatening bleeding. To prevent this, platelets from blood donors are transfused to these patients.
● The collection and preparation of platelet concentrates and subsequent storage before transfusion can affect the ability of the platelets to prevent bleeding.
● In this study, we investigated platelet concentrates prepared from whole blood and how their activation capacity was affected by the preparation and storage period.
What is new?
● We found that the platelet concentrates contained mainly low activated platelets of normal size, but also smaller platelets and platelet fragments.
● Unlike normal-sized platelets, small platelets and fragments exhibited hallmarks that are characteristic of pre-activation.
● Some platelet concentrates contained a relatively high proportion of small platelets and fragments already directly following preparation.
● Investigating several platelet activation markers, we found that platelet concentrates containing a high proportion of small platelets and platelet fragments showed lower activation capacity throughout the storage period.
What is the impact?
● We show that some platelet concentrates show lower activation capacity and might contain a substantial fraction of platelets with characteristics that might potentially trigger spontaneous blood coagulation. The variation between different concentrations is high, even though the preparation procedure is the same.
● If these differences will affect the efficacy of platelet transfusion is an important area for future studies.
Introduction
Platelet transfusions are critical in patients at significant risk of bleeding. Platelets adhere to substances exposed at the site of vessel damage to prevent bleeding. This leads to platelet activation, shape-change, release from granules and formation of a platelet aggregate. Some activated platelets provide a catalytic surface to which the coagulation factors bind and become activated, resulting in a fibrin network, stabilizing the platelet aggregate [Citation1–3].
Preparation and storage of platelet concentrates (PCs) induce platelet storage lesions (PSL), including changes in platelet metabolism, surface receptors, signaling, and secretion. PSL starts early during PC preparation and progress during storage (recently reviewed by [Citation4,Citation5]). Due to the complexity of platelet involvement in hemostasis, changes in platelet function in PCs are not fully understood.
To address this, we studied PCs with different initial compositions in regard to platelet fragmentation and its impact on storage-induced changes in activation potential. A novel flow cytometry protocol was used, which simultaneously evaluates five important platelet functions described below. PAC-1 binds to the active conformational form of the fibrinogen receptor GPIIb/IIIa, necessary for platelet aggregation [Citation6]. Annexin V binds to phosphatidylserine (PS), needed for assembly of the prothrombinase complex on platelets. Loss of DiIC1(5) staining indicates decreased mitochondrial membrane potential as occurs in procoagulant platelets [Citation7]. P-selectin and LAMP-1 exposures are signs of α-granule release and lysosomal exocytosis, respectively [Citation8]. Furthermore, the protocol allows for the analysis of platelet subpopulations, e.g., normal-sized platelets, the formation of smaller, procoagulant platelets, and platelet fragments (microparticles), which normally appear following strong stimulation of the platelets [Citation9]. Furthermore, aggregation capacity and release of soluble platelet activation markers were determined.
Methods
Each PC (n = 10) was prepared from five ABO-identical buffy coats from regular blood donors that had been held overnight before pooling. The standard procedures used for this at Linköping University Hospital have been described previously [Citation10] (but the special additional pooling procedure in [Citation10] was not applied here). The PCs were stored with constant agitation at 22 ± 2°C for 7 days. The PCs contained approximately 35% plasma and 65% SSP+ (MacoPharma, Mouvaux, France). We did not receive any information regarding the blood donors, and no results can be traced back to an individual.
Visual examination of swirling and sampling of the PCs was done on days 1, 5 and 7. Samples (10 mL), for various in vitro assays, were taken aseptically using a sampling bag (MacoPharma). The supernatant from each sample was prepared by centrifugation at 2500 g for 15 min at room temperature and stored at −80°C until analysis of metabolic variables and soluble markers. Platelet concentration, pH at 37ºC (only assessed on day 7), and the extracellular metabolic environment (lactate and glucose concentrations) were determined as per standard procedures at Linköping University Hospital.
Platelet subpopulations and platelet activation measurements by flow cytometry
Platelet function testing was performed using a six-color protocol as described by Södergren et al. [Citation9], but with some alterations regarding the platelet agonist solutions used in this study. To facilitate a standardized assay performance, the agonist solutions were prepared in advance and frozen at −20°C in capped tubes. Agonists used were cross-linked collagen-related peptide (CRP-XL; Gly-Cys-Hyp-(Gly-Pro-Hyp)10-Gly-Cys-Hyp-Gly-NH2, purchased from Prof. Richard Farndale, University of Cambridge, UK) at a final concentration of 1.33 µg/mL, thrombin receptor activating peptides, PAR1-AP (SFLLRN; JPT Peptide Technologies GmbH, Berlin, Germany) at a final concentration of 30 µM and PAR4-AP (AYPGKF; JPT Peptide Technologies GmbH) at a final concentration of 300 µM. Platelets were activated with each agonist separately and combined. Spontaneous platelet activation was determined using HEPES buffer instead of agonist. The HEPES buffer composition can be found in the article by Södergren et al. [Citation9]. The buffer was used in three variants, without calcium (referred to as HEPES), with 1.5 mM Ca2+ (referred to as HEPES-Ca2+), and with 10 mM EDTA (referred to as HEPES-EDTA).
The antibody mastermix used to assess the platelet markers contained HEPES-Ca2+, anti-CD41(GPIIb)-ECD (clone P2, 0.69 µg/mL; Beckman Coulter, Brea, CA), PAC-1-FITC (0.56 µg/mL, Becton Dickinson, Franklin Lakes, NJ), anti-P-selectin-PE (clone AK-4, 0.17 µg/mL, Becton Dickinson), anti-LAMP-1-PE-Cy7 (CD107a, clone H4A3; 0.5 µg/mL, Becton Dickinson), Annexin V-V450 (2.67 ng/mL, Becton Dickinson), and DiIC1(5) (1,1’,3,3,3’,3’-Hexamethylindodicarbocyanine Iodide; 30 nM, Molecular Probes, Eugene, OR). To determine background staining, the antibody mastermix used for these background fluorescence control samples contained HEPES or HEPES-EDTA, and anti-CD41-ECD (0.69 µg/mL), PAC-1-FITC (0.56 µg/mL), PE isotype control antibody (Mouse IgG1κ 0.17 µg/mL, Becton Dickinson), PE-Cy7 isotype control antibody (Mouse IgG1κ 0.5 µg/mL, Becton Dickinson), Annexin V-V450 (2.67 ng/mL), and DiIC1(5). To obtain the fluorescence background for DiIC1(5), CCCP (carbonyl cyanide 3-chlorophenylhydrazone; 100 µM, Sigma-Aldrich, St. Louis, MO) was added to one HEPES stimulated control sample.
To summarize the protocol, the agonist solutions were thawed in a 37°C water bath before adding antibody mastermixes. The platelet concentration of the sample was adjusted to 300 × 109/L by dilution with HEPES and 3 µL added to each tube with antibody mastermix and agonists (final volume of 36 µL). After 10 min incubation at room temperature, the labeling was terminated by 20 times dilution in HEPES-Ca2+ or HEPES for background fluorescence control samples. Flow cytometry (Gallios, Beckman Coulter) was performed immediately after dilution.
Flow cytometry acquisition and gating
Acquisition was performed in an enhanced wide angle mode (W2) for forward scatter (FSC) and with the threshold set on ECD fluorescence (corresponding to the CD41(GPIIb)-antibody), as this allows detection of small platelet-derived particles that would otherwise be removed by an FSC threshold [Citation9]. The fluorescence threshold was adjusted to prevent detection of debris in the gate for platelet fragments, while still being able to detect the appearance of particles in this gate upon strong stimulation of whole blood-derived platelets. Acquisition was performed for 90 sec or until 10 000 platelets had been collected in a temporary platelet gate. The flow cytometer performance was checked daily using procedures recommended by the manufacturer.
Kaluza v.1.3 (Beckman Coulter) was used for data analysis, as previously described [Citation9]. In brief, gates based on anti-CD41(GPIIb)-ECD fluorescence and FSC properties were set dividing platelet-derived particles into subpopulations (normal-sized platelets, small platelets, and platelet fragments). illustrates, using a whole-blood sample, how subpopulations normally appear upon strong platelet stimulation ().
Figure 1. Illustration of gating strategies to detect platelet subpopulations. The dot plots depict the formation of platelet subpopulations, exemplified by a whole blood sample from a healthy individual, before and after stimulation with same agonists as used for the platelet concentrates. a: Spontaneously activated platelets (with HEPES instead of agonist). b: Platelets activated with a combination of CRP-XL (1.33 µg/mL) + PAR1-AP (30 µm) + PAR4-AP (300 µm).
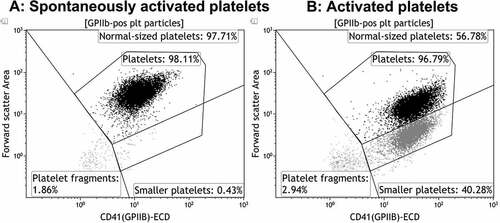
For each of the markers, a threshold gate of 1.5% positive platelets was set in the fluorescence histogram of each channel, using the background fluorescence control with dye corresponding to that channel. The background fluorescence sample with HEPES-EDTA was used to set the threshold positivity gate for PAC-1 as this requires an absolute calcium-free environment. The background fluorescence sample with HEPES was used to set the threshold positivity gates for P-selectin, LAMP-1, and Annexin V. The sample with CCCP was used to set the threshold positivity gate for DiIC1(5). Since small platelets and fragments are normally not present in the resting samples, all CD41+ events (i.e., normal-sized, small platelets and platelet fragments) were included when setting these background fluorescence threshold gates. These gates were then subsequently used to determine the binding of each marker on respective subpopulation. Binding of each marker was determined as the percentage of platelets positive for that marker.
To emphasize change upon platelet activation during storage, activation-induced response was calculated where the percentage for the non-agonist stimulated samples (i.e., with HEPES) was subtracted from the percentage of the agonist-stimulated samples.
Platelet aggregation measurements by impedance aggregometry
Platelet aggregation was measured by impedance technology (Multiplate, Roche Diagnostics, Basel, Switzerland). The platelet concentration of the sample was adjusted to 300 × 109/L by dilution with plasma from an AB Rh D+ donor (stored at −70ºC until use). To the test cuvette, 300 µL of pre-warmed 9 mg/mL NaCl (B. Braun, Melsungen, Germany) supplemented with 3.0 mM CaCl2 was added and 300 µL of diluted sample. After 3 min of incubation with constant stirring, 20 µL of platelet agonist (TRAP test, final concentration 32 µM and COLtest, final concentration 3.2 µg/mL, Roche Diagnostics) was added and the aggregation response followed for 6 min at 37ºC under constant stirring.
Coagulation and viscoelastic properties
Coagulation and viscoelastic properties were determined with a rheometer (ReoRox G2, MediRox AB, Nyköping, Sweden) as previously described [Citation10]. The platelet concentration of the sample was adjusted to 100 × 109/L by dilution with plasma from an AB Rh D+ donor. The plasma was kept at −80ºC until use. The diluted sample was recalcified and activated with PAR1-AP (SFLLRN; JPT Peptide Technologies, a final concentration of 0.09 mmol/L), and clotting time and maximum elasticity (G’max; final clot strength) determined by the rheometer.
Soluble markers of platelet activation
The release of the biologic response modifiers (BRMs) IFN-γ, TNF-α, IL-1β, IL-7, TGF-α, RANTES, VEGF, and sCD40 L were investigated using a custom human cytokine/chemokine made Milliplex magnetic bead panel (MILLIPLEX MAP, HCYT OMAG-60K, Merck Millipore, Danvers, MA) as previously described [Citation11,Citation12]. Soluble GPVI (sGPVI) was analyzed by enzyme-linked immunosorbent assay (ELISA) as previously described [Citation13].
Statistics
Friedman’s test with Dunn’s multiple comparison test for post-hoc comparisons was used for the assessment of storage-induced changes (GraphPad Prism 8, Software Inc., San Diego, CA). The Mann-Whitney U-test was used for comparisons of PCs with high and low fragmentation and Wilcoxon to assess differences between platelet subpopulations. Data are presented as median and interquartile ranges (25th–75th percentiles). A p value of <0.05 was considered statistically significant.
Results
The PCs contained a median of 344 × 109 (304–359) platelets/unit. Swirling was well maintained, and pH was above 7.0 at day 7 for all PCs. Glucose concentration decreased significantly from 4.8 (4.3–5.1) mM on day 1 to 2.8 (2.3–3.2) mM on day 7. Lactate concentration increased significantly from 4.9 (3.2–5.3) mM on day 1 to 9.2 (7.6–10.1) mM on day 7. The standard quality controls for PCs were met through the whole storage period for all units in the study.
Occurrence of platelet subpopulations
The occurrence of platelet subpopulations was assessed directly after preparation on day 1. As illustrated in , three subpopulations with different FSC/CD41 properties could be identified in some PCs without any activation. Gates were placed to separate “normal-sized platelets,” “small platelets”, and “platelet fragments” (we wanted to avoid the term “microparticles,” as this may have included both the last two subpopulations in earlier publications with less sensitive flow cytometers, as previously discussed in [Citation9]). As the division between normal-sized and smaller platelets was not as clear-cut in PCs as in whole blood, and that other brands of flow cytometers may not be able to distinguish these two populations, we also defined a gate called “Platelets,” that include both normal-sized and smaller platelets. Of the CD41+ events recorded by the flow cytometer, the PCs contained a median of 82% normal-sized platelets, 10% small and 8% fragments () after preparation. Neither the agonist nor the agonist combination induced an increase in the percentage of small or platelet fragments (data presented for the samples with HEPES and with the agonist combination in and ). Upon analysis of the collected data, we found that five of the PCs contained a large proportion of small platelets and/or platelet fragments (referred to as “PCs with high fragmentation”) that was significantly higher than the other five PCs (referred to as “PCs with low fragmentation”) (). No significant changes in metabolic variables were observed between these groups. No changes in subpopulations were observed with storage in PCs with low fragmentation, while the fractions of small platelets and fragments had decreased significantly at day 7 in PCs with high initial fragmentation [from 18 (13–27)/14 (10–27) to 10 (8–13)/8 (6–11) small platelets/fragments (median and 25th-75th percentile, p < .05)]. However, the fraction of small platelets and fragments were still significantly higher than for PCs with low fragmentation (p < .01).
Figure 2. Examples of flow cytometry dot plots from platelet units with high and low level of platelet fragmentation at day 1. a, c: Spontaneously activated (with HEPES instead of agonist). b, d: platelets activated with a combination of CRP-XL (1.33 µg/mL) + PAR1-AP (30 µm) + PAR4-AP (300 µm).
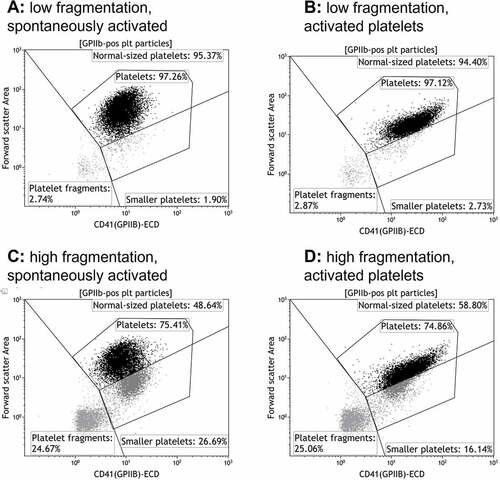
Table I. Platelet subpopulations in PCs on day 1 of storage.
Exposure patterns of activation markers
Following PC preparation, spontaneous PAC-1 binding and LAMP-1 exposure was generally low and this included all platelet subpopulations (). Annexin V binding was low on the platelet population as a whole but was considerably increased on small platelets and platelet fragments, as compared to normal-sized platelets. P-selectin expression on the spontaneously formed small platelets was significantly higher than on the normal-sized platelets. Most normal-sized platelets had DiIC1(5) fluorescence, but markedly fewer of the smaller platelets and almost none of the platelet fragments.
Table II. Expression of activation markers on all platelets and platelet subpopulations on day 1 of storage.
Upon agonist stimulation, normal-sized- and small platelets showed an increase in PAC-1, LAMP-1, and P-selectin, with the most pronounced changes being within the normal-sized platelets ( presents the data for the agonist combination). In contrast, the small platelets showed the most pronounced increase in Annexin V upon stimulation. They also showed a nearly abolished DiIC1(5), as compared to the more modest decrease seen among normal-sized platelets. In general, the platelet fragments responded poorly to agonist stimulation, even though some changes were statistically significant ().
Storage-induced changes in activation markers and platelet function of PCs with low and high fragmentation
As illustrated in , there were large differences in platelet fragmentation between the PCs after preparation. To assess the impact of this fragmentation on platelet activation and function, PCs with high platelet fragmentation (n = 5) were compared to those with low fragmentation (n = 5) longitudinally over storage. Impedance aggregometry showed that there was no difference in aggregation response to PAR1-AP (called TRAP in the commercial reagents) (). However, aggregation response to collagen decreased with storage and PCs with high fragmentation had lower response compared to those with low fragmentation (only statistically significant on day 5). Viscoelastic measurement of coagulation in response to PAR1-AP did not show any difference between PCs with low- and high fragmentation ().
Table III. Aggregation and coagulation response of platelets in PCs with high and low fragmentation.
As the results indicated that the platelet fragments responded poorly to activation (see ) and that the division between normal-sized and smaller platelets is not as clear-cut in PCs as in whole blood platelets (see vs. ), the storage-induced changes in platelet activation markers were investigated using the gate “Platelets” in , which include both the normal-sized and small platelets. High fragmentation in the PCs was associated with higher spontaneous Annexin V binding and lower DilC1(5) on all days compared to PCs with low fragmentation (). PCs with high fragmentation also had higher P-selectin expression on day 1 and PAC-1 binding and LAMP-1 exposure on day 7. An increase in P-selectin expression was reported during storage in PCs with low fragmentation, but the signal was already high on day 1.
Figure 3. Spontaneous exposure of platelet activation markers during storage in PCs with initially high (n = 5) and low (n = 5) platelet fragmentation. The result is for CD41+ events of normal- and small size. The bars show median values and error bars the interquartile ranges. */** = p <.05/0.01 compared to day 1 for low- and high fragmentation, respectively. #/## = p <.05/0.01 as compared to PCs with low fragmentation.
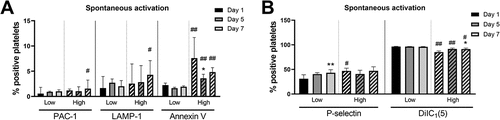
PCs with high fragmentation showed reduced capacity to respond to activation (). For PAC-1, responses were generally high, but the response to CRP-XL decreased to around 50% PAC-1 positive platelets in the PCs with high fragmentation toward the end of storage. P-selectin and LAMP-1, had a similar pattern with decreasing responses with storage for PCs with low fragmentation, while those with high fragmentation were less responsive and did not change with storage. The agonist-induced changes in Annexin V and DilC1(5) binding were generally low (). Since activation mainly altered those markers on small platelets (), this result corresponded with the finding that agonist stimulation did not induce an increase in small platelets.
Figure 4. Activation-induced response in PCs with initially high (n = 5) and low (n = 5) platelet fragmentation. The result is for CD41+ events of normal- and small size. The bars show median values and error bars the interquartile ranges. */** = p <.05/0.01 compared to day 1 for low- and high fragmentation, respectively. # = p <.05 as compared to PCs with low fragmentation. The activation-induced response was calculated where the percentage for the non-agonist stimulated samples (i.e., with HEPES) was subtracted from the percentage of the agonist-stimulated samples.
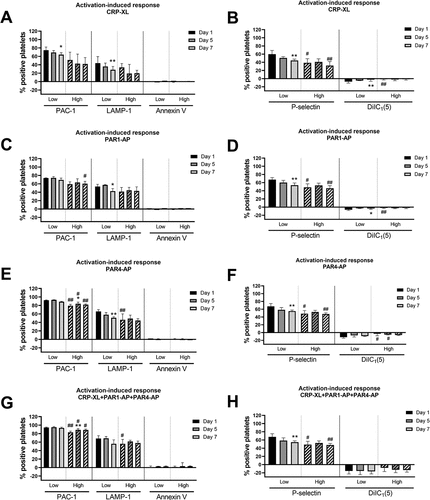
Storage increased the concentration of all investigated BRMs significantly and to a similar extent in PCs with high- and low fragmentation (Supplementary Table S1). The concentration of sGPVI had also increased by day 7, but only in the PCs with low fragmentation. The PCs with high fragmentation had a high level of sGPVI (p = .056 compared to PCs with low fragmentation) already at day 1, which did not increase further during storage (Supplementary Table S1).
Discussion
The results of our study demonstrate that small platelets and fragments represented around 20% of CD41+ events in whole blood derived PCs after preparation, even in the non-agonist stimulated samples. This is much larger than what we previously reported for PCs prepared by the apheresis technique, which contained in total <5% small platelets and fragments following preparation [Citation8]. A study on blood samples showed they contained on average <1% small platelets and 1% platelet fragments [Citation9]. Normally, strong activation is necessary for subpopulation formation [Citation1–3,Citation9]. The study on apheresis PCs also showed that activation with CRP-XL, alone or combined with other agonists, increased the proportion of small platelets and fragments [Citation8], which was not found in this study. Plausible explanations for these variations are the difference in preparation technique (centrifugation variations, thus impacting which platelets that are lost in that process [Citation14]), or the use of different storage media (plasma in the apheresis PCs and platelet additive solution in the whole blood derived PCs). These factors are known to affect platelet activation and function [Citation15–17]. Donor variations could also have affected the results despite pooling. Marcoux et al. [Citation18] and Rank et al. [Citation19] also found that platelet microparticle release occurred early after platelet preparation. Marcoux et al. also showed that this was affected by the PC preparation method [Citation18]. Furthermore, this platelet subpopulation formation was not homogenous. Five PCs were found to contain a significantly higher proportion of CD41+ small platelets and platelet fragments (median of 28% small plus fragments), while the other five PCs had a more similar pattern to whole blood with low spontaneous fragmentation (median of 9% small plus fragments).
The normal-sized platelets had a different exposure pattern than the small platelets and platelet fragments. All normal-sized platelets bound PAC-1 upon agonist stimulation, thus exhibiting proaggregatory features [Citation2]. In addition, they had DiIC1(5) fluorescence, indicating retained mitochondrial membrane potential, in contrast to the small platelets and fragments. The small platelets exhibited procoagulant hallmarks, i.e., P-selectin and PS exposure and affected mitochondria [Citation1]. Our results confirmed our previous findings that PS is mainly expressed on small platelets and platelet fragments [Citation8]. Keuren et al. also found PS expressing microparticles in whole blood derived PCs [Citation20]. Our small platelets are likely the procoagulant platelets described with a multitude of different names in previous publications (reviewed in [Citation1]). The PS-positive procoagulant small platelets and fragments may have formed either due to activation of platelets during the preparation process or from aging apoptotic platelets [Citation4,Citation21,Citation22]. It has been previously suggested that microparticles expressing PS and P-selectin originate from activated platelets [Citation19,Citation23], whereas microparticles from aging platelets express activated GPIIb/IIIa [Citation23]. This indicates that our small platelets and fragments are a result of spontaneous platelet activation rather than apoptosis.
The reduction in PAC-1 binding found on the PS-positive platelets has been reported previously and was described as a down-regulation of the fibrinogen receptor [Citation24]. The small platelets still retained some intact mitochondria (DilC1(5) binding) and an ability to respond to activation by exhibiting procoagulant features in terms of increased PS- and P-selectin exposure [Citation22]. The platelet fragments responded poorly to activation, most likely because they are only parts of an intact platelet. Marcoux et al. [Citation18] identified two microparticle populations in PCs, one with higher FSC with intact mitochondria and one with lower FSC lacking intact mitochondria. Possibly their two microparticle populations represent what we refer to as small and platelet fragments, respectively. However, comparisons of procoagulant platelet formation and microparticle formation between studies are complicated because of different methodologies. These differences include varying gating strategies to identify these populations. For example, some studies include the expression of PS as a marker to identify microparticles [Citation19], others used beads as size discriminators [Citation18,Citation25]. Some quantify the content and present it as concentration [Citation18,Citation19], whereas others, in similarity to us, report the results as a percentage [Citation25]. If flow cytometry is used to detect fragments, the possibility to discriminate between small platelets and platelet fragments might vary. However, regardless of the method used to detect fragmented platelets, it should be possible to connect the findings to reduced activation potential.
Storage resulted in platelet activation in the form of release of BRMs from granules as previously reported [Citation11], which could be interpreted as a measure of the platelet secretory capacity [Citation4]. It needs to be mentioned that the centrifugation settings used here are not sufficient to remove platelet fragments from the supernatants, which means that the analyzed supernatants also include platelet fragments. However, high platelet fragmentation did not seem to have impacted the results, as we found no difference in the release of the BRMs in the PCs with high fragmentation compared to those with low fragmentation.
Only PCs with low fragmentation had a spontaneous increase in P-selectin exposure during storage. PCs with high fragmentation had a higher P-selectin exposure already from the start, and this was not further increased by storage. It has been shown that P-selectin expression can decrease initially during storage (most likely due to cleavage from the surface) before an increase occurs [Citation17], and this might in part account for the modest changes seen during storage. Even though PCs with high fragmentation had higher spontaneous LAMP-1 and PAC-1 binding at the end of storage, the percentage remained low (<10%). Spontaneous Annexin V binding was not increased during storage. This finding is in line with other studies who have reported none- or only small increase within 7 days of storage [Citation8,Citation25–27]. Higher PS exposure might occur when storage is prolonged for more than 7 days [Citation8,Citation25–28]. Here, we found that some PCs contained a high proportion of platelets with the procoagulant phenotype already on the first day of storage.
The PCs containing a high proportion of fragmented platelets had a generally reduced capacity to respond to different agonists. This might be attributed to impaired mitochondrial integrity [Citation29], as DilC1(5) binding was reduced, impairing oxidative phosphorylation [Citation30] and resulting in reduced ATP synthesis [Citation29]. ATP production is crucial for the preservation of platelet function [Citation4]. There is a correlation between mitochondrial function and glycolysis [Citation25]. Thus, during hypoxic conditions, more glucose might be needed to preserve platelet function [Citation4,Citation31]. However, we did not find any difference in glycolysis, and thus no immediate effect of reduced mitochondria function in the PCs with high fragmentation was evident within the investigated storage period. As the division between normal-sized and smaller platelets was not as clear-cut in PCs as in whole blood and that other brands of flow cytometers may not be able to distinguish these two populations, we chose to analyze these two populations together when investigating activation-induced changes. Thus, a partial explanation to the results is the larger fraction of smaller platelets with a differing activation pattern in the PCs with high fragmentation.
Neither aggregometry nor flow cytometry indicated that PC storage had a major impact on the integrity of the GPIIb/IIIa receptors and their ability to transform into the active conformation, as responses to agonist stimulation resulted in generally high PAC-1 binding and aggregation response to TRAP was unaffected. Furthermore, storage did not contribute to any major change in clot strength (elasticity), which is also dependent on functional GPIIb/IIIa receptors. The aggregation capacity was not likely to have been affected by spontaneous activation of GPIIb/IIIa, as PAC-1 binding remained at <5% in the non-agonist stimulated samples throughout storage [Citation4]. Collagen-induced aggregation responses decreased with storage, pointing to changes in the integrity of the collagen receptors or its signaling mechanisms. Flow cytometry results also point to an affected response to collagen (CRP-XL), resulting in only about 50% PAC-1 binding platelets in the PCs with high fragmentation at the end of the storage period. Procoagulant platelet formation is dependent on the activation of GPVI [Citation1], and the poor activation response to collagen is in line with the lack of subpopulation formation and the absent increase in PS exposure after stimulation with collagen [Citation22].
Our findings point to a connection between aggregation responses to collagen and the level of sGPVI as described previously [Citation32]. PCs with high fragmentation had lower aggregation response to collagen compared to PCs with low fragmentation during the first 5 days. PCs with high fragmentation had a high level of sGPVI already on day 1 (p = .056 compared to PCs with low fragmentation) which did not change with storage. In contrast, PCs with low fragmentation had an increase in sGPVI during storage. This finding is similar to another study reporting shedding of GPVI during storage [Citation33] and is also linked to mitochondrial damage [Citation32]. In line with other studies of PCs using impedance aggregometry [Citation34–37], the aggregation responses to collagen, but not TRAP, was very low already on day 1 as compared to the normal ranges for blood.
Each PC contained platelets from five blood donors, and whether all platelet fragments originate from one donor’s platelets or come from platelets from all five donors is unknown. The PCs in this study were prepared on four separate occasions over approximately 1 month. There does not seem to have been any systematic bias regarding the order in which the PCs with high fragmentation occurred and there were days when both PCs with high- and low fragmentation had been prepared. The PCs were not selected for specific properties, and additional studies are needed to establish the frequency of PCs with high fragmentation with different preparation protocols, and whether this has any impact on transfusion efficiency. Additional tests such as thrombin generation [Citation38] could also be considered to further characterize the properties of PCs with different degrees of fragmentation. Since small platelets and fragments are not normally present in resting samples, all CD41+ events (i.e., normal-sized, small platelets and platelet fragments) were included when setting the background fluorescence threshold gates. These gates were then subsequently used to determine the binding of each marker on respective subpopulation. The consequence of using this approach is a minimal underestimation of the binding of markers on small platelets and platelet fragments.
In conclusion, PCs containing a high proportion of fragmented platelets had reduced activation capacity, increased GPVI receptor shedding, and affected mitochondria. A recent review suggested that procoagulant platelets and their high number in some PCs might be beneficial for patients with acute bleeding due to their procoagulant features, partly compensating for reduced adhesive and aggregating functions [Citation4]. Our findings also show that they still retained some activation potential throughout 7 days of storage. Mitochondrial damage has been shown to be associated with GPIb receptor shedding and platelet clearance, but the mechanisms are unclear [Citation39]. In vivo studies are thus needed to determine if high platelet fragmentation impacts platelet transfusion clearance.
Supplemental Material
Download PDF (155.3 KB)Acknowledgements
We would like to thank Therese Enenge and Eimear Dunne for their assistance with GPVI analysis. This study was supported by grants from Region Östergötland.
Disclosure statement
No potential conflict of interest was reported by the authors.
Data availability statement
The data that support the findings of this study are available from the corresponding author upon reasonable request.
Supplementary material
Supplemental data for this article can be accessed online at https://doi.org/10.1080/09537104.2022.2159018
Additional information
Funding
References
- Agbani EO, Poole AW. Procoagulant platelets: generation, function, and therapeutic targeting in thrombosis. Blood. 2017;130(20):2171–10. Epub 2017 10 04. doi:10.1182/blood-2017-05-787259.
- Heemskerk JW, Mattheij NJ, Cosemans JM. Platelet-based coagulation: different populations, different functions. J Thromb Haemost. 2013;11(1):2–16. Epub 2012 10 31. doi:10.1111/jth.12045.
- Mazepa M, Hoffman M, Monroe D. Superactivated platelets: thrombus regulators, thrombin generators, and potential clinical targets. Arterioscler Thromb Vasc Biol. 2013;33(8):1747–1752. Epub 2013 07 19. doi:10.1161/ATVBAHA.113.301790.
- Escolar G, McCullough J. Platelet in vitro assays: their correspondence with their in vivo hemostatic potential. Transfusion. 2019;59(12):3783–3793. Epub 2019 10 24. doi:10.1111/trf.15559.
- Ng MSY, Tung JP, Fraser JF. Platelet storage lesions: what more do we know now? Transfus Med Rev. 2018;32(3):144–154. Epub 2018 05 13. doi:10.1016/j.tmrv.2018.04.001.
- Shattil SJ, Hoxie JA, Cunningham M, Brass LF. Changes in the platelet membrane glycoprotein IIb.IIIa complex during platelet activation. J Biol Chem. 1985;260(20):11107–11114. doi:10.1016/S0021-9258(17)39154-8.
- Remenyi G, Szasz R, Friese P, Dale GL. Role of mitochondrial permeability transition pore in coated-platelet formation. Arterioscler Thromb Vasc Biol. 2005;25(2):467–471. Epub 2004 12 14. doi:10.1161/01.ATV.0000152726.49229.bf.
- Sodergren AL, Tynngard N, Berlin G, Ramstrom S. Responsiveness of platelets during storage studied with flow cytometry–formation of platelet subpopulations and LAMP-1 as new markers for the platelet storage lesion. Vox Sang. 2016;110(2):116–125. Epub 2015 09 22. doi:10.1111/vox.12324.
- Sodergren AL, Ramstrom S. Platelet subpopulations remain despite strong dual agonist stimulation and can be characterised using a novel six-colour flow cytometry protocol. Sci Rep. 2018;8(1):1441. Epub 2018 01 25. doi:10.1038/s41598-017-19126-8.
- Tynngard N, Trinks M, Berlin G. In vitro function of platelets treated with ultraviolet C light for pathogen inactivation: a comparative study with nonirradiated and gamma-irradiated platelets. Transfusion. 2015;55(6):1169–1177. doi:10.1111/trf.12963.
- Sandgren P, Berlin G, Tynngard N. Treatment of platelet concentrates with ultraviolet C light for pathogen reduction increases cytokine accumulation. Transfusion. 2016;56(6):1377–1383. Epub 2016 04 16. doi:10.1111/trf.13601.
- Tynngard N, Wikman A, Uhlin M, Sandgren P. Haemostatic responsiveness and release of biological response modifiers following cryopreservation of platelets treated with amotosalen and ultraviolet a light. Blood Transfus. 2020;18(3):191–199. Epub 2019 08 14. doi:10.2450/2019.0061-19.
- Egan K, Dillon A, Dunne E, Kevane B, Galvin Z, Maguire P, Kenny D, Stewart S, Ainle FN. Increased soluble GPVI levels in cirrhosis: evidence for early in vivo platelet activation. J Thromb Thrombolysis. 2017;43(1):54–59. Epub 2016 07 16. doi:10.1007/s11239-016-1401-0.
- Handtke S, Steil L, Greinacher A, Thiele T. Toward the relevance of platelet subpopulations for transfusion medicine. Front Med. 2018;5:17. Epub 2018 02 21. doi:10.3389/fmed.2018.00017.
- Saunders C, Rowe G, Wilkins K, Holme S, Collins P. In vitro storage characteristics of platelet concentrates suspended in 70% SSP+(TM) additive solution versus plasma over a 14-day storage period. Vox Sang. 2011;101(2):112–121. Epub 2011 03 19. doi:10.1111/j.1423-0410.2011.01468.x.
- Krailadsiri P, Seghatchian J. Are all leucodepleted platelet concentrates equivalent? Comparison of cobe LRS turbo, haemonetics MCS+ LD, and filtered pooled buffy-coat-derived platelets. Vox Sang. 2000;78(3):171–175. doi:10.1046/j.1423-0410.2000.7830171.x.
- Zhang JG, Carter CJ, Culibrk B, Devine DV, Levin E, Scammell K, Weiss S, Gyongyossy-Issa MI. Buffy-coat platelet variables and metabolism during storage in additive solutions or plasma. Transfusion. 2008;48(5):847–856. doi:10.1111/j.1537-2995.2008.01645.x.
- Marcoux G, Duchez AC, Rousseau M, Levesque T, Boudreau LH, Thibault L, Boilard E. Microparticle and mitochondrial release during extended storage of different types of platelet concentrates. Platelets. 2017;28(3):272–280. doi:10.1080/09537104.2016.1218455.
- Rank A, Nieuwland R, Liebhardt S, Iberer M, Grutzner S, Toth B, Pihusch R. Apheresis platelet concentrates contain platelet-derived and endothelial cell-derived microparticles. Vox Sang. 2011;100(2):179–186. Epub 2010 08 13. doi:10.1111/j.1423-0410.2010.01385.x.
- Keuren JF, Magdeleyns EJ, Govers-Riemslag JW, Lindhout T, Curvers J. Effects of storage-induced platelet microparticles on the initiation and propagation phase of blood coagulation. Br J Haematol. 2006;134(3):307–313. doi:10.1111/j.1365-2141.2006.06167.x.
- Schoenwaelder SM, Yuan Y, Josefsson EC, White MJ, Yao Y, Mason KD, O’Reilly LA, Henley KJ, Ono A, Hsiao S, et al. Two distinct pathways regulate platelet phosphatidylserine exposure and procoagulant function. Blood. 2009;114(3):663–666. Epub 2009 04 24. doi:10.1182/blood-2009-01-200345.
- Tohidi-Esfahani I, Lee CSM, Liang HPH, Chen VMY. Procoagulant platelets: laboratory detection and clinical significance. Int J Lab Hematol. 2020;42(Suppl 1):59–67. Epub 2020 06 17. doi:10.1111/ijlh.13197.
- Vasina EM, Cauwenberghs S, Staudt M, Feijge MA, Weber C, Koenen RR, Heemskerk JW. Aging- and activation-induced platelet microparticles suppress apoptosis in monocytic cells and differentially signal to proinflammatory mediator release.Am J Blood Res. 2013;3(2):107–123. Epub 2013 05 16.
- Munnix IC, Kuijpers MJ, Auger J, Thomassen CM, Panizzi P, van Zandvoort MA, Rosing J, Bock PE, Watson SP, Heemskerk JW. Segregation of platelet aggregatory and procoagulant microdomains in thrombus formation: regulation by transient integrin activation. Arterioscler Thromb Vasc Biol. 2007;27(11):2484–2490. Epub 2007 09 01. doi:10.1161/ATVBAHA.107.151100.
- Cookson P, Sutherland J, Turner C, Bashir S, Wiltshire M, Hancock V, Smith K, Cardigan R. Platelet apoptosis and activation in platelet concentrates stored for up to 12 days in plasma or additive solution. Transfus Med. 2010;20(6):392–402. Epub 2010 08 27. doi:10.1111/j.1365-3148.2010.01034.x.
- Leytin V, Allen DJ, Mutlu A, Mykhaylov S, Lyubimov E, Freedman J. Platelet activation and apoptosis are different phenomena: evidence from the sequential dynamics and the magnitude of responses during platelet storage. Br J Haematol. 2008;142(3):494–497. Epub 2008 05 22. doi:10.1111/j.1365-2141.2008.07209.x.
- Ostrowski SR, Bochsen L, Salado-Jimena JA, Ullum H, Reynaerts I, Goodrich RP, Johansson PI. In vitro cell quality of buffy coat platelets in additive solution treated with pathogen reduction technology. Transfusion. 2010;50(10):2210–2219. Epub 2010 05 12. doi:10.1111/j.1537-2995.2010.02681.x.
- Cardigan R, Sutherland J, Garwood M, Bashir S, Turner C, Smith K, Hancock V, Wiltshire M, Pergande C, Williamson LM. In vitro function of buffy coat-derived platelet concentrates stored for 9 days in CompoSol, PASII or 100% plasma in three different storage bags. Vox Sang. 2008;94(2):103–112. doi:10.1111/j.1423-0410.2007.01008.x.
- Perales Villarroel JP, Figueredo R, Guan Y, Tomaiuolo M, Karamercan MA, Welsh J, Selak MA, Becker LB, Sims C. Increased platelet storage time is associated with mitochondrial dysfunction and impaired platelet function. J Surg Res. 2013;184(1):422–429. Epub 2013 07 09. doi:10.1016/j.jss.2013.05.097.
- Picker SM, Schneider V, Oustianskaia L, Gathof BS. Cell viability during platelet storage in correlation to cellular metabolism after different pathogen reduction technologies. Transfusion. 2009;49(11):2311–2318. Epub 2009 07 25. doi:10.1111/j.1537-2995.2009.02316.x.
- Verhoeven AJ, Verhaar R, Gouwerok EG, de Korte D. The mitochondrial membrane potential in human platelets: a sensitive parameter for platelet quality. Transfusion. 2005;45(1):82–89. doi:10.1111/j.1537-2995.2005.04023.x.
- Hosseini E, Mohtashami M, Ghasemzadeh M. Down-regulation of platelet adhesion receptors is a controlling mechanism of thrombosis, while also affecting post-transfusion efficacy of stored platelets. Thromb J. 2019;17(1):20. Epub 2019 10 30. doi:10.1186/s12959-019-0209-5.
- Hosseini E, Ghasemzadeh M, Nassaji F, Jamaat ZP. GPVI modulation during platelet activation and storage: its expression levels and ectodomain shedding compared to markers of platelet storage lesion. Platelets. 2017;28(5):498–508. Epub 2016 10 26. doi:10.1080/09537104.2016.1235692.
- Glas M, Bauer JV, Eichler H, Volk T. Impedance aggregometric analysis of platelet function of apheresis platelet concentrates as a function of storage time. Scand J Clin Lab Invest. 2016;76(8):664–670. Epub 2016 10 06. doi:10.1080/00365513.2016.1238505.
- Ostrowski SR, Bochsen L, Windelov NA, Salado-Jimena JA, Reynaerts I, Goodrich RP, Johansson PI. Hemostatic function of buffy coat platelets in additive solution treated with pathogen reduction technology. Transfusion. 2011;51(2):344–356. Epub 2010 08 21. doi:10.1111/j.1537-2995.2010.02821.x.
- Shams Hakimi C, Hesse C, Wallen H, Boulund F, Grahn A, Jeppsson A. In vitro assessment of platelet concentrates with multiple electrode aggregometry. Platelets. 2015;26(2):132–137. doi:10.3109/09537104.2014.898141.
- Singh S, Shams Hakimi C, Jeppsson A, Hesse C. Platelet storage lesion in interim platelet unit concentrates: a comparison with buffy-coat and apheresis concentrates. Transfus Apher Sci. 2017;56(6):870–874. doi:10.1016/j.transci.2017.10.004.
- Tohidi-Esfahani I, Tan S, Tan CW, Johnson L, Marks DC, Chen VM. Platelet procoagulant potential is reduced in platelet concentrates ex vivo but appears restored following transfusion. Transfusion. 2021;61(12):3420–3431. Epub 2021 10 05. doi:10.1111/trf.16695.
- Quach ME. Gpib-IX-V and platelet clearance. Platelets. 2022;33(6):817–822. Epub 2021 06 24. doi:10.1080/09537104.2021.1942815.