Abstract
Platelet Rich Plasma (PRP) is a biological treatment which, thanks to its enhanced growth factors content, is widely used in the field of regenerative medicine for its reparative effects. Although it is usually used fresh immediately after preparation, its freezing for preservation for future usage could be key in increasing its versatility and new applications. To assess the suitability of freezing, after collecting PRP and platelet lysates (PL) from 6 patients, they were preserved for 1 or 3 months at temperatures of −20ºC and −80°C. Measurements were then made on platelet number and integrity, growth factor levels, biomechanical properties of the clot and its bioactivity on cultured cells. Fresh PRP and PL were used as controls. The results showed an increase in platelet size (p < .01) and clot elasticity (p < .01), as well as decrease in levels of PDGF (P < .05) and VEGF (p < .05), though the overall bioactivity was not affected as culture cells showed the same responsiveness to both frozen and fresh PRP and PL in terms of cell viability. Based on these results, it could be assumed that preservation of PRP by freezing is a feasible and suitable option for its further use.
Plain Language Summary
What is the context?
Platelet-Rich Plasma (PRP) is a biological treatment widely used in regenerative medicine as a result of its high content of growth factors.
In its routine use, PRP has autologous character, since it is obtained from the same patient and its infiltration in the affected area takes place immediately after it is obtained.
Its storability would give PRP versatility in use, which could enable a potential allogeneic use and even significantly reduce the number of blood draws for each patient.
It is necessary to establish a PRP storage protocol where its properties are not affected.
What is new?
PRP was stored at two time points (1 and 3 months) and temperatures (−20ºC and −80ºC) in activated and unactivated states. Afterwards, platelet number, size and activation were measured. Furthermore, the biomechanical properties of the resulting clot, the growth factor content and PRP’s impact on cell viability were analyzed.
The limiting factor was not having used aggregometry or other techniques that measure other cellular processes, as well as the limited sample size.
The results showed that freezing affected platelet size, the levels of platelet-derived GFs and the biomechanical properties of the clot. However, plasmatic levels of growth factors or its capacity to boost cellular proliferation were not affected.
What is the impact?
The clinical impact of this work is the ability to preserve PRP by freezing. This is especially relevant as it allows a possible use of PRP as an allogeneic treatment. Moreover, its preservation significantly reduces the number of blood draws for each patient, especially in those with puncture difficulties or with apprehension.
Introduction
Platelet-Rich Plasma (PRP) is a biological treatment frequently used in the field of regenerative medicine due to its restorative, anti-inflammatory and analgesic properties.Citation1 It is a platelet concentrate usually obtained by the centrifugation of the patient’s own blood. The platelet enrichment allows the release of multiple growth factors stored in platelets, such as Transforming Growth Factor beta (TGF-β), Vascular Endothelial Growth Factor (VEGF) and Platelet-derived Growth Factor (PDGF) among others, when the platelet activation and coagulation cascade is stimulated.Citation2,Citation3 These molecules released from platelets, together with other plasmatic ones such as Insulin-like Growth Factor 1 (IGF-1) or Hepatocyte Growth Factor (HGF), have been found to promote different cellular processes such as proliferation, migration, angiogenesis, inhibition of apoptosis and also play a part in modulating inflammation and fibrosis.Citation4,Citation5 For this reason, PRP is widely used in different fields of the medicine such as orthopedics, dentistry and dermatology, among others.Citation4 With the use of PRP in all these fields, tissue repair and stimulation of regeneration is sought to treat the pathology. For this, it is vital that the biomolecular content of the PRP maintains its bioactivity on the target tissue. In these areas PRP is usually employed, instead of platelet lysate (PL), as it generates a fibrin matrix that allows the gradual release of growth factors as it degrades.Citation6
In routine clinical practice PRP has an autologous nature and the whole obtention process is usually conducted immediately before its injection into the affected area. This implies that the blood is collected and processed during the patients visit for each infiltration session. This aspect, however, has a number of limitations. First, the patient’s waiting time can be prolonged as the whole process, from blood extraction to the infiltration, can be extended in time. Second, the fact that the blood is extracted immediately before its processing would limit a potential allogeneic use, since the patient and donor(s) would have to be scheduled to come along to the medical center the same day and time. Finally, this aspect could also be limiting in the case of patients who present medical difficulties or apprehension at the time of puncture for blood collection, due to the need to draw blood at every infiltration visit. Considering these limitations, PRP preservation could be a solution that solves these problems and might open new doors to new sample processing protocols that better adapt to the needs of each patient.
In recent years, several studies have been carried out to analyze different PRP preservation conditions. These studies revealed that room temperature was only valid for the preservation of PRP for a maximum of one week and would not be valid for longer periods.Citation7–9 Meanwhile, freeze-drying facilitates the optimal preservation of platelets and growth factors for long periods of time in vitroCitation10 and in vivo.Citation11 However, freeze-drying involves the use of specific equipment and higher costs. Finally, there is the possibility of freezing PRP, which is simpler, cheaper, and more feasible in clinical practice. Overall, the studies performed comparing fresh and frozen PRP are short-term focused and showed that, although the platelet and plasma growth factor profile may vary, bioactivity remains at similar levels in both groups in in vitroCitation12,Citation13 and in vivoCitation14 research works. Therefore, no long-term studies have been performed, except for one 6-month study with equine PRP, where it was reported that PRP can be frozen at −80ºC for 1 month to keep the concentrations of TGF-B and IGF-1 intact.Citation15
Considering that there is no firm consensus on the best preservation method, it is necessary to set a preservation protocol where PRP is not affected by the freezing in order to maintain its regenerative properties. Therefore, the purpose of this study is to analyze the quality of the platelets, their activation and the clot formation, as well as the growth factors present in the PRP after different freezing times and temperatures. Finally, the bioactivity on the growth of cell cultures was assessed.
Materials and methods
Platelet-rich plasma preparation
Blood was collected from 6 healthy individuals (3 females/3 males) with ages ranging from 26 to 43. Informed consent for sample donation was obtained from each of the donors from whom blood samples were collected. Ethical approval for this study (Protocol No. UCA-13/EE/21/CON) was obtained from the Ethics Committee of OSI Araba.
PRP and PL were obtained in three different time points. The first extraction was performed three months before the analysis, the second one one month before and the third one on the same day of the analysis to be used as a fresh control.
PRP was obtained after blood centrifugation at 580 × g for 8 min at room temperature into 3.8% (wt/v) sodium citrate containing 9 mL tubes. The 2 mL plasma column located above the sedimented red blood cells, but not including the buffy coat, was collected in a tube. This processing produces a PRP with a platelet concentration of 1.5-3× the concentration of platelets relative to baseline blood levels and an absence of erythrocytes and leukocytes. Half of the PRP volume of each individual was activated by adding CaCl2 (10% wt/vol), and once the clot was generated, it was squeezed and discarded to obtain the PRP lysate. The other half of the volume was stored unactivated to test whether it is more appropriate to store platelet lysates or unactivated PRP ().
Figure 1. Schematic layout of the methodological protocol. PRP was obtained by centrifugation at 580 x g for 8 minutes. The lower half of the plasma layer was transferred to a new tube to obtain PRP. One part was directly preserved at either −20ºC or −80ºC for 1 or 3 months. The other part was first activated by CaCl2 addition and once the clot was formed, the supernatant, also called platelet lysate (PL), was preserved under the same conditions. Finally, frozen PRP and PL were characterized and in vitro evaluated using fresh samples as a control.
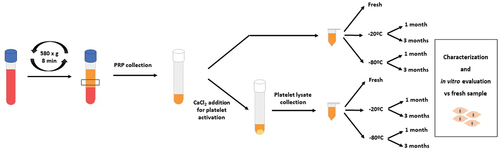
Platelet-rich plasma storage
In order to compare the effect of the temperature on PRP characteristics and bioactivity, unactivated PRP and platelet lysate samples were divided into two batches to be stored at −20°C and −80°C. The PRP volume was stored in aliquots to avoid freezing and thawing cycles. Part of the samples were thawed for analysis after 1 month of storage and the other part after 3 months. Prior to growth factors quantification and cell viability analyses, unactivated stored PRP samples were activated by adding CaCl2 (10% wt/vol), and once the clot was generated, it was squeezed and discarded to obtain the PRP lysate. The samples were compared with fresh samples from the same donors obtained on the same day of analysis ().
Platelet-rich plasma characterization
The platelet content of the PRP was measured on the Sysmex XS-1000i hematological analyzer (Sysmex, Kobe, Japan). Platelet number and mean platelet volume (MPV) were analyzed. In accordance with minimum reporting requirements for PRP studies, the PRP type was characterized according to the UCS (universal coding system).Citation16
Platelet activation analysis
Platelet activation was assessed by platelet labeling (CD41) and activated platelet labeling (CD62) by flow cytometry. For each donor, 10 μL of plasma sample, 5 μL of anti-CD41-FITC, and 5 μL of anti-CD62P-PE (BD Biosciences, San Jose, CA, USA) were added in the test tubes. The remaining volume up to 100 μL was completed with PBS. The appropriate controls for flow cytometric technique were used. Finally, the samples were incubated for 15 minutes at room temperature in the dark and then were fixed with 400 μL of freshly prepared 1.25% formaldehyde in PBS. A Gallios flow cytometer (Beckman-Coulter, High Wycombe, UK) was used to analyze events.
Fibrinogen levels measurement
Fibrinogen levels in PRP were measured using the coagulation analyzer (STA Compact Max, Stago, France). Enzyme-Linked Immunosorbent Assay (ELISA)
In order to determine the effect of storage temperature on the composition of thawed PRP, several growth factors were measured by ELISA kits (Bio-techne; Minneapolis, MN, USA) in duplicate. In order to analyze whether fresh and frozen PRP samples had similar content, the main growth factors were measured using the following ELISA kits: Hepatocyte Growth Factor (HGF) (156–10,000 pg/mL), Insulin-Like Growth Factor 1 (IGF-1) (94–600 ng/mL), Platelet-Derived Growth Factor (PDGF) (780–50,000 pg/mL), Transforming Growth Factor Beta (TGF-β) (1284–80,000 pg/mL), and Vascular Endothelial Growth Factor (VEGF) (31. 3–2000 pg/mL). All the protocols were conducted following manufacturer’s instructions. Growth factor levels were measured by absorbance, and concentrations were extrapolated by the corresponding calibration curve.
Cell cultures and culture media
Normal human lung fibroblasts (CC-2512; Lonza, Basel, Switzerland) were employed for the in vitro evaluation of PRP samples frozen at different temperatures. They were kept in the incubator at 37°C and 5% CO2. Cells were grown in fibroblast growth basal medium (CC-3131; Lonza, Basel, Switzerland) supplemented with insulin, human fibroblast growth factor, and gentamicin sulfate-amphotericin at 0.1% (v/v) each (CC-4126; Lonza, Basel, Switzerland), as recommended by the manufacturer. Additionally, PRP that had been frozen at −20°C or −80°C was added to the media at a concentration of 10%. For the negative control, no medium was added.
Cell viability assay
To assess whether the bioactivity of PRPs could be affected by storage temperature, the growth curves of dermal cells incubated with medium supplemented with PRP stored at different temperatures were performed in triplicate with each of the pools. Cell viability was recorded over 96 hours by Realtime-Glo MT Cell Viability Assay (G9711; Promega, Fitchburg, WI, USA) that bases on the reducing potential of metabolically active cells that catalyze the conversion of a synthetic substrate into a luminescent product. The levels of luminescence that the detector reached can be considered proportional to the number of viable cells present in the assay. Then, the obtained growth curves with the different PRP formulations were compared to assess whether the cryopreservation affects the bioactivity of PRP.
Mechanical tests on fibrin clots
As mentioned above, to carry out the clot formation CaCl2 is added and left for half an hour. To determine the mechanical properties of the fibrin clots, instrumented indentation tests were carried out using a spherical indenter. In these tests, a calibrated spherical indenter with a diameter of 5 mm was pressed onto the sample to be studied and the load used to penetrate the indenter as well as the penetration distance at each moment were recorded. The indentations were performed directly on 24-well plates where the different fibrin clots were produced in situ after PRP activation. The sphere penetrated the sample at a constant speed of 50 μm/s until it reached a depth equivalent to 20% of the initial thickness of the sample. Once this penetration was reached, the direction of movement was reversed, discharging the indenter. Young’s modulus and dissipated energy were analyzed. This discharge was carried out at a constant speed of 100 μm/s. A Zwick/Roell ZwickiLine Z1.0 uniaxial testing machine (Ulm, Germany) was used for these tests. The load cell used was a Zwick/Roell Xforce P with a maximum load of 50 N.
Statistical analysis
Comparisons were performed by analysis of variance (ANOVA) and Student’s t-tests. The normal distribution of samples was assessed by the Shapiro–Wilk test. In case that the data did not fit the normal distribution, nonparametric Kruskal–Wallis one-way ANOVA, Wilcoxon signed-rank test for paired non-parametric data and Mann–Whitney U test for independent non-parametric data were applied and data were considered statistically significant when the p-values were less than 0.05. Statistical analysis was performed with PASW Statistics 20.0 (SPSS®, Chicago, IL, USA) and GraphPad Prism (San Diego, CA, USA).
Results
Platelet-rich plasma characterization
The PRP used for this study had a mean platelet concentration of 470 ± 136 × 103/µL and a higher platelet concentration than that of blood with an average ratio of 2.7-fold. It had no erythrocytes or leukocytes and CaCl2 was used for activation (). In accordance with the UCS (universal coding system) and minimum reporting requirements for PRP studies, the PRP type was 14-00-11.Citation16
Table I. Summary of PRP characteristics.
Freezing effect on platelets
The results of the hematological analyzer measurements showed no difference in platelet number between fresh and frozen samples (). However, samples frozen at −20°C showed a significantly larger size in both those stored at −20°C for one month (p < .0001) and those stored for 3 months (p < .01) (). Mean platelet volume (MPV) was used as an indicative of platelet size.
Figure 2. Platelet concentration, size and activation after freezing preservation. Mean values of platelet concentration (A), size (B) and activation (C) in the PRP and PL according to different storage temperatures (−20/−80ºC) and time (Fresh, 1 month, 3 months). The platelet activation graph represents the CD62 and CD41 positive cells, relative to the fresh control. Error bars = standard deviation.
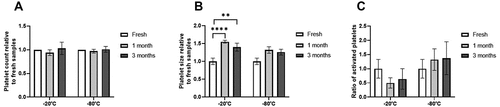
The results showed no significant difference in platelet activation between frozen and fresh samples when CD41 and CD62 expressions were analyzed ().
Freezing effect on growth factors
With regard to platelet factors, when freezing was performed after activation, i.e. platelet lysate, no differences were observed in either PDGF or TGF-B (). The levels of VEGF were significantly reduced in PRP samples frozen at −20°C, both at one and three months, compared to fresh samples (p < .05; ). At −80°C, the differences did not reach statistical significance, but a similar trend of decreasing VEGF was observed.
Figure 3. Platelet growth factors levels. Mean values of platelet growth factors concentration (A/D = PDGF, B/E = TGFB, C/F = VEGF) in the PRP according to activation time (A/B/C = before freezing, D/E/F = after thawing), storage temperatures (−20/–80ºC) and storage time (Fresh, 1 month, 3 months). Error bars = standard deviation. * p < .05; **** p < .0001.
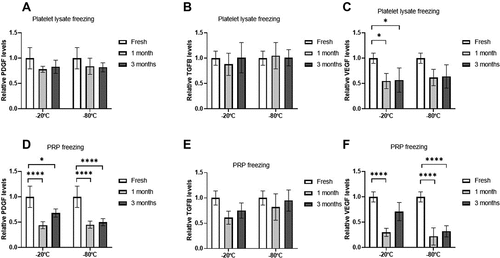
When inactivated PRP was frozen, both PDGF and VEGF showed a significant reduction in their levels at both −20°C and −80°C and at one and three months (p < .0001; ). No significant changes were seen for TGF-β after freezing.
No significant differences were found between fresh and frozen samples for extra-platelet growth factor levels, regardless of whether they were activated before or after freezing ().
Figure 4. Extra-platelet growth factors levels. Mean values of extra-platelet growth factors concentration (A / C = IGF-1, B / D = HGF) in the PRP according to activation time (A / B = before freezing, C / D = after thawing), storage temperatures (−20/−80ºC) and storage time (Fresh, 1 month, 3 months). Error bars = standard deviation. * p < .05; **** p < .0001.
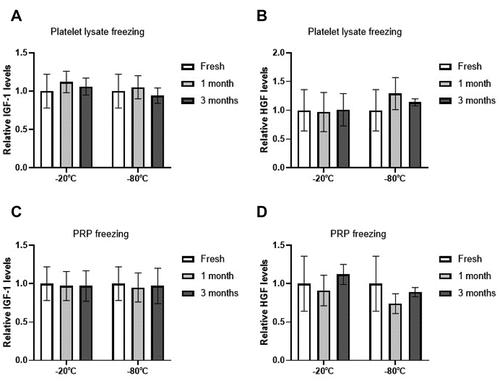
Freezing effect on bioactivity
The growth curves generated to see the effect of freezing PRP and platelet lysates on their bioactivity showed that cells respond in the same way, in terms of proliferation, to fresh and frozen samples, and that there was no significant difference in the growth pattern for each one ().
Figure 5. Growth curves of dermal fibroblasts. Normal human dermal fibroblasts were cultured with a medium supplemented with either fresh or frozen platelet lysate in triplicate, and cell viability was measured every 24 hours. A = platelet lysate obtained before freezing; B = platelet lysate obtained after thawing. Error bars = standard deviation.
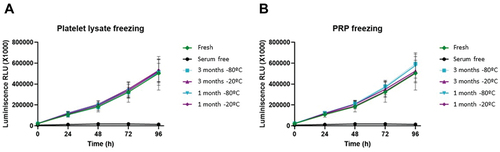
Freezing effect on clot formation
Fibrinogen measurement showed that freezing generated no significant changes in fibrinogen levels ().
Figure 6. Fibrinogen levels and clot properties of the PRP after freezing. Mean values of fibrinogen levels (A), Young’s modulus (B) and dissipated energy (C) of the fibrin clot according to different storage temperatures (−20/−80ºC) and time (Fresh, 1 month, 3 months). Error bars = standard deviation.
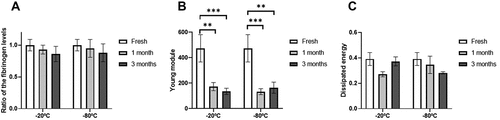
When analyzing the biomechanical properties of the fibrin clot, it was observed that the one obtained from the frozen samples had a significantly lower stiffness than the one obtained from the fresh samples (p < .01) (). However, there were no significant differences when comparing the energy absorption capacity ().
Discussion
The present study demonstrates that, overall, although PRP preservation by freezing could affect some of its characteristics, it does not affect its bioactivity, which indicates that this could be an acceptable and feasible option for its conservation.
Regarding platelet properties, while the freezing process does not alter the number of platelets, differences in platelet size were seen when preservation was carried out at −20°C, both at 1 month and 3 months, which could be a consequence of processes that alter platelet morphology and functionality. This increase in size could mean a possible platelet aggregation or morphological change, which has been previously observed by electron microscopy.Citation17 In fact, there are previous studies where it is reported that, after freezing, platelets suffer alterations in terms of morphology and biochemistry.Citation18 Increased platelet volume has also been previously associated with swelling and, thus, with a possible initiation of platelet activation.Citation19 Surprisingly, no significant differences in platelet activation have been observed between fresh and frozen samples in this study. The finding that activation is unaffected by freezing collides with the fact that the freeze/thaw cycles are commonly used for platelet activation.Citation20,Citation21 However, platelet activation results should be taken with caution since other observed findings such as morphological change may indicate the presence of this process. The use of other markers for platelet activation may yield more enlightening results in this regard.Citation22
The coagulation also proceeded in a similar way in all cases, which is consistent with the fact that the fibrinogen content did not vary between fresh and frozen samples. However, the resultant clot from frozen PRP had dissimilar properties in terms of elasticity from that generated from the fresh PRP. This was concluded based on the Young’s modulus presented by the different clots where it was seen that frozen PRP generated more elastic clots than fresh PRP. This decrease in clot firmness is consistent with the work of Lejdarova et al. in which the authors analyzed the mechanical properties of clots from cryopreserved platelets.Citation23 However, their energy dissipation capacity, which is directly related to their damping capacity, is maintained. These data suggest that the observed changes in the biomechanical properties of the clot should not be an impediment to its use in surgery, where clots typically have to resist joint impact forces.Citation24
Differences in platelet growth factor levels were also detected between fresh and frozen samples. In fact, it was observed that, while plasmatic levels remained at the same levels, platelet-derived ones suffered a decrease due to the freezing process. On the one hand, the fact that plasmatic molecules remain unchanged ensures the maintenance of PRP bioactivity since, in addition to platelet growth factors, plasmatic ones have also been proven to contribute substantially to the bioactivity of PRP, specifically IGF-1.Citation25,Citation26 On the other hand, variation in platelet growth factors were seen in PDGF and VEGF, but not in TGF-β, and were independent from the freezing time, temperature or whether the PRP had been previously activated or not. Though, it seems favorable to preserve platelet lysate and not PRP due to the fact that PDGF levels are maintained intact. This could suggest that morphological changes in platelets during their cryopreservation could affect their functionality and release of growth factors. In this regard, the importance of activating platelets before freezing the PRP, to prevent platelet degranulation after freezing has been also reported,Citation12,Citation27 which, however, is contrary to the findings of this work. However, in clinical use, PRP is usually infiltrated immediately after activation so that the clot is generated within the injured tissue, leading to a controlled release of the growth factors; thus, the PRP preservation, rather that platelet lysates, would seem more interesting from a clinical point of view. The alteration of the growth factor levels after freezing preservation come in line with the study conducted by Hosnuter et al where they found a reduction in platelet growth factors in the frozen samples compared to the fresh control.Citation28 In contrast, in an equine model, McClain et al found that after preservation of PRP at −80°C for one month, the platelet factors PDGF and TGFB remained stable, while the plasma factor IGF-1 decreased significantly.Citation15 Thus, while it is true that the PL seems to be more suitable as there is less growth factor degradation, for occasions where a more gradual release is required, it would be more convenient to use PRP to achieve the fibrin matrix, and therefore a controlled release system.Citation6
However, despite the changes observed in growth factor levels between fresh and frozen samples, no differences in plasma bioactivity in terms of boosting cell proliferation were seen. As mentioned above, this could be due to the unaltered levels of IGF-1 during the freezing process, which is a key molecule in cell proliferation.Citation29 Considering this to be the most important milestone in the study as, despite the alterations, neither platelet lysates nor PRP properties are altered, the study suggests freezing is an acceptable preservation method at both −20°C and −80°C for up to three months. Future research with longer study times is needed to assess the importance of long-term preservation times.
The potential clinical relevance of these findings is that this is a valid method to store PRP for future use. This is especially relevant for allogeneic use, in those patients with difficulties or apprehension at the time of puncture for blood collection as well as for clinics where the PRP protocol has repeated injections with weekly intervals or for patients expecting repeated cycles (e.g. repeat at 3 the PRP injection).
The main limitations are methodological, as the only cellular process studied in vitro was cell viability. In addition, aggregometry techniques, which could provide relevant information about the state of platelets after freezing, were not performed. Additionally, future studies analyzing larger sample size and longer preservation times would be necessary to understand possible long-term changes arising from freezing.
Conclusions
This study demonstrates that 1- and 3-month preservation of PRP by freezing at −20°C and −80°C could affect platelet characteristics and clot elasticity, as well as certain platelet-derived growth factor levels. However, importantly, its bioactivity is not affected in terms of boosting cell viability in vitro. Based on these results, it could be assumed that the preservation of PRP by freezing is a feasible and suitable option for its further use.
Acknowledgments
The authors are grateful for the technical and human support provided by Javier Aldazabal at the University of Navarra.
Disclosure statement
No potential conflict of interest was reported by the author(s).
Additional information
Funding
References
- Everts P, Onishi K, Jayaram P, Lana JF, Mautner K. Platelet-rich plasma: new performance understandings and therapeutic considerations in 2020. Int J Mol Sci. 2020;21(20):7794. doi: 10.3390/ijms21207794.
- Lacci KM, Dardik A. Platelet-rich plasma: support for its use in wound healing. Yale J Biol Med. 2010;83:1–8.
- Pavlovic V, Ciric M, Jovanovic V, Stojanovic P. Platelet rich plasma: a short overview of certain bioactive components. Open Med. 2016;11(1):242–7. doi: 10.1515/med-2016-0048.
- Padilla S, Sánchez M, Orive G, Anitua E. Human-based biological and biomimetic autologous therapies for musculoskeletal tissue regeneration. Trends Biotechnol. 2017;35(3):192–202. doi: 10.1016/j.tibtech.2016.09.008.
- Heijnen H, van der Sluijs P. Platelet secretory behaviour: as diverse as the granules … or not? J Thromb Haemost. 2015;13(12):2141–51. doi: 10.1111/jth.13147.
- Anitua E, Zalduendo MM, Alkhraisat MH, Orive G. Release kinetics of platelet-derived and plasma-derived growth factors from autologous plasma rich in growth factors. Ann Anat Anat Anz Off Organ Anat Ges. 2013;195(5):461–6. doi: 10.1016/j.aanat.2013.04.004.
- Moore GW, Maloney JC, Archer RA, Brown KL, Mayger K, Bromidge ES, Najafi MF. Platelet-rich plasma for tissue regeneration can be stored at room temperature for at least five days. Br J Biomed Sci. 2017;74(2):71–7. doi: 10.1080/09674845.2016.1233792.
- Wen Y-H, Lin W-Y, Lin C-J, Sun Y-C, Chang P-Y, Wang H-Y, J-J L, Yeh W-L, Chiueh T-S. Sustained or higher levels of growth factors in platelet-rich plasma during 7-day storage. Clin Chim Acta Int J Clin Chem. 2018;483:89–93. doi: 10.1016/j.cca.2018.04.027.
- Wilson BH, Cole BJ, Goodale MB, Fortier LA. Short-term storage of platelet-rich plasma at room temperature does not affect growth factor or catabolic cytokine concentration. Am J Orthop Belle Mead NJ. 2018;47(4). doi: 10.12788/ajo.2018.0022.
- Shiga Y, Kubota G, Orita S, Inage K, Kamoda H, Yamashita M, Iseki T, Ito M, Yamauchi K, Eguchi Y, et al. Freeze-dried human platelet-rich plasma retains activation and growth factor expression after an eight-week preservation period. Asian Spine J. 2017;11(3):329–36. doi: 10.4184/asj.2017.11.3.329.
- Huber SC, JLRC J, Silva LQ, Montalvão SAL, Annichino-Bizzacchi JM. Freeze-dried versus fresh platelet-rich plasma in acute wound healing of an animal model. Regen Med. 2019;14(6):525–34. doi: 10.2217/rme-2018-0119.
- Roffi A, Filardo G, Assirelli E, Cavallo C, Cenacchi A, Facchini A, Grigolo B, Kon E, Mariani E, Pratelli L, et al. Does platelet-rich plasma freeze-thawing influence growth factor release and their effects on chondrocytes and synoviocytes? Biomed Res Int. 2014;2014:692913. doi: 10.1155/2014/692913.
- Whitney KE, Dornan GJ, King J, Chahla J, Evans TA, Philippon MJ, LaPrade RF, Huard J. The effect of a single freeze-thaw cycle on matrix metalloproteinases in different human platelet-rich plasma formulations. Biomedicines. 2021;9(10):1403. doi: 10.3390/biomedicines9101403.
- Kaux J-F, Libertiaux V, Dupont L, Colige A, Denoël V, Lecut C, Hego A, Gustin M, Duwez L, Oury C, et al. Platelet-rich plasma (PRP) and tendon healing: comparison between fresh and frozen-thawed PRP. Platelets. 2020;31(2):221–5. doi: 10.1080/09537104.2019.1595563.
- McClain AK, McCarrel TM. The effect of four different freezing conditions and time in frozen storage on the concentration of commonly measured growth factors and enzymes in equine platelet-rich plasma over six months. BMC Vet Res. 2019;15(1):292. doi: 10.1186/s12917-019-2040-4.
- Kon E, Di Matteo B, Delgado D, Cole BJ, Dorotei A, Dragoo JL, Filardo G, Fortier LA, Giuffrida A, Jo CH, et al. Platelet-rich plasma for the treatment of knee osteoarthritis: an expert opinion and proposal for a novel classification and coding system. Expert Opin Biol Ther. 2020;20(12):1–14. doi: 10.1080/14712598.2020.1798925.
- Tegegn TZ, De Paoli SH, Orecna M, Elhelu OK, Woodle SA, Tarandovskiy ID, Ovanesov MV, Simak J. Characterization of procoagulant extracellular vesicles and platelet membrane disintegration in DMSO-cryopreserved platelets. J Extracell Vesicles. 2016;5(1) doi: 10.3402/jev.v5.30422.
- Six KR, Compernolle V, Feys HB. Platelet biochemistry and morphology after cryopreservation. Int J Mol Sci. 2020;21(3):935. doi: 10.3390/ijms21030935.
- Park IJ, Lim YA, Cho SR. Usefulness of delta value of platelet parameters on ADVIA 120 for the functional reactivity of stored platelets. J Clin Lab Anal. 2010;24(1):38–43. doi: 10.1002/jcla.20364.
- Baraño JL, Hammond JM. Serum-free medium enhances growth and differentiation of cultured pig granulosa cells. Endocrinology. 1985;116(1):51–8. doi: 10.1210/endo-116-1-51.
- Rauch C, Feifel E, Amann E-M, Spötl HP, Schennach H, Pfaller W, Gstraunthaler G. Alternatives to the use of fetal bovine serum: human platelet lysates as a serum substitute in cell culture media. ALTEX. 2011;28(4):305–16. doi: 10.14573/altex.2011.4.305.
- Ye S, Wang H, Zhao F, Yuan T, Liang J, Fan Y, Zhang X. Evaluating platelet activation related to the degradation of biomaterials using molecular markers. Colloids Surf B Biointerfaces. 2019; 184:184. doi: 10.1016/j.colsurfb.2019.110516.
- Lejdarova H, Pacasova R, Tesarova L, Koutna I, Polokova N, Michlickova S, Dolecek M. Cryopreserved buffy-coat-derived platelets reconstituted in platelet additive solution: a safe and available product with sufficient haemostatic effectiveness. Transfus Apher Sci off J World Apher Assoc off J Eur Soc Haemapheresis. 2021;60(3):103110. doi: 10.1016/j.transci.2021.103110.
- Sánchez M, Delgado D, Sánchez P, Fiz N, Azofra J, Orive G, Anitua E, Padilla S. Platelet rich plasma and knee surgery. Biomed Res Int. 2014;2014:890630. doi: 10.1155/2014/890630.
- Disser NP, Sugg KB, Talarek JR, Sarver DC, Rourke BJ, Mendias CL. Insulin-like growth factor 1 signaling in tenocytes is required for adult tendon growth. FASEB J Off Publ Fed Am Soc Exp Biol. 2019;33(11):12680–95. doi: 10.1096/fj.201901503R.
- Zhong J, Zhang J, Zhou Z, Pan D, Zhao D, Dong H, Yao B. Novel insights into the effect of deer IGF-1 on chondrocyte viability and IL-1β-induced inflammation response. J Biochem Mol Toxicol. 2023;37(1):e23227. doi: 10.1002/jbt.23227.
- Steller D, Herbst N, Pries R, Juhl D, Hakim SG. Impact of incubation method on the release of growth factors in non-Ca2±activated PRP, Ca2±activated PRP, PRF and A-PRF. J Cranio-Maxillo-Fac Surg off Publ Eur Assoc Cranio-Maxillo-Fac Surg. 2019;47(2):365–72. doi: 10.1016/j.jcms.2018.10.017.
- Hosnuter M, Aslan C, Isik D, Caliskan G, Arslan B, Durgun M. Functional assessment of autologous platelet-rich plasma (PRP) after long-term storage at -20 °C without any preservation agent. J Plast Surg Hand Surg. 2017;51(4):235–9. doi: 10.1080/2000656X.2016.1237956.
- Beitia M, Delgado D, Mercader J, Sánchez P, López de Dicastillo L, Sánchez M. Action of platelet-rich plasma on in vitro cellular bioactivity: more than platelets. Int J Mol Sci. 2023;24(6):5367. doi: 10.3390/ijms24065367.