Abstract
Extracellular vesicles (EVs) contain the characteristics of their cell of origin and mediate cell-to-cell communication. Platelet-derived extracellular vesicles (PEVs) not only have procoagulant activity but also contain platelet-derived inflammatory factors (CD40L and mtDNA) that mediate inflammatory responses. Studies have shown that platelets are activated during storage to produce large amounts of PEVs, which may have implications for platelet transfusion therapy. Compared to platelets, PEVs have a longer storage time and greater procoagulant activity, making them an ideal alternative to platelets. This review describes the reasons and mechanisms by which PEVs may have a role in blood transfusion therapy.
Plain Language Summary
What is the context?
Platelet transfusion is a treatment that can be effective in preventing bleeding and reducing the amount of bleeding. However, platelet transfusion may cause some unsatisfactory effects for patients, such as adverse transfusion reactions and poor prognosis in cancer patients. These benefits and harms caused by platelet transfusion may be related to PEVs. With the prolongation of storage time during the shelf life of platelets, PEVs were continuously released and the therapeutic effect of platelet components seems to get worse.
What is new?
This article not only reviews the evidence for PEVs plays a role in blood transfusion therapy but also introduces the mechanism of PEVs in platelet storage lesion and the common methods of isolation and characterization of PEVs.
What is the impact?
It is necessary to improve the method of isolation and purification of PEVs, to increase the purity of PEVs isolation, and to further demonstrate the potential of PEVs to replace platelets. Further research into the mechanisms by which platelets and PEVs affect the prognosis of cancer patients is required.
Introduction
Platelet transfusion is currently an irreplaceable therapy for treating or preventing bleeding and accelerating wound healing in cancer, hematology, surgery, and trauma patients.Citation1,Citation2 Platelet concentrates (PCs) form nearly ten percent of all blood components (BCs) that are transfused.Citation3 Platelets (PLTs) are usually stored for only 5 days. During storage, the platelets’ morphology and function are gradually damaged, a process known as platelet storage lesion (PSL).Citation4,Citation5 With the prolongation of storage time, platelet activation increased and platelet extracellular vesicles (PEVs) were continuously released.Citation6 EVs (50 nm to over 1 μm in diameter) contain the membrane structure and cytoplasmic components of the originating cells.Citation7,Citation8 Moreover, EVs are both a means for secretory cells to process intracellular components and an important mediator of intercellular signaling.Citation6,Citation9 Studies have shown that PEVs are not only rich in tissue factor (TF) and phosphatidylserine (PS) for hemostasis, but also contain significant amounts of growth factors that promote wound healing and inflammatory factors involved in the transfusion response to platelet transfusion.Citation10,Citation11 Therefore, the efficacy of platelet transfusion and the occurrence of adverse transfusion reactions are likely to be influenced by PEVs.
The immunologic function of platelets
As well as being involved in clotting and mediating inflammatory responses, platelets are now considered to be immune cells ().Citation1,Citation12 Activated platelets not only initiate hemostasis but also perform immune functions.Citation13 First of all, activated platelet secrete a large number of biological response regulators (BMRs), including growth factors (kininogen, thrombospondin, etc.), Cytokines/Chemokines (sCD40L/CD40L, CD62P, platelet factor 4 (PF4), interleukin 1β, and factors that regulate the expression of activated normal T cells and secretion (RANTES), etc.).Citation5,Citation14 Cytokines/chemokines (CKs/CHs) directly or indirectly regulate the innate and adaptive immune system through binding to adhesion receptors expressed on immune cells or other cells such as endothelial cells.Citation5,Citation14 CD40L is produced during platelet storage and can enhance the interaction of platelets with immune cells, which is associated with serious side effects of platelet transfusion (e.g. transfusion-related acute lung injury) ().Citation15,Citation16 Moreover, Platelets express immune receptors and Toll-like receptors (TLRs) (). FCR (FcεRII, FcγRIIA, and FcαRI) can recognize immunoglobulins of the IgE, IgG, and IgA subclasses.Citation17 FcγRIIA on the platelet surface can bind to the Fc parts of anti-PF4/polyanion antibodies leading to heparin-induced thrombocytopenia (HIT).Citation18 Platelets contain all Toll-like receptors (TLR1 to TLR10), which are key regulators in initiating the innate immune response to foreign organisms.Citation19 During storage, HMGB1 (a type of DAMPs) can accumulate in platelet components and their association with TLR4 triggers a unique signal transduction response that induces platelet activation and releases immunoregulatory factors.Citation13,Citation20 In addition, platelets promote the phagocytosis of bacteria by white blood cells ().Citation5 Although the innate immune system recognizes the negative charge on the surface of bacteria, the adaptive immune system cannot be activated by the negative charge.Citation21 However, platelet-derived PF4, when attached to the negative charge on the bacterial surface, can form a new epitope that can be bound by anti-PF4/polyanionic (IgG-type) antibodies and promote phagocytosis of bacteria by granulocytes.Citation5,Citation22 IgG-coated bacteria are also engulfed by platelets, which are then activated to release alpha-granules at the site of the bacteria to kill them.Citation5,Citation22
Figure 1. The immunologic function of platelets. (a) Activated platelets secrete a large number of biological response regulators (BMRs). If patients with previous inflammatory pathological conditions or external factors attracting leukocytes to lung vessels, the BMRs contained in the transfused platelet concentrations will activate neutrophils to produce ROS, which damages the pulmonary endothelium and cause transfusion-related acute lung injury. Moreover, the growth factors are associated with angiogenesis; (b) Platelets express immune receptors, and Toll-like receptors (TLR) are involved in immune regulation. During heparin treatment, if patients with anti-PF4/polyanion antibodies (These antibodies probably result from bacterial infections or heparin treatment), their Fab parts will bind to PF4/heparin complexes, and then FcγRIIA on the platelet surface can bind to the Fc parts of anti-PF4/polyanion antibodies leading to HIT. TLR4 can bind to damage-associated molecular patterns (DAMPs, e.g. HMGB1) to trigger platelet activation and the release of BMRs. (c) Platelets can interact directly or indirectly with bacteria to achieve a bactericidal effect. Lipopolysaccharides on the surface of bacteria activate platelets and promote the production of PF4, which can bind to the negative charge of the bacterial surface, exposing a new epitope. This epitope is bound by anti-PF4/polyanion antibodies, promoting phagocytosis of the bacteria by granulocytes. Platelets can also internalize IgG-encapsulated bacteria to achieve bactericidal effects.
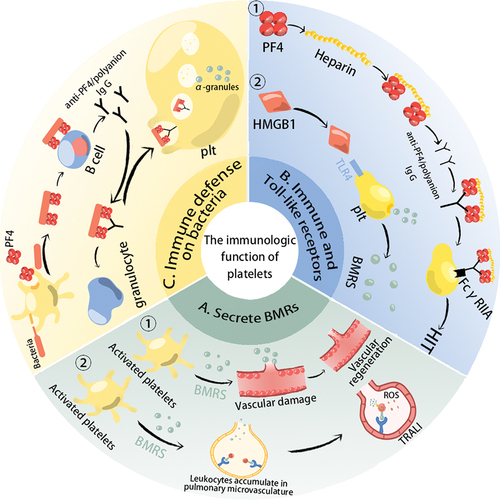
As a multifunctional immune cell, platelet transfusion is a “cell transplantation” that can actively participate in the immune regulation of the host, which may harm the efficacy of transfusion.Citation23 Numerous mediators produced during platelet storage are involved in a variety of adverse reactions, leading to an increased risk of platelet transfusion and shortened infusion intervals.Citation24 What’s more, PEVs produced during storage are highly homologous to platelets, have similar functions to platelets, and mediate intercellular communication.Citation7,Citation8 PEVs are believed to be related to enhanced platelet aggregation and regulation of the immune system, may influence the efficacy of platelet transfusion, and participate in the adverse reactions of immune transfusion.Citation2,Citation7,Citation8
Platelet produce PEVs during storage
Production mechanisms of PEVs
Platelet storage lesions (PSL) began at the time of blood collection and progressed through reprocessing operations and storage. Platelet activation due to the method of platelet concentrate preparation and storage conditions is the main cause of PSL.Citation25 With the prolongation of storage time, the intracellular Ca2+ levels were gradually increased, which mediates the activation of gelsolin and the polymerization of actin, ultimately leading to changes in platelet morphology and membrane lipids.Citation26 Under resting conditions, the inner leaflet of the platelet lipid membrane consists mainly of phosphatidylserine (PS) and phosphatidylethanolamine (PE), and the outer leaflet consists mainly of sphingomyelin (SM) and phosphatidylcholine (PC).Citation27 Adenosine triphosphate (ATP)-dependent translocase maintains the stability of the inner leaflet of the platelet membrane (transfers PS and PE to the inner leaflet), and ATP-dependent floppase maintains the stability of the outer leaflet (transfers SM and PC to the outer leaflet).Citation28 The increase of Ca2+ level activates Calcium-dependent scramblase and inhibits ATP-dependent transporters and floppase, resulting in asymmetric changes in membrane phospholipid molecules and promoting the expression of PS and PE on the outer leaflet of the platelet membrane ().Citation8 As the significant alterations of membrane lipids and restructure of the cytoskeleton filaments, the platelet membrane is prone to foaming, and the extracellular domain of the platelet receptor falls off.Citation29 Increased Ca2+ can also lead to increased generation of platelet microvesicles (PMVs) by promoting the activation of calcium-dependent thiol proteases and calpain.Citation30 In addition, the activation of NADPH oxidase (NOX2) during platelet storage causes the production of O2-, which is rapidly converted to H2O2 by superoxide dismutase (SOD), mobilizing intracellular calcium, promoting platelet activation and accelerating PEV production.Citation31 Platelet exosome (P-exos) formation begins with membrane bulging into the endosome lumen, which is subsequently converted into a multivesicular body (MVB). Finally, MVBs are either fused to the plasma membrane for release as exosomes or are taken up by lysosomes for degradation.Citation29 Some researchers have proved the process of exosome secretion in several cell types is regulated by Ca2+, but whether it applies to P-exos is still unclear.Citation32 Some studies have also proposed that the activation and closure of integrin αIIbβ3, cytoskeletal rearrangement, and its related signaling pathways have a crucial role in the release of EVs.Citation33
Figure 2. The mechanism of PEVs produced by platelet during storage. With the intracellular Ca2+ level was gradually increased during platelet storage, the membrane phospholipid molecules have been asymmetrically changed, which promotes the generation of platelet microvesicles (PMVs). The platelet exosomes (P-exos) generation begins with the membrane bulging into the lumen of endsome, which is converted into a multi-vesicular body (MVB), then the MVBs fuse with the plasma membrane to secrete exosomes. Both PMVs and P-exos belong to PEVs. The biomarkers and contents of platelet-derived extracellular vesicles (PEVs) usually contain CD62P, CD42a, CD9, CD63, CD41b, PF4, and RNA.
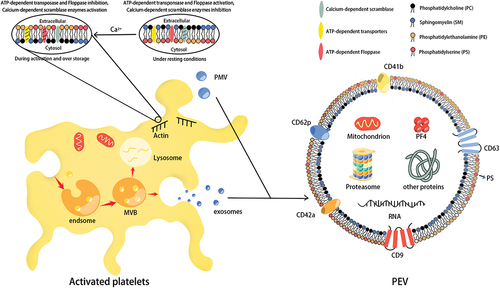
PLTs preparation and storage conditions can affect the production and characterization of PEVs
Platelet products are prepared from whole blood by centrifugation.Citation34 The high centrifugal force during preparation leads to increased platelet activation and the production of PEVs. Buffy Coat (BC) prevents direct contact between platelets and the container during centrifugation and reduces platelet activation due to centrifugation. Therefore, there are fewer PEVs than platelet-rich plasma (PRP) PCs in the buffy coat (BC) and apheresis PCs (collected by the Trima device).Citation34,Citation35 The purified platelets are suspended in plasma, platelet additive solution (PAS), or a mixture of both.Citation36–38 In addition to saving more plasma products, the use of PAS is also effective in reducing the side effects of plasma on patients; for example, the infusion of PAS-preserved PC results in a significant reduction in allergic reactions in patients.Citation36,Citation38 In addition, the acetates contained in PAS, by reducing the level of lactic acid and producing bicarbonate, prevent the pH in the PC from falling and reduce the activation of platelets.Citation36,Citation38 Studies have shown that PCs preserved in PAS contain fewer EVs than those preserved in plasma.Citation35 There are also slight differences in the number and size of PEVs due to the use of different PAS. Compared to PAS-B, the presence of phosphate (improves buffering capacity), potassium, and magnesium (reduces lactate production) in PAS-E further reduces platelet activation and the production of PEVs.Citation38 In addition, the diameter of PEVs in PAS-B increased with storage time, whereas the diameter of PEVs in PAS-E did not change significantly.Citation38
Shaking at room temperature can preserve platelet activity and function well, but also provides a favorable environment for bacterial growth.Citation39–41 Although platelets are screened before use, window period infections and emerging pathogens limit the accuracy of screening.Citation42 Pathogen Inactivation Technology (PRT) inactivates pathogens by interfering with bacterial replication and protein assembly. Common pathogen reduction technology systems available today include Intercept® (amoxicillin and UVA combined), Mirasol® (riboflavin/vitamin B2 and UVA/UVB combined), and Theraflex® (UVC only). Although PRTs are effective in increasing the security of PCs and prolonging storage time, they can exacerbate PSL and promote the release of PEVs.Citation39,Citation42 Furthermore, PRTs altered the microRNA content of PEVs.Citation43,Citation44 The main effect of Mirasol® was a slight reduction in the levels of several microRNAs (e.g. hsa-miR-22-3p or hsa-miR-423-5p). Intercept® reduced the levels of some microRNAs (e.g. hsa-miR-185-5p) while increasing the levels of others (e.g. hsa-miR-451a). By transferring microRNAs, PEVs can alter the function of macrophages.Citation29 As hsa-miR-451a can downregulate macrophage migration inhibitory factor (MIF) and promote M1 and M2 polarization in macrophages, the transfer of PEVs containing this MiRNA may affect macrophage function in PC recipients.Citation44
It was once thought that cold storage (4°C) and cryopreservation (−80°C) caused dramatic changes in platelet morphology and function, resulting in reduced platelet survival and recovery rates after transfusion.Citation13,Citation36,Citation39 However, the corrected count increment (CCI) and platelet recovery rates are not fully representative of platelet function.Citation2 Recent studies have shown that the hemostatic efficacy of cold-stored PLTs (CCPs) and cryopreserved PLTs (CPPs) is comparable or superior to that of PLTs stored at room temperature.Citation45,Citation46 This may be related to cold temperatures causing platelets to pre-activate and produce large amounts of PS+ PEVs.Citation47,Citation48 The concentration of PEVs in CPPs components is 15 times higher than in platelets stored at room temperature and 10 times higher than in CCPs.Citation47,Citation48 In contrast to PEVs from fresh platelets, CPPs had higher levels of PS+ PEVs and PEVs showed increased expression of several adhesion receptors (e.g. GPIIb, Gpix, and integrin-related proteins).Citation39,Citation49 CCPs and CPPs are a good alternative to storing platelets at room temperature, not only reducing the risk of bacterial contamination but also preserving the clotting activity of the platelets.Citation13,Citation36,Citation39 However, due to the high cost, time, complexity, and need for further research, these methods are not yet widely available.Citation36
Studies have shown that PAS can reduce PSLs and platelet activation caused by PRTs and hypothermia.Citation50 PAS is also expected to reduce PEVs levels in PRTs and cryogenic-treated PCs, but further experimental analysis is required to prove this.Citation34 Due to several limitations, such as the large number of PEVs required to characterize EVs and the many drawbacks of the methods used to isolate and purify PEVs, most research only compares the number of PEVs in different preparation methods and does not characterize PEVs.Citation36,Citation51
Separation and characteristic analysis of PEVs
Depending on their release mechanism, PEVs are commonly classified as exosomes, microparticles or microvesicles, and apoptotic bodies (EVs released by apoptotic platelets).Citation52 Platelet exosomes (P-exos), with a diameter of about 40–100 nm, are formed by the inward budding of the intracellular granular membrane and released by the exocytosis of secretory granules; Microparticles (MPs), also known as microvesicles (MVs), with a diameter of about 100-1000 nm, are vesicles released by the budding of the cytoplasmic membrane.Citation53 The largest EVs, apoptotic bodies (ABs, 1–5 m in diameter), represented aggregates produced at the late stage of apoptosis, which is cleared by phagocytes immediately after production and regardless of intracellular communication.Citation54 When using ultracentrifugation to separate PEVs, 20000 g is used to obtain mainly large PEVs (>200 nm) and 100,000 g to obtain mainly small and medium PEVs (<200 nm).Citation55
Separation of PEVs
There is no optimal method to isolate, classify and characterize PEVs.Citation56 Common methods for the separation PEVs include ultrafiltration, Polymer precipitation, density gradient centrifugation, ultracentrifugation, asymmetric flow field-flow, size exclusion chromatography (SEC), and immune affinity ().Citation57 SEC has a recovery of up to 90%. Compared to ultracentrifugation, SEC is effective in preventing the aggregation of EVs and is better able to retain the integrity and biological function of PEVs.Citation58 Although asymmetric flow-field-flow fractionation is currently the best method for PEVs separation, the yield is poor and the process is very complicated [59]. Ultracentrifugation is regarded as the gold standard method for separating EVs populations, allowing for the removal of platelets while obtaining PEVs of moderate purity.Citation59 Immune affinity does not efficiently elute the entire EVs from the separation beads, but high purity and exosomes of specific origin are obtained.Citation55 It is possible to obtain purer PEVs using a novel method combining multiple separation methods. For example, a combination of size exclusion chromatography and ultracentrifugation has been used to obtain pure PEVs, but the concentrations have not reached the sample concentrations required to test the functional properties of PEVs.Citation58
Table I. Commonly used methods and differences in isolation of PEVs.
Characteristic analysis of PEVs
Microstructures of PEVs were usually observed under Low Voltage Scanning Electron Microscopy (LVSEM), confocal microscope, atomic force microscope (AFM), transmission electron microscope (TEM), and cryo-electron microscopy (cryo-EM).Citation64 Cryo-EM is currently the most advanced method for PEV characterization. Under the observation of cryo-EM, there were three main forms of PEVs released by platelet activation: spherical EVs (50 nm-1 μm in diameter); tubular EVs (1–5 μm in length); and membrane fragments that are neither circular nor tubular.Citation65 The quantification and characterization of PEVs were mainly performed using flow cytometry (FC), nanoparticle tracking analysis (NTA), and tunable resistance pulse sensing (TRPs).Citation66 Flow cytometry is the gold standard for the detection and characterization of PEVs and is widely used to identify and quantify PS, CD41, and CD62P on the surface of PEVs.Citation67 Based on lipid-specific fluorescent probes and light-scattering event detection, the high-sensitivity FC (hs-FC) can detect vesicles down to 60 nm.Citation68 In contrast to conventional flow cytometry, NTAs can not only detect EVs smaller than 300 nm in diameter, but also analyze the concentration, refractive index, and size of individual EVs suspended in liquid.Citation55 TRPS can accurately measure the size, count, and concentration of EVs and analyze the zeta potential of individual EVs by detecting the resistance pulses of particles passing through the nanopore.Citation69 Furthermore, TRPS can distinguish between different types of EVs within a single experiment by tuning the size and conductivity properties of the nanopores.Citation70 However, the separated samples need to be pure as NTA and TRP cannot distinguish between PEVs and impurities.Citation71
In addition to the expression of EVs surface markers (CD9, CD63, TSG101, ALIX), PEVs also express PECAM-1 (CD31), glycoprotein GPIIb/IIIa (CD41/CD61), GPIba (CD42b), GPIaIIa(CD49b/CD29), p-selectin (CD62P), platelet factor 4 (PF4), and phosphatidylserine (PS), which are characteristic of platelets.Citation72 According to the recommendations of The Minimum Information for Extracellular Vesicle Studies 2018 (MISEV2018) guidelines, two requirements are needed to characterize EVs: 1. At least three positive protein markers, including ≥ 1 transmembrane/GPI-anchored protein and ≥ 1 cytosolic protein; 2. At least one negative marker.Citation73 As transmembrane proteins, CD9 and CD63 can prove the existence of lipid bilayer of EVs.Citation74 CD41b, CD42a, and CD62P can be used as platelet-specific markers.Citation75 PF4 is a specific cytoplasmic protein synthesized by platelet alpha-granules and can be present in PEVsCitation76. In addition, apolipoproteins A1/2 and B or albumin may be used as a negative control to assess the purity of PEVs.Citation75 It is generally believed that P-exos do not expose PS but selectively enrich for the exosome-specific marker CD63, whereas PMVs usually expose PS on its outer surface and contain coagulation-related factors (e.g., FX, prothrombin, etc.).Citation77,Citation78 In addition, P-exos are rich in protein derived from platelet alpha-granules, while PMVs contain many lipid mediators, mitochondrial proteins, and proteasomes.Citation29,Citation78,Citation79 High-sensitivity flow cytometry showed a large increase in the number of PEVs during storage, which may have important implications for the transfusion therapy of platelets and is worthy of our attention.Citation68
PEVs have an important role in platelet transfusion therapy
The main role of platelet transfusions is to prevent and treat bleeding.Citation80 However, platelets also contain a variety of inflammation factors involved in the inflammatory responseCitation13 and contain molecules related to the regenerative processes promoting angiogenesis as well as tissue regeneration.Citation81 Therefore, platelet transfusion therapy may have both positive and negative effects on patients, and PEVs may be a major contributor to these effects.Citation82
PEVs as a biomarker of platelet storage lesions
The number of platelets does not change much during PLT storage, but great changes take place in morphology, function, and metabolism.Citation83 The expression of P-selectin (CD62P) is considered to be the gold standard for assessing platelet function and its expression level is negatively correlated with the degree of platelet function loss and the PEVs levels.Citation84 Doubling of the number of PEVs in the PC at the end of storage and a significant decrease in platelet surface CD62P expression.Citation27,Citation83 Therefore, pretransfusion testing for PEVs in platelet products may help prevent patients from ineffective transfusions due to the introduction of severely compromised platelets.
PEVs exert the hemostasis and coagulation function of PCs
In vivo and in vitro experiments, Well-characterized PEVs have strong procoagulant activity.Citation85 Platelet-enriched plasma (PEP), derived from platelet-rich plasma (PRP), contains high levels of procoagulant PEVs that retain their function after freeze-thaw cycles.Citation85,Citation86 PEVs express TF, PS, and GPIIb/IIIa (CD41/CD61), and contain thrombospondin complex and alpha granule-derived factors V and X. Coagulation factor VII binds to TF on the surface of PEVs and activates the coagulation cascadeCitation11; PEVs (PS+) provide a negative catalytic surface for coagulation and promote the formation of tenase complexes (FVIIIa and FIXa) and prothrombinase (FVa and FXa).Citation87 In addition, PEVs (CD61+) are not only able to attach to fibrin fibers directly, but also enhance the rapid production of thrombin to support the formation of fibrin clots.Citation88 Therefore, PEVs (mainly PMVs play the role) can enhance induced hemostasis.Citation82
Even though the surface area of PEVs is approximately 100 times smaller than that of platelets, when thrombin generation and spatial clot growth parameters were measured in ultra-free plasma supplemented with different concentrations of activated platelets or PEVs (activated by the calcium ionophore A23187), the procoagulant activity of individually activated platelets was found to be almost identical to that of individual PEVs.Citation89 This suggests that the coagulation capacity of the PEVs activated by calcium ionophore A23187 is around 50–100 times greater than that of activated platelets.Citation89 In vitro studies have shown that the procoagulant effect of platelet-free plasma increases with increasing concentration of added PEVs (produced during storage).Citation10 Furthermore, injection of PEVs isolated from three-day stored de-leukocyte platelet plasma into a mouse bleeding model can significantly reduce hemorrhage.Citation10 Another study showed that PEVs provide hemostasis by enhancing hemodynamic stability in a mouse model and preventing ischemia from developing.Citation82
PEVs are involved in adverse transfusion reactions
PEVs may play a pro- or anti-inflammatory role in inflammation, according to the expression of surface markers, the release of cargo, and the influence of the microenvironment and receptor cells.Citation82 On the one hand, PEVs contain various BRMs and surface receptors (e.g. CD62p and CD61) to promote monocyte adhesion and activation.Citation8,Citation13 On the other hand, some studies have shown that PEVs can suppress inflammation by inhibiting the activation of macrophages and dendritic cellsCitation82 and reducing the production of inflammatory cytokines such as TNF-α (produced by macrophages and dendritic cells) and IL-8 (produced by dendritic cells).Citation90–92
Most studies show that PEVs have pro-inflammatory properties and are involved in adverse platelet transfusion reactions.Citation82 PEV in PC express the activation markers CD62P and CD61, which bind to P-selectin glycoprotein ligand-1 (PSGL-1) and Mac-1 expressed on the neutrophil surface, activating neutrophils to form filamentous meshwork structures (neutrophil extracellular traps, NETs) involved in the inflammatory response.Citation11,Citation13 Many BRMs in PEVs are also associated with inflammation, such as PEVs contain platelet-derived CD40L (CD154), which mediates TRALI (an acute lung injury).Citation11,Citation15 In addition, PEV transports platelet-derived mitochondria.Citation7 Circulating mitochondrial DNA (mtDNA) is an inflammatory factor that is part of the DAMPs and is associated with adverse blood transfusion reactions.Citation7 Compared to plasma and RBC products, PCs often contain higher levels of mtDNA, which may explain the much higher incidence of non-hemolytic transfusion reactions with PC transfusions than with red blood cell (RBC) transfusions.Citation11,Citation93
PEVs lead to poor prognosis of platelet transfusion in cancer patients
Thrombocytopenia is common in cancer patients.Citation94 On the one hand, the tumor can infiltrate the body’s hematopoietic organs (bone marrow, spleen), directly leading to thrombocytopenia.Citation94 On the other hand, platelet counts are significantly reduced in oncology patients after prolonged chemotherapy.Citation23 Platelet transfusion significantly reduces the risk of bleeding due to thrombocytopenia in cancer patients and is an irreplaceable means of immediate hemostasis.Citation95 However, studies have shown that activated platelets promote the proliferation and metastasis of cancer cells and that cancer cells also activate platelets—a mutually reinforcing vicious circle that may lead to platelet transfusions being highly detrimental to the prognosis of cancer patients.Citation23 PEVs contain molecules from alpha-granules like platelet-derived growth factor, vascular endothelial growth factor (VEGF), and fibroblast growth factor, which have pro-angiogenic properties.Citation29 MiRNAs in PEVs are involved in angiogenesis (e.g. miRNA Let-7 a) and tumor growth (e.g. miR-126 and miRNA-223).Citation29,Citation96
Long-term platelet transfusions in cancer patients may be harmful due to the propensity of PEVs to induce thrombosis and support tumors.Citation97 Firstly, PEVs suppress immune defense in tumor patients. The miR-183 in PEVs transferred into tumor-associated NK cells inhibits their membrane protein DAP12 activity and suppresses their cytolytic functionCitation98; The PS receptor (PSR) on immune cells can specifically recognize PS+ PEVs, leading to the innate and adaptive immune systems becoming inhibited in the tumor microenvironment (TME).Citation99 Moreover, PEVs promote tumor cell proliferation by activating mitogen-activated protein kinase (MAPK), enhance tumor cell invasion by inducing the secretion of matrix metalloproteinases (MMPs) and activating cyclin D2, and accelerate tumor metastasis by inducing epithelial-mesenchymal transition (ETM).Citation100,Citation101 In addition, PEVs induce the production of extracellular vesicles (TEVs) in tumor cells, and cancer cells can also stimulate platelet activation to produce PEVs, as TEVs initiate thrombin production.Citation29,Citation102 Therefore, giving platelet transfusion to oncology patients to reduce the risk of bleeding requires more prudent decision-making, as both platelets and PEVs may increase the progression of cancer.
Discussion
The known mechanisms by which PEVs contribute to platelet transfusions are summarized in . Although the procoagulant activity of PEVs was much higher than that of activated platelets, platelet concentrate storage time was negatively associated with clinical efficacy outcomes, which suggests that the effect of platelet transfusion is not better with the increase of PEVs. However, it is interesting to note that in vitro studies have shown that platelet-enhanced plasma, obtained by removing platelets from platelet products, has a better hemostatic effect than platelet-rich plasma, suggesting a role for PEV in promoting coagulation.Citation85 We speculate that activated platelets whose morphology and function have changed during platelet storage may interfere with the function of PEVs. PEVs have platelet properties and can retain their function after freeze-thaw cycles.Citation10 Therefore, in acute situations (e.g. trauma), infusion of PEVs has the potential to be an effective treatment alternative to platelet transfusion. As the difficulty and low purity of PEVs isolation and extraction from stored platelets, it is necessary to further improve the method of isolation and purification of PEVs and to further study their effects on human hemostasis and adverse reactions to blood transfusions.
Figure 3. PEVs contributions in platelet transfusion therapy. (a) PEVs as a biomarker of platelet storage lesions. With the prolongation of storage time, the number of platelets does not change much, but the amount of PEVs in PCs is doubled, and the level of CD62p (the gold standard for the evaluation of PLT function) expression on platelet surface is negatively correlated with PEVs level; (b) PEVs can facilitate their adhesion to leukocytes, then several BRMs produced by PEVs and neutrophil extracellular traps (NETs) formed by activated neutrophils are associated with the inflammatory response; (c) PEVs can inhibit the innate and adaptive immune response in the tumor microenvironment (TME). What’s more, PEVs could promote the proliferation, invasion, and metastasis of tumor cells; (d) PEVs can promote hemostasis and coagulation. Factor VII on the PEVs surface combines with TF to activate the coagulation cascade; PS on the PEVs surface provides a catalytic surface for coagulation and promotes prothrombin and tenase complex formation; and CD61 on the PEVs surface promotes fast thrombin generation and assists in fibrin clotting.
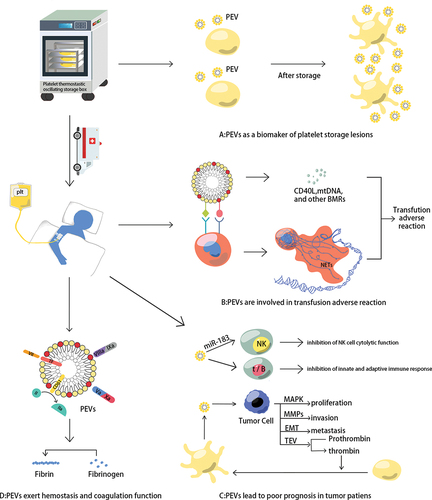
Platelet has been suggested as a multifunctional immune blood cell, adverse progress of cancer patients and adverse transfusion reactions are related to PEVs produced by platelet storage lesion. Transfusion of platelets with short storage time, leucocyte-depleted platelets, and platelet storage in platelet additive solutions (PAS) may reduce the incidence of adverse transfusion reactions.Citation103–105 Cancer patients’ overall survival could be significantly improved by washing platelets to remove cytokines and PEVs before blood transfusion.Citation23 Therefore, cancer patients should be advised to transfuse leukocyte-depleted platelets with a short storage time. Further work is needed to demonstrate how platelets and PEVs affect the prognosis of cancer patients.
Authors contributions
ZC wrote the manuscript. JYF and ND drew the figures. PZ, YSH, and HWZ revised the paper. All authors read and approved the final manuscript. Data authentication is not applicable.
Disclosure statement
No potential conflict of interest was reported by the author(s).
Additional information
Funding
References
- Prodger CF, Rampotas A, Estcourt LJ, Stanworth SJ, Murphy MF. Platelet transfusion: alloimmunization and refractoriness. Semin Hematol. 2020;57(2):92–11. doi:10.1053/j.seminhematol.2019.10.001.
- Ng MSY, Tung JP, Fraser JF. Platelet storage lesions: what more do we know now? Transfus Med Rev. 2018;S0887-7963(17)30189–X. doi:10.1016/j.tmrv.2018.04.001.
- Tissot JD, Bardyn M, Sonego G, Abonnenc M, Prudent M. The storage lesions: from past to future. Transfus Clin Biol. 2017;24(3):277–84. doi:10.1016/j.tracli.2017.05.012.
- Hosseini E, Ghasemzadeh M, Nassaji F, Jamaat ZP. GPVI modulation during platelet activation and storage: its expression levels and ectodomain shedding compared to markers of platelet storage lesion. Platelets. 2017;28(5):498–508. doi:10.1080/09537104.2016.1235692.
- Sut C, Tariket S, Aubron C, Aloui C, Hamzeh-Cognasse H, Berthelot P, Laradi S, Greinacher A, Garraud O, Cognasse F. The non-hemostatic aspects of transfused platelets. Front Med. 2018;5:42. doi:10.3389/fmed.2018.00042.
- Valkonen S, Holopainen M, Colas RA, Impola U, Dalli J, Käkelä R, Siljander PR, Laitinen S. Lipid mediators in platelet concentrate and extracellular vesicles: molecular mechanisms from membrane glycerophospholipids to bioactive molecules. Biochim Biophys Acta Mol Cell Biol Lipids. 2019;1864(8):1168–82. doi:10.1016/j.bbalip.2019.03.011.
- Marcoux G, Magron A, Sut C, Laroche A, Laradi S, Hamzeh-Cognasse H, Allaeys I, Cabon O, Julien AS, Garraud O, et al. Platelet-derived extracellular vesicles convey mitochondrial DAMPs in platelet concentrates and their levels are associated with adverse reactions. Transfusion. 2019;59(7):2403–14. doi:10.1111/trf.15300.
- Green SM, Padula MP, Marks DC, Johnson L. The lipid composition of platelets and the impact of storage: an overview. Transfus Med Rev. 2020 Apr;34(2):108–16. doi:10.1016/j.tmrv.2019.12.001.
- Cocozza F, Grisard E, Martin-Jaular L, Mathieu M, Théry C. SnapShot: extracellular vesicles. Cell. 2020;182(1):262–262.e1. doi:10.1016/j.cell.2020.04.054.
- Lopez E, Srivastava AK, Burchfield J, Wang YW, Cardenas JC, Togarrati PP, Miyazawa B, Gonzalez E, Holcomb JB, Pati S, et al. Platelet-derived- extracellular vesicles promote hemostasis and prevent the development of hemorrhagic shock. Sci Rep. 2019;9(1):17676. doi:10.1038/s41598-019-53724-y.
- Melki I, Tessandier N, Zufferey A, Boilard E. Platelet microvesicles in health and disease. Platelets. 2017;28(3):214–21. doi:10.1080/09537104.2016.1265924.
- Garraud O, Cognasse F. Are platelets cells? And if yes, are they immune cells? Front Immunol. 2015;6:70. doi:10.3389/fimmu.2015.00070.
- Wood B, Padula MP, Marks DC, Johnson L. The immune potential of ex vivo stored platelets: a review. Vox Sang. 2021;116(5):477–88. doi:10.1111/vox.13058.
- Cognasse F, Duchez AC, Audoux E, Ebermeyer T, Arthaud CA, Prier A, Eyraud MA, Mismetti P, Garraud O, Bertoletti L, et al. Platelets as key factors in inflammation: focus on CD40L/CD40. Front Immunol. 2022;13:825892. doi:10.3389/fimmu.2022.825892.
- Sprague DL, Elzey BD, Crist SA, Waldschmidt TJ, Jensen RJ, Ratliff TL. Platelet-mediated modulation of adaptive immunity: unique delivery of CD154 signal by platelet-derived membrane vesicles. Blood. 2008;111(10):5028–36. doi:10.1182/blood-2007-06-097410.
- Tariket S, Hamzeh-Cognasse H, Laradi S, Arthaud C-A, Eyraud M-A, Bourlet T, Berthelot P, Garraud O, Cognasse F. Evidence of CD40L/CD40 pathway involvement in experimental transfusion-related acute lung injury. Sci Rep. 2019;9(1):12536. doi:10.1038/s41598-019-49040-0.
- McFadyen JD, Kaplan ZS. Platelets are not just for clots. Transfus Med Rev. 2015;29(2):110–19. doi:10.1016/j.tmrv.2014.11.006.
- Krauel K, Pötschke C, Weber C, Kessler W, Fürll B, Ittermann T, Maier S, Hammerschmidt S, Bröker BM, Greinacher A. Platelet factor 4 binds to bacteria, [corrected] inducing antibodies cross-reacting with the major antigen in heparin-induced thrombocytopenia. Blood. 2011;117(4):1370–8. doi:10.1182/blood-2010-08-301424.
- Koupenova M, Mick E, Mikhalev E, Benjamin EJ, Tanriverdi K, Freedman JE. Sex differences in platelet toll-like receptors and their association with cardiovascular risk factors. Arterioscler Thromb Vasc Biol. 2015;35(4):1030–7. doi:10.1161/ATVBAHA.114.304954.
- Vogel S, Bodenstein R, Chen Q, Feil S, Feil R, Rheinlaender J, Schäffer TE, Bohn E, Frick JS, Borst O, et al. Platelet-derived HMGB1 is a critical mediator of thrombosis. J Clin Invest. 2015;125(12):4638–54. doi:10.1172/JCI81660.
- Nguyen TH, Medvedev N, Delcea M, Greinacher A. Anti-platelet factor 4/polyanion antibodies mediate a new mechanism of autoimmunity. Nat Commun. 2017;8(1):14945. doi:10.1038/ncomms14945.
- Palankar R, Kohler TP, Krauel K, Wesche J, Hammerschmidt S, Greinacher A. Platelets kill bacteria by bridging innate and adaptive immunity via platelet factor 4 and FcγRIIA. J Thromb Haemost. 2018;16(6):1187–97. doi:10.1111/jth.13955.
- Wang J, Zhou P, Han Y, Zhang H. Platelet transfusion for cancer secondary thrombocytopenia: platelet and cancer cell interaction. Transl Oncol. 2021;14(4):101022. doi:10.1016/j.tranon.2021.101022.
- Kreuger AL, Caram-Deelder C, Jacobse J, Kerkhoffs JL, van der Bom JG, Middelburg RA. Effect of storage time of platelet products on clinical outcomes after transfusion: a systematic review and meta-analyses. Vox Sang. 2017;112(4):291–300. doi:10.1111/vox.12494.
- Greening DW, Simpson RJ, Sparrow RL. Preparation of platelet concentrates for research and transfusion purposes. Methods Mol Biol. 2017;1619:31–42. doi:10.1007/978-1-4939-7057-5_3.
- Egidi MG, D’Alessandro A, Mandarello G, Zolla L. Troubleshooting in platelet storage temperature and new perspectives through proteomics. Blood Transfus. 2010;8(Suppl 3):s73–s81. doi:10.2450/2010.012S.
- Pienimaeki-Roemer A, Ruebsaamen K, Boettcher A, Orsó E, Scherer M, Liebisch G, Kilalic D, Ahrens N, Schmitz G. Stored platelets alter glycerophospholipid and sphingolipid species, which are differentially transferred to newly released extracellular vesicles. Transfusion. 2013;53(3):612–26. doi:10.1111/j.1537-2995.2012.03775.x.
- Zhao XM, Wang Y, Yu Y, Jiang H, Babinska A, Chen XY, He KG, Min XD, Han JJ, Yang CX, et al. Plasma phospholipid transfer protein promotes platelet aggregation. Thromb Haemost. 2018;118(12):2086–97. doi:10.1055/s-0038-1675228.
- Żmigrodzka M, Witkowska-Piłaszewicz O, Winnicka A. Platelets extracellular vesicles as regulators of cancer progression-an updated perspective. Int J Mol Sci. 2020;21(15):5195. doi:10.3390/ijms21155195.
- Chu Y, Guo H, Zhang Y, Qiao R. Procoagulant platelets: generation, characteristics, and therapeutic target. J Clin Lab Anal. 2021;35(5):e23750. doi:10.1002/jcla.23750.
- Ghasemzadeh M, Hosseini E, Roudsari ZO, Zadkhak P. Intraplatelet reactive oxygen species (ROS) correlate with the shedding of adhesive receptors, microvesiculation and platelet adhesion to collagen during storage: does endogenous ROS generation downregulate platelet adhesive function? Thromb Res. 2018;163:153–61. doi:10.1016/j.thromres.2018.01.048.
- van Niel G, D’Angelo G, Raposo G. Shedding light on the cell biology of extracellular vesicles. Nat Rev Mol Cell Biol. 2018;19(4):213–28. doi:10.1038/nrm.2017.125.
- Heinzmann ACA, Karel MFA, Coenen DM, Vajen T, Meulendijks NMM, Nagy M, Suylen DPL, Cosemans JMEM, Heemskerk JWM, Hackeng TM, et al. Complementary roles of platelet αIIbβ3 integrin, phosphatidylserine exposure and cytoskeletal rearrangement in the release of extracellular vesicles. Atherosclerosis. 2020;310:17–25. doi:10.1016/j.atherosclerosis.2020.07.015.
- van der Meer PF, de Korte D. Platelet additive solutions: a review of the latest developments and their clinical implications. Transfus Med Hemother. 2018;45(2):98–102. doi:10.1159/000487513.
- Maurer-Spurej E, Larsen R, Labrie A, Heaton A, Chipperfield K. Microparticle content of platelet concentrates is predicted by donor microparticles and is altered by production methods and stress. Transfus Apher Sci. 2016;55(1):35–43. doi:10.1016/j.transci.2016.07.010.
- Capraru A, Jalowiec KA, Medri C, Daskalakis M, Zeerleder SS, Mansouri Taleghani B. Platelet transfusion-insights from current practice to future development. J Clin Med. 2021;10(9):1990. Published 2021 May 6. doi:10.3390/jcm10091990.
- van Hout FMA, Middelburg RA, van der Meer PF, Pors A, Wiersum-Osselton JC, Schipperus MR, Kerkhoffs JL, van der Bom JG. Effect of storage of platelet concentrates in PAS-B, PAS-C, or plasma on transfusion reactions. Transfusion. 2019;59(10):3140–5. doi:10.1111/trf.15497.
- Valkonen S, Mallas B, Impola U, Valkeajärvi A, Eronen J, Javela K, Siljander P-M, Laitinen S. Assessment of time-dependent platelet activation using extracellular vesicles, CD62P exposure, and soluble glycoprotein V content of platelet concentrates with two different platelet additive solutions. Transfus Med Hemother. 2019;46(4):267–75. doi:10.1159/000499958.
- Waters L, Cameron M, Padula MP, Marks DC, Johnson L. Refrigeration, cryopreservation and pathogen inactivation: an updated perspective on platelet storage conditions. Vox Sang. 2018;113(4):317–28. doi:10.1111/vox.12640.
- Magron A, Laugier J, Provost P, Boilard E. Pathogen reduction technologies: the pros and cons for platelet transfusion. Platelets. 2018;29(1):2–8. doi:10.1080/09537104.2017.1306046.
- Osman A, Hitzler WE, Provost P. The platelets’ perspective to pathogen reduction technologies. Platelets. 2018;29(2):140–7. doi:10.1080/09537104.2017.1293806.
- Marschner S, Goodrich R. Pathogen reduction technology treatment of platelets, plasma and whole blood using riboflavin and UV light. Transfus Med Hemother. 2011;38(1):8–18. doi:10.1159/000324160.
- Seghatchian J, Amiral J. Unresolved clinical aspects and safety hazards of blood derived- EV/MV in stored blood components: from personal memory lanes to newer perspectives on the roles of EV/MV in various biological phenomena. Transfus Apher Sci. 2016;55(1):10–22. doi:10.1016/j.transci.2016.07.007.
- Diallo I, Benmoussa A, Laugier J, Osman A, Hitzler WE, Provost P. Platelet pathogen reduction technologies alter the microRNA profile of platelet-derived microparticles. Front Cardiovasc Med. 2020;7:31. doi:10.3389/fcvm.2020.00031.
- Bohonek M, Kutac D, Landova L, Koranova M, Sladkova E, Staskova E, Voldrich M, Tyll T. The use of cryopreserved platelets in the treatment of polytraumatic patients and patients with massive bleeding. Transfusion. 2019;59(S2):1474–8. doi:10.1111/trf.15177.
- Johnson L, Tan S, Wood B, Davis A, Marks DC. Refrigeration and cryopreservation of platelets differentially affect platelet metabolism and function: a comparison with conventional platelet storage conditions. Transfusion. 2016;56(7):1807–18. doi:10.1111/trf.13630.
- Johnson L, Tan S, Jenkins E, Wood B, Marks DC. Characterization of biologic response modifiers in the supernatant of conventional, refrigerated, and cryopreserved platelets. Transfusion. 2018;58(4):927–37. doi:10.1111/trf.14475.
- Johnson L, Coorey CP, Marks DC. The hemostatic activity of cryopreserved platelets is mediated by phosphatidylserine-expressing platelets and platelet microparticles. Transfusion. 2014;54(8):1917–26. doi:10.1111/trf.12578.
- Raynel S, Padula MP, Marks DC, Johnson L. Cryopreservation alters the membrane and cytoskeletal protein profile of platelet microparticles. Transfusion. 2015;55(10):2422–32. doi:10.1111/trf.13165.
- Getz TM, Montgomery RK, Bynum JA, Aden JK, Pidcoke HF, Cap AP. Storage of platelets at 4°C in platelet additive solutions prevents aggregate formation and preserves platelet functional responses. Transfusion. 2016;56(6):1320–8. doi:10.1111/trf.13511.
- Hermida-Nogueira L, García Á. Extracellular vesicles in the transfusion medicine field: The potential of proteomics. Proteomics. 2021;21(13–14):e2000089. doi:10.1002/pmic.202000089.
- Xu Y, Feng K, Zhao H, Di L, Wang L, Wang R. Tumor-derived extracellular vesicles as messengers of natural products in cancer treatment. Theranostics. 2022;12(4):1683–714. Published 2022 Jan 16. doi:10.7150/thno.67775.
- Tkach M, Théry C. Communication by extracellular vesicles: where we are and where we need to go. Cell. 2016;164(6):1226–32. doi:10.1016/j.cell.2016.01.043.
- Hernández A, Arab JP, Reyes D, Lapitz A, Moshage H, Bañales JM, Arrese M. Extracellular vesicles in NAFLD/ALD: from pathobiology to therapy. Cells. 2020;9(4):817. doi:10.3390/cells9040817.
- Brahmer A, Neuberger E, Esch-Heisser L, Haller N, Jorgensen MM, Baek R, Möbius W, Simon P, Krämer-Albers EM. Platelets, endothelial cells and leukocytes contribute to the exercise-triggered release of extracellular vesicles into the circulation. J Extracell Vesicles. 2019;8(1):1615820. doi:10.1080/20013078.2019.1615820.
- Liu L, Deng QJ. Role of platelet-derived extracellular vesicles in traumatic brain injury-induced coagulopathy and inflammation. Neural Regen Res. 2022;17(10):2102–7. doi:10.4103/1673-5374.335825.
- Malenica M, Vukomanović M, Kurtjak M, Masciotti V, Dal Zilio S, Greco S, Lazzarino M, Krušić V, Perčić M, Jelovica Badovinac I, et al. Perspectives of microscopy methods for morphology characterisation of extracellular vesicles from human biofluids. Biomedicines. 2021;9(6):603. doi:10.3390/biomedicines9060603.
- Ferreira PM, Bozbas E, Tannetta SD, Alroqaiba N, Zhou R, Crawley JTB, Gibbins JM, Jones CI, Ahnström J, Yaqoob P. Mode of induction of platelet-derived extracellular vesicles is a critical determinant of their phenotype and function. Sci Rep. 2020;10(1):18061. doi:10.1038/s41598-020-73005-3.
- De Paoli SH, Tegegn TZ, Elhelu OK, Strader MB, Patel M, Diduch LL, Tarandovskiy ID, Wu Y, Zheng J, Ovanesov MV, et al. Dissecting the biochemical architecture and morphological release pathways of the human platelet extracellular vesiculome. Cell Mol Life Sci. 2018;75(20):3781–801. doi:10.1007/s00018-018-2771-6.
- Lamparski HG, Metha-Damani A, Yao JY, Patel S, Hsu DH, Ruegg C, Le Pecq JB. Production and characterization of clinical grade exosomes derived from dendritic cells. J Immunol Methods. 2002;270(2):211–26. doi:10.1016/s0022-1759(02)00330-7.
- Nordin JZ, Lee Y, Vader P, Mäger I, Johansson HJ, Heusermann W, Wiklander OP, Hällbrink M, Seow Y, Bultema JJ, et al. Ultrafiltration with size-exclusion liquid chromatography for high yield isolation of extracellular vesicles preserving intact biophysical and functional properties. Nanomedicine. 2015;11(4):879–83. doi:10.1016/j.nano.2015.01.003.
- Tiwari S, Kumar V, Randhawa S, Verma SK. Preparation and characterization of extracellular vesicles. Am J Reprod Immunol. 2021;85(2):e13367. doi:10.1111/aji.13367.
- Shao H, Im H, Castro CM, Breakefield X, Weissleder R, Lee H. New technologies for analysis of extracellular vesicles. Chem Rev. 2018;118(4):1917–50. doi:10.1021/acs.chemrev.7b00534.
- Shan C, Liang Y, Cai H, Wang F, Chen X, Yin Q, Wang K, Wang Y. Emerging function and clinical significance of extracellular vesicle noncoding RNAs in lung cancer. Mol Ther Oncolytics. 2022;24:814–33. doi:10.1016/j.omto.2022.02.016.
- Daurat A, Roger C, Gris J, Daurat G, Feissel M, Le Manach Y, Lefrant J, Muller L. Apheresis platelets are more frequently associated with adverse reactions than pooled platelets both in recipients and in donors: a study from French hemovigilance data. Transfusion. 2016;56(6):1295–303. doi:10.1111/trf.13475.
- Vogel R, Savage J, Muzard J, Camera GD, Vella G, Law A, Marchioni M, Mehn D, Geiss O, Peacock B, et al. Measuring particle concentration of multimodal synthetic reference materials and extracellular vesicles with orthogonal techniques: who is up to the challenge? J Extracell Vesicles. 2021;10(3):e12052. doi:10.1002/jev2.12052.
- Martin N, Tu X, Egan AJ, Stover C. Complement activation on endothelial cell-derived microparticles-a key determinant for cardiovascular risk in patients with systemic lupus erythematosus? Medicina (Kaunas). 2020;56(10):533. doi:10.3390/medicina56100533.
- Fedorov A, Kondratov K, Kishenko V, Mikhailovskii V, Kudryavtsev I, Belyakova M, Sidorkevich S, Vavilova T, Kostareva A, Sirotkina O, et al. Application of high-sensitivity flow cytometry in combination with low-voltage scanning electron microscopy for characterization of nanosized objects during platelet concentrate storage. Platelets. 2020;31(2):226–35. doi:10.1080/09537104.2019.1599337.
- Vogel R, Pal AK, Jambhrunkar S, Patel P, Thakur SS, Reátegui E, Parekh HS, Saá P, Stassinopoulos A, Broom MF. High-resolution single particle zeta potential characterisation of biological nanoparticles using tunable resistive pulse sensing. Sci Rep. 2017;7(1):17479. doi:10.1038/s41598-017-14981-x.
- Roberts GS, Kozak D, Anderson W, Broom MF, Vogel R, Trau M. Tunable nano/micropores for particle detection and discrimination: scanning ion occlusion spectroscopy. Small. 2010;6(23):2653–8. doi:10.1002/smll.201001129.
- Kurtjak M, Kereïche S, Klepac D, Križan H, Perčić M, Krušić Alić V, Lavrin T, Lenassi M, Wechtersbach K, Kojc N, et al. Unveiling the native morphology of extracellular vesicles from human cerebrospinal fluid by atomic force and cryogenic electron microscopy. Biomedicines. 2022;10(6):1251. doi:10.3390/biomedicines10061251.
- Antwi-Baffour S, Adjei J, Aryeh C, Kyeremeh R, Kyei F, Seidu MA. Understanding the biosynthesis of platelets-derived extracellular vesicles. Immun Inflamm Dis. 2015;3(3):133–40. doi:10.1002/iid3.66.
- Théry C, Witwer KW, Aikawa E, Alcaraz MJ, Anderson JD, Andriantsitohaina R, Antoniou A, Arab T, Archer F, Atkin-Smith GK, et al. Minimal information for studies of extracellular vesicles 2018 (MISEV2018): a position statement of the international society for extracellular vesicles and update of the MISEV2014 guidelines. J Extracell Vesicles. 2018;7(1):1535750. doi:10.1080/20013078.2018.1535750.
- Freitas D, Balmaña M, Poças J, Campos D, Osório H, Konstantinidi A, Vakhrushev SY, Magalhães A, Reis CA. Different isolation approaches lead to diverse glycosylated extracellular vesicle populations. J Extracell Vesicles. 2019;8(1):1621131. doi:10.1080/20013078.2019.1621131.
- Ekström K, Crescitelli R, Pétursson HI, Johansson J, Lässer C, Olofsson Bagge R. Characterization of surface markers on extracellular vesicles isolated from lymphatic exudate from patients with breast cancer. BMC Cancer. 2022;22(1):50. doi:10.1186/s12885-021-08870-w.
- Sobolewska A, Włodarczyk M, Stec-Michalska K, Fichna J, Wiśniewska-Jarosińska M. Mean platelet volume in crohn’s disease patients predicts sustained response to a 52-week infliximab therapy: a pilot study. Dig Dis Sci. 2016;61(2):542–9. doi:10.1007/s10620-015-3894-3.
- Heijnen HF, Schiel AE, Fijnheer R, Geuze HJ, Sixma JJ. Activated platelets release two types of membrane vesicles: microvesicles by surface shedding and exosomes derived from exocytosis of multivesicular bodies and alpha-granules. Blood. 1999;94(11):3791–9. doi:10.1182/blood.V94.11.3791.
- Dean WL, Lee MJ, Cummins TD, Schultz DJ, Powell DW. Proteomic and functional characterisation of platelet microparticle size classes. Thromb Haemost. 2009;102(4):711–8. doi:10.1160/TH09-04-243.
- Marcoux G, Laroche A, Hasse S, Bellio M, Mbarik M, Tamagne M, Allaeys I, Zufferey A, Lévesque T, Rebetz J, et al. Platelet EVs contain an active proteasome involved in protein processing for antigen presentation via MHC-I molecules. Blood. 2021;138(25):2607–20. doi:10.1182/blood.2020009957.
- Guo SC, Tao SC, Yin WJ, Qi X, Yuan T, Zhang CQ. Exosomes derived from platelet-rich plasma promote the re-epithelization of chronic cutaneous wounds via activation of YAP in a diabetic rat model. Theranostics. 2017;7(1):81–96. Published 2017 Jan 1. doi:10.7150/thno.16803.
- Intini G, Andreana S, Intini FE, Buhite RJ, Bobek LA. Calcium sulfate and platelet-rich plasma make a novel osteoinductive biomaterial for bone regeneration. J Transl Med. 2007;5(1):13. doi:10.1186/1479-5876-5-13.
- Spakova T, Janockova J, Rosocha J. Characterization and therapeutic use of extracellular vesicles derived from platelets. Int J Mol Sci. 2021;22(18):9701. doi:10.3390/ijms22189701.
- Jain A, Marwaha N, Sharma RR, Kaur J, Thakur M, Dhawan HK. Serial changes in morphology and biochemical markers in platelet preparations with storage. Asian J Transfus Sci. 2015;9(1):41–7. doi:10.4103/0973-6247.150949.
- Black A, Pienimaeki-Roemer A, Kenyon O, Orsó E, Schmitz G. Platelet-derived extracellular vesicles in plateletpheresis concentrates as a quality control approach. Transfusion. 2015;55(9):2184–96. doi:10.1111/trf.13128.
- Price J, Gardiner C, Harrison P. Platelet-enhanced plasma: Characterization of a novel candidate resuscitation fluid’s extracellular vesicle content, clotting parameters, and thrombin generation capacity. Transfusion. 2021;61(7):2179–94. doi:10.1111/trf.16423.
- Frank J, Richter M, de Rossi C, Lehr CM, Fuhrmann K, Fuhrmann G. Extracellular vesicles protect glucuronidase model enzymes during freeze-drying. Sci Rep. 2018;8(1):12377. doi:10.1038/s41598-018-30786-y.
- Chatterjee V, Yang X, Ma Y, Wu MH, Yuan SY. Extracellular vesicles: new players in regulating vascular barrier function. Am J Physiol Heart Circ Physiol. 2020;319(6):H1181–H96. doi:10.1152/ajpheart.00579.2020.
- Zubairova LD, Nabiullina RM, Nagaswami C, Zuev YF, Mustafin IG, Litvinov RI, Weisel JW. Circulating microparticles alter formation, structure, and properties of fibrin clots. Sci Rep. 2015;5(1):17611. doi:10.1038/srep17611.
- Sinauridze EI, Kireev DA, Popenko NY, Pichugin AV, Panteleev MA, Krymskaya OV, Ataullakhanov FI. Platelet microparticle membranes have 50- to 100-fold higher specific procoagulant activity than activated platelets. Thromb Haemost. 2007;97(3):425–34. doi:10.1160/th06-06-0313.
- Ceroi A, Delettre FA, Marotel C, Gauthier T, Asgarova A, Biichlé S, Duperrier A, Mourey G, Perruche S, Lagrost L, et al. The anti-inflammatory effects of platelet-derived microparticles in human plasmacytoid dendritic cells involve liver X receptor activation. Haematologica. 2016;101(3):e72–e6. doi:10.3324/haematol.2015.135459.
- Johnson J, Wu YW, Blyth C, Lichtfuss G, Goubran H, Burnouf T. Prospective therapeutic applications of platelet extracellular vesicles. Trends Biotechnol. 2021;39(6):598–612. doi:10.1016/j.tibtech.2020.10.004.
- Puhm F, Boilard E, Machlus KR. Platelet extracellular vesicles: beyond the blood. Arterioscler Thromb Vasc Biol. 2021;41(1):87–96. doi:10.1161/ATVBAHA.120.314644.
- Yasui K, Matsuyama N, Kuroishi A, Tani Y, Furuta RA, Hirayama F. Mitochondrial damage-associated molecular patterns as potential proinflammatory mediators in post-platelet transfusion adverse effects. Transfusion. 2016;56(5):1201–12. doi:10.1111/trf.13535.
- Fletcher CH, DomBourian MG, Millward PA. Platelet transfusion for patients with cancer. Cancer Control. 2015;22(1):47–51. doi:10.1177/107327481502200107.
- Stanworth SJ, Hudson CL, Estcourt LJ, Johnson RJ, Wood EM. TOPPS study investigators. Risk of bleeding and use of platelet transfusions in patients with hematologic malignancies: recurrent event analysis. Haematologica. 2015;100(6):740–7. doi:10.3324/haematol.2014.118075.
- Anene C, Graham AM, Boyne J, Roberts W. Platelet microparticle delivered microRNA-Let-7a promotes the angiogenic switch. Biochim Biophys Acta Mol Basis Dis. 2018;1864(8):2633–43. doi:10.1016/j.bbadis.2018.04.013.
- Goubran H, Sabry W, Kotb R, Seghatchian J, Burnouf T. Platelet microparticles and cancer: an intimate cross-talk. Transfus Apher Sci. 2015;53(2):168–72. doi:10.1016/j.transci.2015.10.014.
- Sadallah S, Schmied L, Eken C, Charoudeh HN, Amicarella F, Schifferli JA. Platelet-derived ectosomes reduce NK cell function. J Immunol. 2016;197(5):1663–71. doi:10.4049/jimmunol.1502658.
- Park M, Kang KW. Phosphatidylserine receptor-targeting therapies for the treatment of cancer. Arch Pharm Res. 2019;42(7):617–28. doi:10.1007/s12272-019-01167-4.
- Labelle M, Begum S, Hynes RO. Direct signaling between platelets and cancer cells induces an epithelial-mesenchymal-like transition and promotes metastasis. Cancer Cell. 2011;20(5):576–90. doi:10.1016/j.ccr.2011.09.009.
- Janowska-Wieczorek A, Wysoczynski M, Kijowski J, Marquez-Curtis L, Machalinski B, Ratajczak J, Ratajczak MZ. Microvesicles derived from activated platelets induce metastasis and angiogenesis in lung cancer. Int J Cancer. 2005;113(5):752–60. doi:10.1002/ijc.20657.
- Tao SC, Guo SC, Zhang CQ. Platelet-derived extracellular vesicles: an emerging therapeutic approach. Int J Biol Sci. 2017;13(7):828–34. doi:10.7150/ijbs.19776.
- Reesink HW, Lee J, Keller A, Dennington P, Pink J, Holdsworth R, Schennach H, Goldman M, Petraszko T, Sun J, et al. Measures to prevent transfusion-related acute lung injury (TRALI). Vox Sang. 2012;103(3):231–59. doi:10.1111/j.1423-0410.2012.01596.x.
- Wagner T, Vetter A, Dimovic N, Guber SE, Helmberg W, Kröll W, Lanzer G, Mayr WR, Neumüller J. Ultrastructural changes and activation differences in platelet concentrates stored in plasma and additive solution. Transfusion. 2002;42(6):719–27. doi:10.1046/j.1537-2995.2002.00125.x.
- Mowla SJ, Kracalik IT, Sapiano MRP, O’Hearn L, Andrzejewski CJ, Basavaraju SV. A comparison of transfusion-related adverse reactions among apheresis platelets, whole blood-derived platelets, and platelets subjected to pathogen reduction technology as reported to the national healthcare safety network hemovigilance module. Transfus Med Rev. 2021;35(2):78–84. doi:10.1016/j.tmrv.2021.03.003.