Abstract
The contribution of platelets is well recognized in thrombosis and hemostasis. However, platelets also promote tumor progression and metastasis through their crosstalk with various cells of the tumor microenvironment (TME). For example, several cancer models continue to show that platelet functions are readily altered by cancer cells upon activation leading to the formation of platelet-tumor aggregates, triggering release of soluble factors from platelet granules and altering platelet turnover. Further, activated platelets protect tumor cells from shear forces in circulation and assault of cytotoxic natural killer (NK) cells. Platelet-secreted factors promote proliferation of malignant cells, metastasis, and chemoresistance. Much of our knowledge of platelet biology in cancer has been achieved with animal models, particularly murine. However, this preclinical understanding of the complex pathophysiology is yet to be fully realized and translated to clinical trials in terms of new approaches to treat cancer via controlling the platelet function. In this review, we summarize the current state of knowledge of platelet physiology obtained through existing in vivo and in vitro cancer models, the complex interactions of platelets with cancer cells in TME and the pathways by which platelets may confer chemoresistance. Since the FDA Modernization Act recently passed by the US government has made animal models optional in drug approvals, we critically examine the existing and futuristic value of employing bioengineered microphysiological systems and organ-chips to understand the mechanistic role of platelets in cancer metastasis and exploring novel therapeutic targets for cancer prevention and treatment.
Plain Language Summary
The recent passage of the FDA Modernization Act by the US government has removed the requirement of the use of animal models in disease modeling and drug discovery process. This has resulted in a much-renewed excitement within engineers, scientists, and industry in applying in vitro cell biosystems as a platform technology that assists or replaces animals in reproducing human biology. The contribution of platelets is well recognized in thrombosis and hemostasis. However, platelets also promote tumor progression and metastasis through their crosstalk with various cells of the tumor microenvironment. In this review, we summarize the current state of knowledge of platelet physiology obtained through existing cancer models and also critically examine the existing and futuristic value of employing bioengineered organ-chips to improve the knowledge of the underlying biology.
Introduction
Platelets are anucleate discoid blood cells known to regulate hemostasis and thrombosis.Citation1 The association between thrombosis and cancer has long been recognized, with thromboembolic complications often preceding cancer diagnosis.Citation2 Thromboembolism commonly occurs during cancer progression or as a complication of oncologic treatment.Citation3 Thrombocytosis, an elevated platelet count, is frequently observed in 10% of breast cancer cases to as high as 55% of lung and colorectal cancer patients can promote cancer growth and metastasis.Citation3,Citation4 Increased platelet count is correlated with poor prognosis in various cancers, including colon, lung, gastric, renal, prostatic, cervical, endometrial, and ovarian cancer.Citation5–10 Pretreatment of thrombocytosis is a negative prognostic factor associated with aggressive tumor behavior in cervical, colon, and non-small cell lung cancer.Citation11,Citation12 Conversely, thrombocytopenia, a decreased platelet count, is associated with worse prognosis in patients with bone marrow, spleen, or pancreatic tumors.Citation13 Thus, platelets play a complex role in disease progression and metastasis depending on the cancer type and stageCitation14 (). Platelets contribute to tumor growth, migration, and metastasis by promoting angiogenesisCitation15 through the transport of proangiogenic factors like vascular endothelial growth factor (VEGF). Recent clinical studies also indicate a strong correlation between platelet count and tumor chemoresistanceCitation16 which is in agreement with in vitro studies (). Platelets have been extensively studied for their systemic effects on tumor angiogenesis, progression, and metastasis in cancer patients. However, the direct involvement of platelets in TME remains poorly understood. This is partly because animal studies have some limitations as preclinical model systems in being able to dissect specific signaling pathways and offer simplicity in analyzing individual cells or cell–drug interactions. Recent advancements in organ-on-chip microdevices offer a controlled platform to model the human TME and study its interactions with platelets. These bioengineered organ-chip technologies use human cells to create miniaturized models of specific stages of cancer, providing real-time information on tumor progression and blood cell interactions. This review article focuses on the strengths and limitations of organ-chips as a complementary platform to enhance our understanding of platelet biology in cancer. It also explores how platelet function can be modeled with these systems in different stages of tumor progression and highlights the potential of combining anti-cancer and anti-platelet therapies as a strategy to treat cancer.
Figure 1. Contribution of platelets in cancer. Platelets get activated by soluble factors and chemokines present in the tumor microenvironment and play a vital role in cancer progression. Platelets play crucial role in supporting cancer growth by inducing stable angiogenesis and neovascularization. They also promote epithelial to mesenchymal transition of tumor cells thereby facilitating metastasis. High platelet counts contribute to chemoresistance by countering the inhibitory effect of chemotherapeutics thereby facilitating tumor cell proliferation and disease spread. Created with BioRender.com.
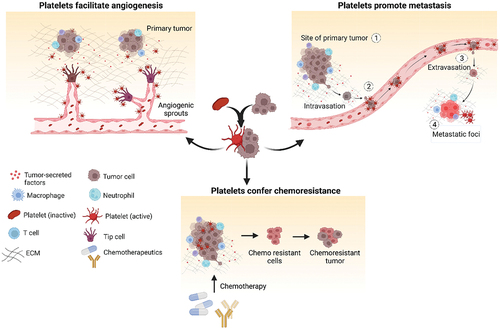
Platelet functions in cancer
Vascular stress and angiogenesis
Inflammation is one of the determinants of morbidity and mortality of cancer patients.Citation17 Inflammatory molecules and platelets have reciprocal signaling transduction and mechanisms, ultimately triggering thrombi formation, leukocyte recruitment, endothelial activation, and angiogenesis in the tumor microenvironment.Citation18 Various cell adhesion molecules (CAMs) and integrins like ICAM-1, VCAM-1, LFA-1, and Mac-1 facilitate adhesion of leukocytes to endothelial cells (ECs) through P-selectin and CD40L receptors disrupting vascular integrity and promoting platelet activation and adhesion.Citation19 Activated platelets interact with leukocytes and eventually induce thrombus formation.Citation20 P-selection present on both ECs and platelets contributes to tumor progressionCitation21 and metastasis.Citation22 Cancer cells stimulate platelets to promote angiogenesis for preserving integrity of leaky blood vessels.Citation23 Stored proangiogenic factors like VEGF, FGF, EGF, and anti-angiogenic factors namely ANGPT1, S1P, TSP1, and endostatinCitation24 are released by platelets based on signals from the tumor niche.Citation25 A xenograft breast cancer model showed that platelets sequester cancer cell-secreted cytokines and deliver them to tumor sites, promoting angiogenesis.Citation26 Tumor-derived VEGF activates ECs, induces platelet aggregation, and promotes thrombin generation thus facilitating angiogenesis.Citation27 Platelet accumulation on activated endothelial surfaces enhances EC survival and migration.Citation28 Cancer patient-derived platelet microparticles (PMPs) contain growth factors, cytokines, and inflammatory molecules aiding angiogenesis.Citation29,Citation30 These PMPs carry microRNAs contributing to neovascularization and enhance platelet-dependent endothelial tube formation thus inhibiting Thrombospondin-1 (TSP1) and increasing tumorigenicity. Platelets play dual role in tumor progression. They promote pathological angiogenesis while being involved in tumor inhibition by blood vessel normalization. Platelets recruit and mature endothelial progenitor cells, support pericyte coverage and release factors like angiopoietin-1 and serotonin to promote vessel maturation.Citation31 Reduced vessel density and impaired vessel maturation were observed in tumors from thrombocytopenic mice.Citation32,Citation33 Initially, platelets are recruited to restore vessel integrity in TME but upon activation by tumor-released factors, initiate pathological angiogenesis. Further research is needed to understand the specific signaling cascades, conditions, and events favoring platelet-induced angiogenesis.
Metastasis
Cancer metastasis involves complex interactions between cancer cells and the host.Citation34 Only small fraction of circulating tumor cells (CTCs) survive the high shear in circulation evading immune system to form metastatic foci.Citation35 Platelets are the first to interact and protect CTCs from natural killer (NK) cells via thrombi formation.Citation36,Citation37 Platelet count is an influential factor in cancer metastasis. The condition of elevated platelet count, thrombocytosis, is associated with enhanced pro-metastatic functions resulting in increased tumor metastasis and poor prognosis in lung, ovarian, and colorectal cancers.Citation38,Citation39 Conversely, induced thrombocytopenia, a low platelet count, or impaired platelet activation in preclinical models have shown reduced metastatic ability of primary tumors.Citation33,Citation40 Activated platelets in association with fibrinogen mask cancer cells from immune surveillance by transferring major histocompatibility class I complex (MHC 1) to tumor cells and preventing cytolysis by cytotoxic NK cells.Citation41,Citation42 Platelet-mediated shedding of Natural Killer Group 2D (NKG2D) ligands compromises tumor cell recognition by NK cells, facilitating immune evasion.Citation43 Tumor cells release factors like ADP, thromboxane A2 (TXA2), and high-mobility group box 1 (HMGB1)Citation44 activate platelets resulting in granule secretion, ATP release, and endothelial junction disruption. Tissue factor (TF) expressed on cancer cell membranes,Citation45 tumor cell-derived matrix metalloproteinases (MMPs) and TF detected on tumor-derived vesiclesCitation46 can also induce platelet activation. Platelets also play a crucial role in tumor growth and metastasis by releasing transforming growth factor-β (TGF-β), which suppresses immune responses creating an immunosuppressive TME.Citation47 Platelets secreted TGF-β downregulates NKG2D receptor on NK cells, inhibiting anti-tumor activity. Elevated TGF-β levels are associated with reduced NKG2D expression in lung cancer patients.Citation48 Labelle et al. showed that the inhibition of TGFβ1 expression in platelets and megakaryocytes prevents metastasis and lung colonization by tumor cells.Citation49 Forming thrombi, platelets confer anoikis (homelessness, form of programmed cell death) resistance to CTCs by releasing growth factors, cytokines, and allowing integrin αIIbβ3 receptors to interact with cancer cell integrins, enabling survival, proliferation, and colonization of tumor in distant organs. Platelets activate signaling pathways promoting apoptosis resistance, nuclear translocation of YAP1 and metastasis.Citation49–51 Labelle and Hynes demonstrated that platelets and granulocytes are sequentially recruited to disseminated tumor cells forming “early metastatic niches.” Inhibiting platelet–granulocyte interactions could be a potential therapy for preventing metastasis alongside targeting tumor cells.Citation52 However, limited knowledge of platelet supporting tumor cells at secondary sites and CTC survival mechanisms exists and needs further investigation.
Resistance to therapy
Chemotherapy resistance is a major challenge in cancer treatment resulting in relapse and metastasis. Tumors that initially respond to chemotherapy can develop resistance and continue growth.Citation53 Chemoresistance is associated with an increased risk of thromboembolic disorders in patients.Citation54 Studies have shown a strong correlation between platelet count and tumor chemoresistance,Citation53 where higher platelet counts contribute to resistance against drugs like paclitaxel and 5-fluorouracilCitation55 and decreased platelet counts enhanced effectiveness of drugs like doxorubicin and paclitaxel.Citation56,Citation57 Glycoprotein VI (GPVI) on platelet surface is responsible for tumor inhibition, and using antibody fragment (JAQ1 F(ab)2) to inhibit GPVI has shown promising result in reducing tumor growth and enhancing accumulation of Doxil and paclitaxel.Citation56 Focal adhesion kinase (FAK) regulates platelet migration into TME, while FAK-deficient platelets prevent tumor relapse upon discontinuation of anti-angiogenic therapy.Citation58 Soluble factors released by activated platelets alter the balance between anti-apoptotic and pro-apoptotic genes, counteract cell cycle arrest induced by anti-cancer agents, promote tumor growth, enhance phosphorylation of DNA repair proteins like Chk1, BRCA1, and Mre11, and inhibit the cytotoxic effects of drugs like sorafenib and regorafenib.Citation59 Platelets being the primary source of TGF-β1 in blood induce epithelial–mesenchymal transition, a process associated with tumor progression and metastasis.Citation49 Recent studies have described the unique roles of platelets after withdrawal of anti-angiogenic therapy, followed by antiangiogenic effects of aspirin, and predicted that anti-angiogenesis escape by tumors may be arrested by investigating mechanisms and combining other drugs.Citation58,Citation60,Citation61 Therefore, preclinical models will be beneficial in exploring the potential of combining antiplatelet drugs with chemotherapeutic and antiangiogenic agents to arrest cancer metastasis and overcome chemoresistance. Such combinatorial approaches could offer new therapeutic strategies to improve clinical outcomes for cancer patients.
Microphysiological systems as contemporary models of platelet biology in cancer
Murine models are considered as trustworthy preclinical model systems to predict human biology, as they have majorly contributed to our current understanding of platelet biology in cancer and have shaped numerous successful clinical trials. But along with their strengths, there exists some limitations. For example, in many cases, murine physiology does not translate to humans directly. Secondly, they are complex and while providing a system-level response to stressors and drugs, cannot be dissected for studying individual signaling pathways or describe cell-cell interactions with high specificity. To complement these models, microphysiological systems, also termed as organ-on-chips (OoC), have provided an in vitro cell culture system by closely mimicking human physiology, enabling researchers to gain meaningful insights into disease states and underlying mechanisms. As an example, these devices replicate blood flow and allow integration of multiple cell types, mirroring the cellular diversity found in organs. In cancer research, OoC technology has spurred investigations in cancer metastasis,Citation62 intravasation,Citation63 extravasation,Citation64,Citation65 and even angiogenesis.Citation66 Studies have previously shown how OoC can be used to perform quantitative analysis of vascular dysfunction, platelet hyperactivity, and thrombosis.Citation67–69 But recently, studies conducted by Saha et. al. using microfluidic OoC technology revealed that compromised vessel integrity in ovarian TME facilitate extravasation of platelets toward cancer.Citation70 This study revealed similar behaviors of platelets as observed during cancer progression. Soluble factors released from tumor chamber activated the platelets stimulating movement toward cancer-influenced blood endothelial cells, and finally extravasating to the tumor chamber. The data also suggest statins to be a potential drug in preventing cancer growth, partially by blocking direct contact with plateletsCitation70 (). Another study by the same group reported successful development of ovarian tumor microenvironment chip (OTME-chip) () demonstrating mechanisms through which platelets promote metastasis and highlighting a novel drug target to augment chemotherapeutic response.Citation71 The study revealed that interaction of platelet GPVI with tumor cell expressing galectin-3 under shear in time-dependent manner results in upregulation of numerous growth factors inducing metastasis. The metastatic potential of tumor was diminished resulting in improved chemosensitivity on administration of combinatorial composition of chemotherapeutic agent with anti-platelet drug.Citation71 Gene-edited tumors and next generation RNA-seq-on-chip performed in this study revealed temporal dynamics of vascular disintegration (), making the vascular tissue leaky and promoting platelet extravasation () in ovarian cancer. Study reported by Crippa et. al. designed human early metastatic niche (EMN)-on-a-chip to mimick transient microenvironment forms in circulation during cancer dissemination and understand the crosstalk among cancer, platelets, leukocytes, and ECs. EMN-on-a-chip exhibited increased transendothelial migration of cancer cells in presence of neutrophils and platelets, along with enhanced expression of epithelial to mesenchymal transition (EMT) markers in cancer cells under the influence of platelets. Use of antiplatelet drugs have demonstrated effectiveness in reducing platelet aggregation and lowering EMT markers.Citation72 These emerging examples of organ-chips integrating blood and vascular components within TME demonstrate the positive value of these approaches in cancer research and drug discovery. By studying the interactions between different cell types in an organ-chip, researchers can gain a deeper understanding of diseases and develop more effective treatment strategies. While not strictly organ-chips, researchers have also developed in vitro microfluidic assays that enable real-time observation and quantification of critical steps in metastasis, such as tumor cell extravasation. These assays feature perfusable microvascular networks, allowing the study of tumor cell dynamics, morphological changes, and screening of therapeutic agents.Citation64 Additionally, the isolation of platelets or leukocytes from human blood and their perfusion through the device with tumor cells enables the investigation of tumor-immune cell interactions independently of other cell types.Citation64 This reductionist approach in the in vitro system offers advantages in studying specific interactions that are challenging to perform in vivo. For example, Papa et al. demonstrated the effects of platelet decoys on platelet adhesion on exposing to physiological flow using microfluidic devices with collagen-coated channels. Platelet decoys mixed with normal platelets partially inhibited platelet adhesion. The antiplatelet effect was reversible upon adding fresh platelets, while the clinical antiplatelet agent abciximab showed a sustained effect.Citation73 In cancer, platelets are activated by inflammatory cytokines released from tumor cells, contributing to the thrombi formation in the presence of procoagulant-like tissue factor also released by tumor cells.Citation34,Citation74 This phenomenon, known as tumor-associated thrombosis, is a common complication in cancer patients. Since platelets are the drivers of thrombosis, cancer-associated thrombosis is also a complex physiology where organ-chips can make significant contributions. Organ-on-a-chip systems utilize microfluidic flow chambers to include endothelial lumens and enable the perfusion of whole blood, allowing visualization of platelet-endothelial interactions.Citation69 These microphysiological models of thrombosis can mimic the composition and behavior of thrombi in different blood vessels by perfusing whole blood at high arterial and low venous wall shear rates.Citation75 They accurately model anatomical structures observed in vivo like plaques, bifurcations and incorporate vascular cells, enhancing the physiological relevance of thrombosis studies in various diseases.Citation76
Figure 2. Organ-on-chip model of ovarian cancer vessel–platelet interaction, vascular degradation, metastasis and RNA-sequencing data analysis. (a) Schematic showing OvCa-chip components and assembly in detail. (b) Microscopic images showing human ovarian cancer cells, A2780 cultured in the upper microchannel (top) of OvCa-chip (center) and endothelial vessel formed in lower channel (bottom). Bars represent 100 µm. (c) Fluorescence micrographs displaying cross sectional view of the OvCa-chip with A2780 (red, anti-ZO1) cocultured with primary endothelial cells (green, VE-cadherin) to form a blood-perfusing vessel. The tumor-vascular tissue interface distance of cross-sectional 3D view is 10 µm. Bars represent 100 µm. (d) Immunohistochemical images of tumor biopsies obtained from healthy individual and patient with ovarian cancer showing platelets extravasation (CD42b+, brown and marked by arrows) into the tumor stroma in patients with and without statin treatment. Scale bar = 50 µm. Insets: mag = 40×. (e) Quantification of platelets observed with histology. Error bars are means ± SEM; n = 5. (f) Computer aided design of OTME-chip showing two PDMS compartments separated by thin porous membrane reproducing the microarchitecture of tumor-vascular interface. (g) OTME-Chip schematic depicting the influence of extravasated platelets in tumor invasion dynamics. (h) Cross-sectional 3D confocal view of OTME-Chip representing cancer cells (yellow), endothelial cells (red), and platelets (cyan) at 0 hours (left) and 72 hours (right) after platelet extravasation. Scale bars, 100 μm. (i) Volcano plots exhibiting differentially expressed genes in OTME-Chip, Cx-OTME-Chip, CxRx-OTME-Chip, and KO-OTME-Chip respectively relative to control (black dots). Red dots mark the genes regulating EMT and metastasis. (j) KEGG pathway clustering shows differential presence of EMT regulatory pathways for all study groups relative to control-chip (P < .05). Adapted with permissionCitation70 Copyright 2020, ASH publications and copyright 2021, Science publishing.
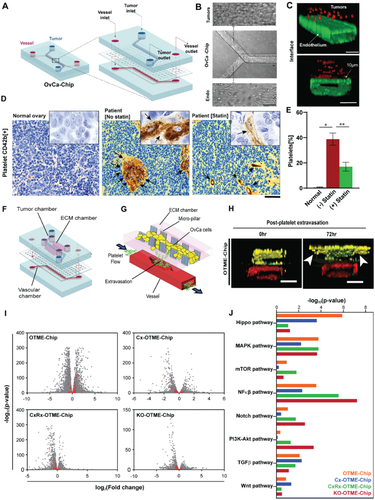
While there are no visible organ chip studies modeling thrombosis in cancer, it has been shown that tumor cells can induce platelet activation and aggregation, leading to microthrombus formation and impaired blood flow within micro-vessels.Citation77 Such findings highlight the potential of organ-on-a-chip platforms to simulate tumor-induced platelet activation and thrombosis, providing insights into underlying mechanisms and facilitating the development of targeted therapeutic interventions. The use of organ-chips and other microfluidic technologies together provide an opportunity to bridge the gap between traditional cell culture and in vivo models, offering a useful representation of human physiology and advancing our knowledge of disease mechanisms. They may provide significant scope to easily visualize and spatiotemporally control specific biological processes in detail to gain a better understanding of the disease progression and employing the platform for drug testing application.Citation71,Citation78 Thus, with more advancement, OoC can evolve as a new in vitro dissectible platform to model the mechanisms of cancer-vascular-hematology nexus and analyze as well as predict personalized therapeutics toward disease management ().
Figure 3. Importance of organ-on-chip (OoC) technologies in platelet cancer research. OoCs are the next generation modeling system facilitating in vitro disease modeling by closely mimicking the in vivo conditions in humans. This tool provides a dissectible platform by introducing flow mechanism and interaction of multiple cell types in real time by forming microchambers. OoC technology can be widely employed to better understand the role of platelets in vascular stress, angiogenesis and metastasis in cancer as well as finding new therapeutic targets and personalized therapeutic strategy for better disease management. Created with BioRender.com.
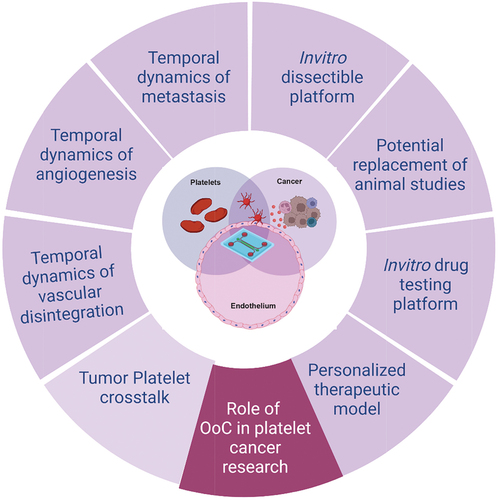
Limitations of microphysiological systems and future perspective
Platelets play a crucial role in tumor progression and metastasis by interacting with other cells in circulation and stimulating signaling pathways affecting tumor cells and TME, contributing to angiogenesis, immunoevasion, metastasis, and thrombosis. Targeting platelet-cancer cell interaction is a potential strategy to reduce metastasis and cancer-associated thrombosis, although clinical trials with antiplatelet agents like prasugrel, ticagrelor, or vorapaxar along with aspirin have yielded inconsistent results.Citation79 Further research exploring platelet contribution at different stages of cancer, the balance between pro- and anti-tumor activities, involved signaling pathways and molecular variants are necessary. Complex microphysiological models continue to evolve aiming to mimic in vivo conditions closely to explore the unanswered role of platelets in cancer (). However, these models still have limitations in studying the systemic effects of cancer and platelet evolution as the disease progresses. Despite promising advantages, OoC models are low throughput. Some progress has been made in developing high-throughput systems by parallelizing multiple culture chambers in a single device, allowing studies with numerous replicates.Citation80 High drug absorption by polydimethylsiloxane (PDMS) in OoC devices is a concern, thus not recapitulating in vivo pharmacokinetics. Use of biologics, non-absorptive coatings,Citation81 alternative materials like polyurethanes and polycarbonate-thermoplastic elastomer hybrids,Citation82 and non-absorptive rigid thermoplastics like polystyrene or polycarbonateCitation83 can mitigate this challenge. Organ-on-chips are yet to meet the regulatory benchmarks set by the IQ Consortium and the FDA to replace animal studies.Citation84 Therefore, at the moment, organ-on-chips and animal models can cooperatively leverage their strengths and cross-validate preclinical findings. Despite recent advancements, challenges in microfluidic device designs for modeling thrombosis include channel shape, setup costs, limited 3D capability, and turbulence creation. Advances in 3D printing hold promise for personalized models using patient-derived tissues and stem cellsCitation69,Citation85 aiming to mimic pathophysiology of thrombosis, predict therapeutic responses, improve patient health outcomes, and reduce costs. Finally, organ-on-a-chip platforms focus on local effects within specific tissue or organ. For example, they may lack the inclusion of the bone marrow, the primary site of platelet production and maturation. Therefore, the systemic effects of cancer involving interactions with other organs and dynamic changes in platelet biology may not be fully understood with these systems. Nevertheless, organ-on-chips provide a necessary toolbox to study platelet behavior in context of cancer by co-culturing cancer cells and ECs, investigating platelet activation, aggregation, and prothrombotic properties within TME. Integrating multiple models including bone marrow-on-a-chip can furnish a comprehensive understanding of platelet evolution in cancer, bridging the gap between local and systemic effects. Overall, the future prospects of learning platelet physiology in cancer appear to be bright, and microphysiological systems may serve a critical role alongside animal models in deriving new therapeutic and preventive strategies against cancer.
Disclosure statement
No potential conflict of interest was reported by the author(s).
Additional information
Funding
References
- de Gaetano G. Historical overview of the role of platelets in hemostasis and thrombosis. Haematologica. 2001;86(4):349–8.
- Francis JL, Biggerstaff J, Amirkhosravi A. Hemostasis and malignancy. Semin Thromb Hemost. 1998;24(2):93–109. doi:10.1055/s-2007-995829.
- Wojtukiewicz MZ, Sierko E, Kisiel W. The role of hemostatic system inhibitors in malignancy. Semin Thromb Hemost. 2007;33(7):621–642. doi:10.1055/s-2007-991530.
- Rickles FR, Levine M, Edwards RL. Hemostatic alterations in cancer patients. Cancer Metastasis Rev. 1992;11(3–4):237–248. doi:10.1007/BF01307180.
- Symbas NP, Townsend MF, El‐Galley R, Keane TE, Graham SD, Petros JA. Poor prognosis associated with thrombocytosis in patients with renal cell carcinoma. BJU Int. 2000;86(3):203–207. doi:10.1046/j.1464-410x.2000.00792.x.
- Ikeda M, Furukawa H, Imamura H, Shimizu J, Ishida H, Masutani S, Tatsuta M, Satomi T. Poor prognosis associated with thrombocytosis in patients with gastric cancer. Ann Surg Oncol. 2002;9(3):287–291. doi:10.1007/BF02573067.
- Stone RL, Nick AM, McNeish IA, Balkwill F, Han HD, Bottsford-Miller J, Rupairmoole R, Armaiz-Pena GN, Pecot CV, Coward J, et al. Paraneoplastic thrombocytosis in ovarian cancer. N Engl J Med. 2012;366(7):610–618. doi:10.1056/NEJMoa1110352.
- Cheng J, Zeng Z, Ye Q, Zhang Y, Yan R, Liang C, Wang J, Li M, Yi M. The association of pretreatment thrombocytosis with prognosis and clinicopathological significance in cervical cancer: a systematic review and meta-analysis. Oncotarget. 2017;8(15):24327–24336. doi:10.18632/oncotarget.15358.
- Jósa V, Krzystanek M, Vass T, Lang T, Juhász V, Szilágyi K, Tihanyi B, Harsányi L, Szállási Z, Salamon F, et al. Thrombocytosis of liver metastasis from colorectal cancer as predictive factor. Pathol Oncol Res. 2015;21(4):991–997. doi:10.1007/s12253-015-9925-8.
- Barlow M, Hamilton W, Ukoumunne OC, Bailey SER. The association between thrombocytosis and subtype of lung cancer: a systematic review and meta-analysis. Transl Cancer Res. 2021;10(3):1249–1260. doi:10.21037/tcr-20-3287.
- Wang YH, Deng SJ, Yang YD, Yao N, Zhao JM, Min GT, Wang J, Xu TF, Zhao PY, Wang HP, et al. The pretreatment thrombocytosis may predict prognosis of patients with colorectal cancer: a systematic review and meta-analysis. Biomark Med. 2017;11(2):195–210. doi:10.2217/bmm-2016-0214.
- Gao L, Zhang H, Zhang B, Zhang L, Wang C. Prognostic value of combination of preoperative platelet count and mean platelet volume in patients with resectable non-small cell lung cancer. Oncotarget. 2017;8(9):15632–15641. doi:10.18632/oncotarget.14921.
- Liebman HA. Thrombocytopenia in cancer patients. Thromb Res. 2014;133(Suppl 2):S63–S69. doi:10.1016/S0049-3848(14)50011-4.
- Honn KV, Tang DG, Crissman JD. Platelets and cancer metastasis: a causal relationship? Cancer Metastasis Rev. 1992;11(3–4):325–351. doi:10.1007/BF01307186.
- Wojtukiewicz MZ, Sierko E, Klement P, Rak J. The hemostatic system and angiogenesis in malignancy. Neoplasia. 2001;3(5):371–384. doi:10.1038/sj.neo.7900184.
- Yu L, Guo Y, Chang Z, Zhang D, Zhang S, Pei H, Pang J, Zhao ZJ, Chen Y. Bidirectional interaction between cancer cells and platelets provides potential strategies for cancer therapies. Front Oncol. 2021;11:764119. doi:10.3389/fonc.2021.764119.
- Varki A. Trousseau’s syndrome: multiple definitions and multiple mechanisms. Blood. 2007;110(6):1723–1729. doi:10.1182/blood-2006-10-053736.
- Zuchtriegel G, Uhl B, Puhr-Westerheide D, Pörnbacher M, Lauber K, Krombach F, Reichel CA, Bhandoola A. Platelets guide leukocytes to their sites of extravasation. PLoS Biol. 2016;14(5):e1002459. doi:10.1371/journal.pbio.1002459.
- Thomas MR, Storey RF. The role of platelets in inflammation. Thromb Haemost. 2015;114(3):449–458. doi:10.1160/TH14-12-1067.
- Palabrica T, Lobb R, Furie BC, Aronovitz M, Benjamin C, Hsu YM, Sajer SA, Furie B. Leukocyte accumulation promoting fibrin deposition is mediated in vivo by P-selectin on adherent platelets. Nature. 1992;359(6398):848–851. doi:10.1038/359848a0.
- da Costa Martins PA, van Gils JM, Mol A, Hordijk PL, Zwaginga JJ. Platelet binding to monocytes increases the adhesive properties of monocytes by up-regulating the expression and functionality of beta1 and beta2 integrins. J Leukoc Biol. 2006;79(3):499–507. doi:10.1189/jlb.0605318.
- Peinado H, Zhang H, Matei IR, Costa-Silva B, Hoshino A, Rodrigues G, Psaila B, Kaplan RN, Bromberg JF, Kang Y, et al. Pre-metastatic niches: organ-specific homes for metastases. Nat Rev Cancer. 2017;17(5):302–317. doi:10.1038/nrc.2017.6.
- Kisucka J, Butterfield CE, Duda DG, Eichenberger SC, Saffaripour S, Ware J, Ruggeri ZM, Jain RK, Folkman J, Wagner DD. Platelets and platelet adhesion support angiogenesis while preventing excessive hemorrhage. Proc Natl Acad Sci USA. 2006;103(4):855–860. doi:10.1073/pnas.0510412103.
- Salgado R, Junius S, Benoy I, Van Dam P, Vermeulen P, Van Marck E, Huget P, Dirix LY. Circulating interleukin-6 predicts survival in patients with metastatic breast cancer. Int J Cancer. 2003;103(5):642–646. doi:10.1002/ijc.10833.
- Lakka Klement G, Yip T-T, Cassiola F, Kikuchi L, Cervi D, Podust V, Italiano JE, Wheatley E, Abou-Slaybi A, Bender E, et al. Platelets actively sequester angiogenesis regulators. Blood. 2009;113(12):2835–2842. doi:10.1182/blood-2008-06-159541.
- Kuznetsov HS, Marsh T, Markens BA, Castaño Z, Greene-Colozzi A, Hay SA, Brown V, Richardson AL, Signoretti S, Battinelli EM, et al. Identification of luminal breast cancers that establish a tumor-supportive macroenvironment defined by proangiogenic platelets and bone marrow-derived cells. Cancer Discov. 2012;2(12):1150–1165. doi:10.1158/2159-8290.CD-12-0216.
- Bodó I, Eikenboom J, Montgomery R, Patzke J, Schneppenheim R, Di Paola J. Platelet-dependent von Willebrand factor activity. Nomenclature and methodology: communication from the SSC of the ISTH. J Thromb Haemost. 2015;13(7):1345–1350. doi:10.1111/jth.12964.
- Pipili-Synetos E, Papadimitriou E, Maragoudakis ME. Evidence that platelets promote tube formation by endothelial cells on matrigel. Br J Pharmacol. 1998;125(6):1252–1257. doi:10.1038/sj.bjp.0702191.
- Garcia BA, Smalley DM, Cho, Shabanowitz J, Ley K, Hunt DF. The platelet microparticle proteome. J Proteome Res. 2005;4(5):1516–1521. doi:10.1021/pr0500760.
- Żmigrodzka M, Guzera M, Miśkiewicz A, Jagielski D, Winnicka A. The biology of extracellular vesicles with focus on platelet microparticles and their role in cancer development and progression. Tumour Biol. 2016;37(11):14391–14401. doi:10.1007/s13277-016-5358-6.
- Langer H, May AE, Daub K, Heinzmann U, Lang P, Schumm M, Vestweber D, Massberg S, Schönberger T, Pfisterer I, et al. Adherent platelets recruit and induce differentiation of murine embryonic endothelial progenitor cells to mature endothelial cells in vitro. Circ Res. 2006;98(2):e2–e10. doi:10.1161/01.RES.0000201285.87524.9e.
- Li R, Ren M, Chen N, Luo M, Deng X, Xia J, Yu G, Liu J, He B, Zhang X, et al. Presence of intratumoral platelets is associated with tumor vessel structure and metastasis. BMC Cancer. 2014;14:167. doi:10.1186/1471-2407-14-167.
- Shirai T, Revenko AS, Tibbitts J, Ngo ATP, Mitrugno A, Healy LD, Johnson J, Tucker EI, Hinds MT, Coussens LM, et al. Hepatic thrombopoietin gene silencing reduces platelet count and breast cancer progression in transgenic MMTV-PyMT mice. Blood Adv. 2019;3(20):3080–3091. doi:10.1182/bloodadvances.2019000250.
- Gay LJ, Felding-Habermann B. Contribution of platelets to tumour metastasis. Nat Rev Cancer. 2011;11(2):123–134. doi:10.1038/nrc3004.
- Luzzi KJ, MacDonald IC, Schmidt EE, Kerkvliet N, Morris VL, Chambers AF, Groom AC. Multistep nature of metastatic inefficiency: dormancy of solitary cells after successful extravasation and limited survival of early micrometastases. Am J Pathol. 1998;153(3):865–873. doi:10.1016/S0002-9440(10)65628-3.
- Nieswandt B, Hafner M, Echtenacher B, Männel DN. Lysis of tumor cells by natural killer cells in mice is impeded by platelets. Cancer Res. 1999;59:1295–1300.
- Morvan MG, Lanier LL. NK cells and cancer: you can teach innate cells new tricks. Nat Rev Cancer. 2016;16(1):7–19. doi:10.1038/nrc.2015.5.
- Zaslavsky A, Baek KH, Lynch RC, Short S, Grillo J, Folkman J, Italiano JE Jr, Ryeom S. Platelet-derived thrombospondin-1 is a critical negative regulator and potential biomarker of angiogenesis. Blood. 2010;115(22):4605–4613. doi:10.1182/blood-2009-09-242065.
- Menter DG, Tucker SC, Kopetz S, Sood AK, Crissman JD, Honn KV. Platelets and cancer: a casual or causal relationship: revisited. Cancer Metastasis Rev. 2014;33(1):231–269. doi:10.1007/s10555-014-9498-0.
- Gasic GJ, Gasic TB, Stewart CC. Antimetastatic effects associated with platelet reduction. Proc Natl Acad Sci USA. 1968;61(1):46–52. doi:10.1073/pnas.61.1.46.
- Palumbo JS, Talmage KE, Massari JV, La Jeunesse CM, Flick MJ, Kombrinck KW, Jirousková M, Degen JL. Platelets and fibrin(ogen) increase metastatic potential by impeding natural killer cell-mediated elimination of tumor cells. Blood. 2005;105(1):178–185. doi:10.1182/blood-2004-06-2272.
- Placke T, Örgel M, Schaller M, Jung G, Rammensee HG, Kopp HG, Salih HR. Platelet-derived MHC class I confers a pseudonormal phenotype to cancer cells that subverts the antitumor reactivity of natural killer immune cells. Cancer Res. 2012;72(2):440–448. doi:10.1158/0008-5472.CAN-11-1872.
- Maurer S, Kropp KN, Klein G, Steinle A, Haen SP, Walz JS, Hinterleitner C, Märklin M, Kopp HG, Salih HR. Platelet-mediated shedding of NKG2D ligands impairs NK cell immune-surveillance of tumor cells. Oncoimmunology. 2018;7(2):e1364827. doi:10.1080/2162402X.2017.1364827.
- Yu LX, Yan L, Yang W, Wu FQ, Ling Y, Chen SZ, Tang L, Tan YX, Cao D, Wu MC, et al. Platelets promote tumour metastasis via interaction between TLR4 and tumour cell-released high-mobility group box1 protein. Nat Commun. 2014;5(1):5256. doi:10.1038/ncomms6256.
- Patmore S, Dhami SPS, O’Sullivan JM. Von Willebrand factor and cancer; metastasis and coagulopathies. J Thromb Haemostasis. 2020;18(10):2444–2456. doi:10.1111/jth.14976.
- Geddings JE, Hisada Y, Boulaftali Y, Getz TM, Whelihan M, Fuentes R, Dee R, Cooley BC, Key NS, Wolberg AS, et al. Tissue factor-positive tumor microvesicles activate platelets and enhance thrombosis in mice. J Thromb Haemost. 2016;14(1):153–166. doi:10.1111/jth.13181.
- Lazarova M, Steinle A. Impairment of NKG2D-Mediated tumor Immunity by TGF-β. Front Immunol. 2019;10:2689. doi:10.3389/fimmu.2019.02689.
- Lee YS, Choi H, Cho H-R, Son W-C, Park Y-S, Kang C-D, Bae J. Downregulation of NKG2DLs by TGF-β in human lung cancer cells. BMC Immunol. 2021;22(1):44. doi:10.1186/s12865-021-00434-8.
- Labelle M, Begum S, Hynes RO. Direct signaling between platelets and cancer cells induces an epithelial-mesenchymal-like transition and promotes metastasis. Cancer Cell. 2011;20(5):576–590. doi:10.1016/j.ccr.2011.09.009.
- Buchheit CL, Weigel KJ, Schafer ZT. Cancer cell survival during detachment from the ECM: multiple barriers to tumour progression. Nat Rev Cancer. 2014;14(9):632–641. doi:10.1038/nrc3789.
- Haemmerle M, Taylor ML, Gutschner T, Pradeep S, Cho MS, Sheng J, Lyons YM, Nagaraja AS, Dood RL, Wen Y, et al. Platelets reduce anoikis and promote metastasis by activating YAP1 signaling. Nat Commun. 2017;8(1):310. doi:10.1038/s41467-017-00411-z.
- Labelle M, Begum S, Hynes RO. Platelets guide the formation of early metastatic niches. Proc Natl Acad Sci USA. 2014;111(30):E3053–E3061. doi:10.1073/pnas.1411082111.
- Ishikawa S, Miyashita T, Inokuchi M, Hayashi H, Oyama K, Tajima H, Takamura H, Ninomiya I, Ahmed AK, Harman JW, et al. Platelets surrounding primary tumor cells are related to chemoresistance. Oncol Rep. 2016;36(2):787–794. doi:10.3892/or.2016.4898.
- Moore RA, Adel N, Riedel E, Bhutani M, Feldman DR, Tabbara NE, Soff G, Parameswaran R, Hassoun H. High incidence of thromboembolic events in patients treated with cisplatin-based chemotherapy: a large retrospective analysis. J Clin Oncol. 2011;29(25):3466–3473. doi:10.1200/JCO.2011.35.5669.
- Radziwon-Balicka A, Medina C, O’Driscoll L, Treumann A, Bazou D, Inkielewicz-Stepniak I, Radomski A, Jow H, Radomski MW. Platelets increase survival of adenocarcinoma cells challenged with anticancer drugs: mechanisms and implications for chemoresistance. Br J Pharmacol. 2012;167(4):787–804. doi:10.1111/j.1476-5381.2012.01991.x.
- Volz J, Mammadova-Bach E, Gil-Pulido J, Nandigama R, Remer K, Sorokin L, Zernecke A, Abrams SI, Ergün S, Henke E, et al. Inhibition of platelet GPVI induces intratumor hemorrhage and increases efficacy of chemotherapy in mice. Blood. 2019;133(25):2696–2706. doi:10.1182/blood.2018877043.
- Demers M, Ho-Tin-Noé B, Schatzberg D, Yang JJ, Wagner DD. Increased efficacy of breast cancer chemotherapy in thrombocytopenic mice. Cancer Res. 2011;71(5):1540–1549. doi:10.1158/0008-5472.CAN-10-2038.
- Haemmerle M, Bottsford-Miller J, Pradeep S, Taylor ML, Choi HJ, Hansen JM, Dalton HJ, Stone RL, Cho MS, Nick AM, et al. FAK regulates platelet extravasation and tumor growth after antiangiogenic therapy withdrawal. J Clin Invest. 2016;126(5):1885–1896. doi:10.1172/JCI85086.
- Elaskalani O, Berndt MC, Falasca M, Metharom P. Targeting platelets for the treatment of cancer. Cancers Basel. 2017;9(7):94. doi:10.3390/cancers9070094.
- Huang Y, Lichtenberger LM, Taylor M, Bottsford-Miller JN, Haemmerle M, Wagner MJ, Lyons Y, Pradeep S, Hu W, Previs RA, et al. Antitumor and antiangiogenic effects of aspirin-PC in ovarian cancer. Mol Cancer Ther. 2016;15(12):2894–2904. doi:10.1158/1535-7163.MCT-16-0074.
- Mitamura T, Gourley C, Sood AK. Prediction of anti-angiogenesis escape. Gynecol Oncol. 2016;141(1):80–85. doi:10.1016/j.ygyno.2015.12.033.
- Xu Z, Li E, Guo Z, Yu R, Hao H, Xu Y, Sun Z, Li X, Lyu J, Wang Q. Design and construction of a multi-organ microfluidic chip mimicking the in vivo microenvironment of lung cancer metastasis. ACS Appl Mater Interfaces. 2016;8(39):25840–25847. doi:10.1021/acsami.6b08746.
- Nagaraju S, Truong D, Mouneimne G, Nikkhah M. Microfluidic tumor-vascular model to study breast cancer cell invasion and intravasation. Adv Healthcare Mater. 2018;7(9):e1701257. doi:10.1002/adhm.201701257.
- Chen MB, Whisler JA, Fröse J, Yu C, Shin Y, Kamm RD. On-chip human microvasculature assay for visualization and quantification of tumor cell extravasation dynamics. Nat Protoc. 2017;12(5):865–880. doi:10.1038/nprot.2017.018.
- Jeon JS, Bersini S, Gilardi M, Dubini G, Charest JL, Moretti M, Kamm RD. Human 3D vascularized organotypic microfluidic assays to study breast cancer cell extravasation. Proc Natl Acad Sci USA. 2015;112(1):214–219. doi:10.1073/pnas.1417115112.
- Kim S, Lee H, Chung M, Jeon NL. Engineering of functional, perfusable 3D microvascular networks on a chip. Lab Chip. 2013;13(8):1489–1500. doi:10.1039/c3lc41320a.
- Jain A, Barrile R, van der Meer AD, Mammoto A, Mammoto T, De Ceunynck K, Aisiku O, Otieno MA, Louden CS, Hamilton GA, et al. Primary human lung alveolus-on-a-chip model of intravascular thrombosis for assessment of therapeutics. Clin Pharmacol Ther. 2018;103(2):332–340. doi:10.1002/cpt.742.
- Jain A, Graveline A, Waterhouse A, Vernet A, Flaumenhaft R, Ingber DE. A shear gradient-activated microfluidic device for automated monitoring of whole blood haemostasis and platelet function. Nat Commun. 2016;7(1):10176. doi:10.1038/ncomms10176.
- Jain A, van der Meer AD, Papa AL, Barrile R, Lai A, Schlechter BL, Otieno MA, Louden CS, Hamilton GA, Michelson AD, et al. Assessment of whole blood thrombosis in a microfluidic device lined by fixed human endothelium. Biomed Microdevices. 2016;18(4):73. doi:10.1007/s10544-016-0095-6.
- Saha B, Mathur T, Handley KF, Hu W, Afshar-Kharghan V, Sood AK, Jain A. OvCa-chip microsystem recreates vascular endothelium–mediated platelet extravasation in ovarian cancer. Blood Adv. 2020;4(14):3329–3342. doi:10.1182/bloodadvances.2020001632.
- Saha B, Mathur T, Tronolone JJ, Chokshi M, Lokhande GK, Selahi A, Gaharwar AK, Afshar-Kharghan V, Sood AK, Bao G, et al. Human tumor microenvironment chip evaluates the consequences of platelet extravasation and combinatorial antitumor-antiplatelet therapy in ovarian cancer. Sci Adv. 2021;7(30):eabg5283. doi:10.1126/sciadv.abg5283.
- Crippa M, Bersini S, Gilardi M, Arrigoni C, Gamba S, Falanga A, Candrian C, Dubini G, Vanoni M, Moretti M. Correction: a microphysiological early metastatic niche on a chip reveals how heterotypic cell interactions and inhibition of integrin subunit β3 impact breast cancer cell extravasation. Lab Chip. 2021;21(6):1185–1185. doi:10.1039/D1LC90024B.
- Papa A-L, Jiang A, Korin N, Chen MB, Langan ET, Waterhouse A, Nash E, Caroff J, Graveline A, Vernet A, et al. Platelet decoys inhibit thrombosis and prevent metastatic tumor formation in preclinical models. Sci Transl Med. 2019;11(479):eaau5898. doi:10.1126/scitranslmed.aau5898.
- Khorana AA, Francis CW, Culakova E, Kuderer NM, Lyman GH. Thromboembolism is a leading cause of death in cancer patients receiving outpatient chemotherapy. J Thromb Haemost. 2007;5(3):632–634. doi:10.1111/j.1538-7836.2007.02374.x.
- Estrada R, Giridharan GA, Nguyen MD, Prabhu SD, Sethu P. Microfluidic endothelial cell culture model to replicate disturbed flow conditions seen in atherosclerosis susceptible regions. Biomicrofluidics. 2011;5(3):32006–32011. doi:10.1063/1.3608137.
- Mannino RG, Myers DR, Ahn B, Wang Y, Margo R, Gole H, Lin AS, Guldberg RE, Giddens DP, Timmins LH, et al. Do-it-yourself in vitro vasculature that recapitulates in vivo geometries for investigating endothelial-blood cell interactions. Sci Rep. 2015;5(1):12401. doi:10.1038/srep12401.
- Zhang YS, Aleman J, Shin SR, Kilic T, Kim D, Mousavi Shaegh SA, Massa S, Riahi R, Chae S, Hu N, et al. Multisensor-integrated organs-on-chips platform for automated and continual in situ monitoring of organoid behaviors. Proc Natl Acad Sci USA. 2017;114(12):E2293–E2302. doi:10.1073/pnas.1612906114.
- McMinn PH, Hind LE, Huttenlocher A, Beebe DJ. Neutrophil trafficking on-a-chip: an in vitro , organotypic model for investigating neutrophil priming, extravasation, and migration with spatiotemporal control. Lab Chip. 2019;19(21):3697–3705. doi:10.1039/C9LC00562E.
- Serebruany VL, Cherepanov V, Cabrera-Fuentes HA, Kim MH. Solid cancers after antiplatelet therapy: Confirmations, controversies, and challenges. Thromb Haemost. 2015;114(6):1104–1112. doi:10.1160/TH15-01-0077.
- Gard AL, Luu RJ, Miller CR, Maloney R, Cain BP, Marr EE, Burns DM, Gaibler R, Mulhern TJ, Wong CA, et al. High-throughput human primary cell-based airway model for evaluating influenza, coronavirus, or other respiratory viruses in vitro. Sci Rep. 2021;11(1):14961. doi:10.1038/s41598-021-94095-7.
- van Meer BJ, de Vries H, Firth KSA, van Weerd J, Tertoolen LGJ, Karperien HBJ, Jonkheijm P, Denning C, IJzerman AP, Mummery CL. Small molecule absorption by PDMS in the context of drug response bioassays. Biochem Biophys Res Commun. 2017;482(2):323–328. doi:10.1016/j.bbrc.2016.11.062.
- Domansky K, Sliz JD, Wen N, Hinojosa C, Thompson G, Fraser JP, Hamkins-Indik T, Hamilton GA, Levner D, Ingber DE. SEBS elastomers for fabrication of microfluidic devices with reduced drug absorption by injection molding and extrusion. Microfluid Nanofluidics. 2017;21(6):107. doi:10.1007/s10404-017-1941-4.
- Schneider S, Brás EJS, Schneider O, Schlünder K, Loskill P. Facile patterning of thermoplastic elastomers and robust bonding to glass and thermoplastics for microfluidic cell culture and organ-on-chip. Micromachines (Basel). 2021;12(5):575. doi:10.3390/mi12050575.
- Baran SW, Brown PC, Baudy AR, Fitzpatrick SC, Frantz C, Fullerton A, Gan J, Hardwick RN, Hillgren KM, Kopec AK, et al. Perspectives on the evaluation and adoption of complex in vitro models in drug development: workshop with the FDA and the pharmaceutical industry (IQ MPS affiliate). Altex. 2022;39(2). doi:10.14573/altex.2112203.
- Bhattacharjee N, Urrios A, Kang S, Folch A. The upcoming 3D-printing revolution in microfluidics. Lab Chip. 2016;16(10):1720–1742. doi:10.1039/C6LC00163G.