Abstract
Alternate splicing is among the regulatory mechanisms imparting functional diversity in proteins. Studying protein isoforms generated through alternative splicing is therefore critical for understanding protein functions in many biological systems. Spleen tyrosine kinase (Syk) plays an essential role in ITAM/hemITAM signaling in many cell types, including platelets. However, the spectrum of Syk isoforms expressed in platelets has not been characterized. Syk has been shown to have a full-length long isoform SykL and a shorter SykS lacking 23 amino acid residues within its interdomain B. Furthermore, putative isoforms lacking another 23 amino acid-long sequence or a combination of the two deletions have been postulated to exist. In this report, we demonstrate that mouse platelets express full-length SykL and the previously described shorter isoform SykS, but lack other shorter isoforms, whereas human platelets express predominantly SykL. These results both indicate a possible role of alternative Syk splicing in the regulation of receptor signaling in mouse platelets and a difference between signaling regulation in mouse and human platelets.
Plain Language Summary
Platelets express two sizes of the Syk molecule with possible alternate functions in the cell. We need to understand how these two differ in their structure so that further studies can be developed by selectively deleting one of them to evaluate their function in platelets. This study shows that platelet Syk molecules differ in their structure with and without a linker region in the molecule.
Introduction
Syk is a non-receptor protein-tyrosine kinase that contains two tandem SH2 domains and a C-terminal tyrosine kinase domain linked by two linker regions (interdomains A and B) (). Syk is highly expressed by hematopoietic cells playing a critical role in signaling through various immunoreceptors, but also by nonhematopoietic cells, including hepatocytes, neurons, and vascular endothelial cells.Citation1–4 In particular, Syk is essential for platelet activation, mediating signals triggered by receptors bearing an immunoreceptor tyrosine-based activation motif (ITAM), such as the glycoprotein VI (GPVI)/Fc receptor-γ chain (FcR-γ chain) complex and FcγRIIA, as well as receptors bearing HemITAMs (hemi ITAM is a signaling structure representing one half of an ITAM), such as the C-type lectin-like receptor-2 (CLEC-2).Citation5–8
Figure 1. Syk gene structure and protein isoforms. The Syk gene consisting of 13 coding exons (2–14) and two 5’ untranslated exons 1a and 1b is presented schematically. The full-length protein SykL (629 amino acid-long in mice) and two relevant alternatively spliced isoforms – the previously characterized short isoform SykS (606 amino acid-long) lacking the sequence encoding by exon 7 and the hypothetical isoform corresponding to the mouse transcript designated Syk-202 (583 amino acid-long) lacking sequences encoded by exons 7 and 14 – are shown. The deletion/truncation locations, their borders and the exons corresponding to them as well as the major protein domains of Syk are indicated. The protein-length scale is shown at the bottom of the figure.
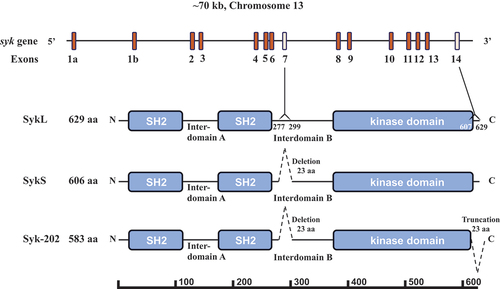
Several studies have identified differential functions of Syk isoforms in cancer progression and metastasis,Citation9–11 including such fundamental cellular phenomena like mitosis, cell cycle, motility, apoptosis, and survival.Citation12–14 In general, Syk activity is induced and regulated by a dynamic network of distinct mechanisms, including phosphorylation of specific tyrosine and serine residues on Syk itself and Syk-interacting structures, including ITAMs and hemITAMs, allosteric activation upon binding to phosphorylated ITAMs and hemITAMs, changes in sub-cellular localization from cytoplasm to membrane, dephosphorylation by protein tyrosine phosphatases, e.g.TULA-2, and proteasomal degradation induced by binding of Syk to the E3 ubiquitin-protein ligase Cbl.Citation1–4,Citation15–17
Besides the full-length isoform of Syk, a shorter splice variant form of termed that lacks a 23 amino acid residues-long stretch in the interdomain B region as a result of exon 7 of the syk gene being spliced out is also expressed in humans and rodents.Citation18–21 Several nomenclature systems exist to denote these isoforms; they will be termed here SykL (long) and SykS (short) for consistency’s sake. In human cells, these two isoforms have been shown to have differential expression, sub-cellular localization, and functional effects in cancer cellsCitation1–4,Citation21 suggesting the existence of alternative splicing-dependent mechanisms operating in concert with other mechanisms controlling Syk activity. Also, a putative short isoform of Syk lacking two protein sequences each containing 23 amino acid residues, which corresponds to a syk transcript lacking exons 7 and 14 of the long transcript, is proposed to exist in mice (ENSMUST00000118756.8 Syk-202; https://useast.ensembl.org/Mus_musculus/) (see ).
Since Syk is a key signaling protein of platelets, and the functions and regulation of various Syk isoforms may differ substantially, identification of Syk isoforms in platelets is important for understanding the role Syk plays in these cells. However, despite their potential importance, little is known about the expression of Syk isoforms in platelets. In this report, we describe the identification of Syk isoforms expressed in human and mouse platelets, providing evidence that human platelets express only full-length Syk (SykL), while mouse platelets express both SykL and SykS, an isoform lacking a protein sequence inside the interdomain B but retaining the C-terminal sequence.
Results
Molecular weight determination of Syk isoforms in platelets
Syk expressed in human and mouse platelets was first characterized using immunoblotting with a monoclonal antibody directed to the N-terminal region of Syk (aa 5–360 of human Syk). Comparison of anti-Syk reactive bands indicated that a protein species of 72 kDa is predominant in the lysates of human platelets, while two distinct species of 69 and 66 kDa are evident in mouse platelet lysates (). The apparent molecular mass of the major Syk band in human platelets corresponded to the calculated molecular mass of human full-length Syk (SykL), whereas mouse platelet Syk species appeared to correspond to either SykS lacking the sequence encoded by exon 7 (69 kDa) or the putative short-form Syk-202, lacking the sequences encoded by exon 7 and exon 14 of the full-length transcript (66 kDa), in the Ensemble description of mouse Syk (https://useast.ensembl.org/Mus_musculus/Transcript/Summary?db=core;g=ENSMUSG00000021457;r=13:52737473–52797650;t=ENSMUST00000118756). However, apparent molecular masses of proteins determined using electrophoresis may differ substantially from their calculated molecular masses.
Figure 2. Molecular masses of Syk isoforms in various tissues. (A) lysates of human and mouse platelets were resolved using SDS-PAGE and probed with SYK-01, a monoclonal antibody raised against the 5-360aa region of Syk. (B) lysates of various mouse cells and tissues as indicated have been analyzed as described in (A). The molecular masses of Syk isoforms determined using protein standards are indicated in (A) and (B). The data are representative of at least three independent experiments from three different human donors and mice.
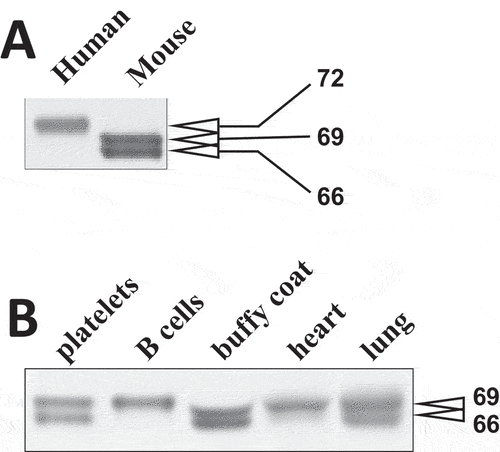
Comparison of Syk isoforms in platelets to Syk isoforms in other cells and tissues
To further examine this issue, we first compared the electrophoretic mobility of mouse platelet Syk to that of Syk from other mouse tissues. It has been shown previously that both SykL and SykS proteins are abundant in mouse lungs, whereas only SykL is abundant in mouse spleen.Citation20 Notably, the transcripts for both forms of Syk were found in all tissues analyzed, but the ratio of SykL mRNA to SykS mRNA varied from more than 10 in spleen and liver to less than 1 in colon.Citation20 Comparison of mouse Syk from platelets to that from B cells, which are the major source of Syk in the spleen, and other cells and tissues demonstrated that the top platelet Syk band co-migrated with the predominant spleen Syk band and the top Syk band from other sources, including lung, which are thought to represent SykL (). This result strongly suggests that a disparity in the apparent molecular mass between human platelet Syk and the mouse platelet Syk top band is due to the difference in amino acid composition (thus, the calculated isoelectric points of human and mouse full-length Syk are 8.4 and 7.9, respectively, demonstrating a considerable difference) and not due to the lack of a protein fragment.
Detecting specific marker sequences in platelet Syk isoforms
To directly determine the presence of amino acids encoded by exon 7 in mouse Syk, we employed immunoblotting with anti-Syk(pSer291) (amino acid numbering is for mouse full-length Syk; corresponds to Syk Ser297 in humans). This residue is a key feature of the exon 7-encoded protein sequence present in full-length Syk, which is missing in SykS, and thus can be used as a marker of this sequence. Immunoblotting of platelet lysates following activation through the GPVI receptor/Fc-γ chain complex shows that only the top Syk band, but not the bottom band of mouse platelets, is reactive with an anti-Syk(pSer291) antibody, indicating the presence of the amino acid sequence encoded by exon 7 in the reactive species (). This also rules out the exon 7 coded protein segment in the lower molecular weight Syk in mice.
Figure 3. Exon 7-encoded amino acid sequence is present in the top mouse Syk isoform. (A) the Syk sequence encoded by exon 7, the numbers of amino acid residues corresponding to its borders, and SER291, a stimulation-induced phosphorylation site located within this sequence, are presented on a diagram. (B) WT mouse platelets were stimulated with CRP at different concentrations or left non-stimulated as indicated. Platelet lysates were resolved using SDS-PAGE and analyzed as described in the legend of except that pSER291 was also probed with a specific anti-pSER291 antibody. The positions of protein standards are indicated schematically with bars and presented on the immunoblots (intense red bands). Their molecular masses in kDa are indicated. The results are presented as individual panels for anti-pSER291 and anti-Syk (B) or a combination panel (C). The data are representative of at least three independent experiments.
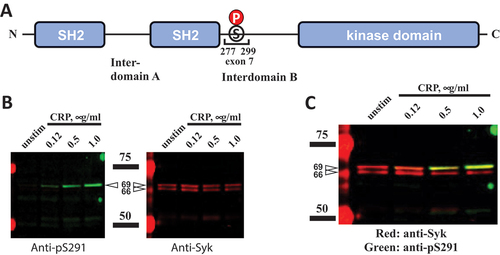
As indicated above, a 72-kDa size of the human platelet Syk band (see ) strongly suggested that this band represents the full-length SykL form. In contrast, despite the results shown in it remained possible that the top, 69-kDa Syk band of mouse platelets is not SykL, but the short form of Syk that contains the sequence encoded by exon 7 but lacks the C-terminal sequence encoded by exon 14 of the full-length isoform. The lower mouse Syk band in platelets could be either the exon 7-missing SykS (if the top band represents SykL) or the isoform lacking sequences encoded by both exons 7 and 14 corresponding to the predicted Syk-202 transcript (ENSMUST00000118756.8) (if the top band is having the exon 7-encoded sequence but lacking the exon 14-encoded one) (). To determine the presence of the C-terminal sequence of SykL in the mouse platelet Syk isoforms, we used immunoblotting with an antibody raised against the C-terminal fragment of full-length Syk. The human Syk and both mouse Syk isoforms reacted with this anti-Syk antibody indicating the presence of the C-terminal sequence in all these Syk species (). To validate this result, we assured the specificity of the observed antigen–antibody interactions by its blocking with the peptide corresponding to the Syk sequence between residues 607 and 629 (VENRPGFTAVELRLRNYYYDVVN) which represents most of the sequence encoded by exon 14. The Syk 607–629 peptide added to anti-Syk antibody in excess completely blocked recognition of all human and mouse platelet Syk species in immunoblotting, thus indicating that this interaction is specific ().
Figure 4. Exon 14-encoded C-terminal amino acid sequence is present in all Syk isoforms examined. (A) the Syk sequences encoded by exons 7 and 14 and their borders are indicated on a diagram. The synthetic peptide corresponding to the 613-629aa Syk sequence, which was used for blocking, is shown. (B) human and mouse platelet lysates were analyzed as described in the legend of fig. 2 with two modifications: SDS-PAGE-separated proteins were probed with an antibody raised to the C-terminal fragment of Syk (CST-2712), which was preincubated with the Syk 607–629 peptide VENRPGFTAVELRLRNYYYDVVN (blocking) or a scrambled peptide (control) for 30 min at room temperature. Blocking by the 607–629 peptide was examined at two different concentrations as indicated. The positions of protein standards and their molecular masses in kDa are indicated. The data are representative of at least three independent experiments.
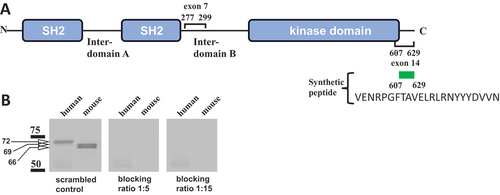
Discussion
Several conclusions can be drawn from the results described above. Two Syk isoforms are present in mouse platelets, and neither isoform lacks the C-terminal region encoded by exon 14 of the syk gene. The short Syk isoform in mouse platelets lacks the region encoded by exon 7, while the long isoform retains this region. Hence, the long mouse platelet Syk isoform corresponds to the full-length Syk (SykL), while the short one corresponds to the isoform termed SykS. The presence of two comparably abundant Syk isoforms differentiates mouse platelets from human platelets and mouse B cells, in which Syk plays a key role in signal transductionCitation1–4 and which are examined in this study. Full-length Syk represents a predominant isoform in both human platelets and mouse B cells, while the short isoform of Syk is not detectable in these cells. The expression of Syk isoforms has not been studied previously in platelets, especially in the context of interspecies differences.
Protein composition of human and mouse platelets has been studied by Burkhart et al.Citation22 and Zeiler et al.Citation23 respectively, using proteomics. These approaches could identify individual protein isoforms if the isoforms are substantially different in their sequences, and thus these groups identified platelet PLC-β3 and PLC-β4 isoforms, which exhibit identity of ~40%, but did not differentiate SykS and SykL, which are identical with the exception that ~4% of the SykL sequence is missing from SykS. The only conclusion that can potentially be drawn from such experiments is the presence of the SykL isoform if the peptides missing from SykS are detected. Thus, Burkhart et al. show the presence of phosphosites specific for SykL (S295, Y296, and S297).Citation22 However, these data say nothing definitive about the presence of SykS, since the presence of SykL-specific peptides does not rule out the simultaneous presence of SykS. Furthermore, the sequence coverage in such experiments is typically incomplete (thus, in Zeiler et al., it varies from ~20% to 100% for different proteins and ~64% for SykCitation23), so even the absence of the SykL-specific peptides does not guarantee the absence of SykL.
The difference between the functions of SykL and SykS has been studied extensively in cancer. It appears that SykL, but not SykS, plays a tumor-suppressing role in several types of cancer, affecting proliferation, survival, invasion, and metastasis.Citation10–13,Citation21 The differential role of SykL and SykS might be linked to the impairment of nuclear localization of Syk by the deletion of the exon 7-encoded sequence; SykL, but not SykS, is capable of translocating to the nucleus.Citation12,Citation21 It would be interesting to know the intraplatelet localization of these two isoforms. At this point, we are limited by the antibodies that recognize each of these isoforms separately. The only antibody that we have is for phosphorylated Syk S291. This will determine the localization of SykL upon platelet activation but not in quiescent platelet. Thus, we will not be able to determine the intraplatelet localization of these isoforms in unstimulated platelets. However, such a difference between the two Syk isoforms is unlikely to be relevant in platelets, which are anucleated, although such differential role for the two Syk isoforms in megakaryocytes needs to be investigated.
A more potentially significant implication is related to the possibility of differential signaling functions of SykL and SykS. This issue has been examined, but the results remain inconclusive. In one study, it was shown that SykL is more phosphorylated than SykS and is more efficient in mediating signaling and activation of basophilic cells through FcɛR1, a receptor sharing the critical ITAM-bearing γ-chain with the GPVI collagen receptor of platelets.Citation24 This study also showed that SykL associates better with phosphopeptides corresponding to various ITAMs.Citation24 In contrast, it has also been shown that both SykL and SykS similarly mediate signaling and responses in basophilic cells.Citation19 Furthermore, the kinase activity of short and long Syk has been shown to be similarCitation19,Citation25 and to be similarly up-regulated by phosphopeptides corresponding to the γ-chain ITAM.Citation19 Finally, it has been shown that SykL and SykS do not differ in their ability to support phagocytosis mediated by Fcγ receptors.Citation26 Taken together, these results suggest that SykL and SykS are overall similar in their signaling functions, but quantitative differences between their effects are quite possible.
The major significance of the difference between SykL and SykS in platelets may be related to the presence of several potential regulatory tyrosine (Y290) and serine (S289, S291) residues within the insert. It has been shown that these sites can be phosphorylated in platelets and B cells in response to receptor stimulation.Citation27–29 Phosphorylation of Y290 does not seem to affect Syk-mediated signaling significantly.Citation24,Citation30 However, phosphorylation of Ser291, which appears to be mediated by PKC,Citation30,Citation31 is likely to be regulatory, although the views on the effect of this phosphorylation differ. The experiments with mouse Syk in chicken DT40 B cells suggested that pSer291 facilitates B-cell receptor (BCR)-induced activation of Raf, Erk, and NF-AT,Citation30 while the experiments with human Syk in the same cells suggested that pSer297, which is the human homologue of mouse pSer291, suppresses phosphorylation of BCR-proximal substrates SLP-65 and PLC-γ2 as well as the BCR-induced Ca2+ flux.Citation29 Thus, although the exact nature of the regulatory effect of pSer291 (human pS297) is unclear, the studies consistently indicate that this pSer-site exerts a substantial regulatory effect on Syk-mediated receptor signaling. Therefore, the SykL isoform can potentially be regulated by pSer291, whereas SykS cannot be a target for this regulation due to the absence of the exon 7-encoded insert, which contain Ser291.
Biological significance of the observed difference in the Syk isoform pattern between mouse and human platelets remains to be understood. However, it should be noted that several differences relevant for receptor signal transduction exist between mouse and human platelets. Some of these differences are qualitative, e.g. the ITAM-bearing receptor FcγRIIA is present in humans but absent in mouse platelets.Citation32 Some very substantial quantitative differences also exist, e.g. mouse platelets demonstrate an approximately three- to fourfold molar excess of Syk over the GPVI receptor, while human platelets demonstrate an inverse situation where GPVI is expressed at a level 2- to 5-times higher than Syk.Citation33 It is possible that these variations justify the differential expression of Syk isoforms in human and mouse platelets. The differences between mouse platelets and B cells may be due to the global differences between their ITAM-bearing receptors and even between their non-ITAM-being receptors, such as Toll-like and Dectin-1 receptors, which also require Syk for signal transduction.Citation34,Citation35
Platelets are anucleate cells derived from megakaryocytes, from which platelets inherit a pool of proteins and mRNAs. Although transcription is likely impossible in anucleate cells, there is ample evidence of rapid and sustained signal-dependent translation in platelets.Citation36 Recently, it has also been shown that resident immature pre-mRNAs in resting platelets undergo splicing and subsequent protein synthesis leading to functionally relevant modification of the platelet proteome in response to stimulation through either collagen or thrombin receptors.Citation37 These findings suggest that RNA splicing and protein synthesis in activated platelets may fine-tune the functions of these cells. Hence, the differences in the Syk isoform pattern between mouse and human platelets reported here may be a manifestation of differential regulation of Syk based on pre-mRNA splicing in these cells.
Experimental procedures
Antibodies and reagents
All reagents were purchased from Thermo Fischer Scientific unless otherwise stated. Anti-pSyk Ser297 (mouse Ser291) (cat#14140) and anti-Syk (cat#2712; directed against the C-terminal of Syk) were purchased from Cell Signaling Technology (Beverly, MA). Anti-Syk was also purchased from Santa Cruz technology (SYK-01; cat# sc-51 703; Santa Cruz, CA). OneBlock Western-FL blocking buffer was purchased from Genesee Scientific (El Cajon, CA). Secondary antibodies IRDye 800CW goat anti-rabbit and IRDye 680LT goat anti-mouse were purchased from Li-Cor (Lincoln, NE). CRP-XL was purchased from Cambcol (the United Kingdom). The synthetic peptide VENRPGFTAVELRLRNYYYDVVN was purchased from GenScript Biotech (Piscataway, NJ).
Animal housing and production
Mice were housed in a pathogen-free facility, and all animal procedures were approved by the Temple University Institutional Animal Care and Use Committee (protocol #4864).
Preparation of mouse platelets
Mouse blood was collected and processed as previously described.Citation38 Briefly, blood from anesthetized mice was collected via cardiac puncture into one-tenth volume 3.8% sodium citrate and centrifuged at 100 × g for 10 min at room temperature. The platelet-rich plasma (PRP) was removed, and 400 µl sodium citrate was added to the remaining packed cells prior to a second 100 × g spin. The resulting batches of PRP were combined, and 1 μM PGE1 was added to the PRP before centrifugation at 400×g for 10 min. The resulting platelet pellet was resuspended in Tyrode's buffer (138 mM NaCl, 2.7 mM KCl, 2 mM MgCl 2, 0.42 mM NaH2PO4, 10 mM HEPES, 0.1% glucose, and 0.2 U/mL apyrase, pH 7.4), and platelets were counted using a Hemavet 950FS blood cell counter (Drew Scientific, Dallas, TX) and adjusted to a final concentration of 1.5 × 10 8 cells/ml.
When necessary, the buffy coat was collected after removal of the PRP, and proteins were extracted in RIPA buffer as described below.
Preparation of mouse organ samples
The heart and lung were excised from an anesthetized mouse, cut into small pieces, and homogenized in 200 μl RIPA buffer. (1× RIPA: 100 mM Tris, 500 mM NaCl, 1% SDS, 0.5% sodium deoxycholate, 1% NP40 (pH 7.4) containing protease/phosphatase inhibitors.) Samples were centrifuged at 14 000 × g for 20 min at +4°C. The supernatant was removed and recentrifuged as above. Proteins were precipitated with 0.6 N HClO4 and washed with water prior to the addition of sample loading buffer.
Preparation of human platelets
Blood was drawn from informed healthy volunteers according to a protocol approved by the Institutional Review Board of Temple University (Approval #23688) in accordance with the Declaration of Helsinki into one-sixth volume of acid-citrate-dextrose (85 mM sodium citrate, 111 mM glucose, 71.4 mM citric acid). PRP was isolated by centrifugation at 230 × g for 20 min. Platelets were obtained by centrifugation of the PRP for 10 min at 980 × g and resuspended in Tyrode’s buffer as described above. The platelet counts were adjusted to 2 × 108 platelets/ml.
Western blotting
Western blotting procedures were performed as described previously.Citation38 Briefly, proteins were precipitated with 0.6 N HClO4 and washed with water prior to the addition of sample loading buffer. Samples were then boiled for 5 min prior to resolution by 8% SDS-PAGE and transferred to nitrocellulose membranes with the iBlot2 (Invitrogen). The membranes were then blocked using Prometheus OneBlock Western-FL blocking buffer and incubated overnight with primary antibodies against the indicated protein. The blocking or control peptide was added to the incubation medium containing the antibody to the C-terminal Syk sequence at an indicated excess (by weight). The membranes were then washed with Tris-buffered saline containing 0.1% Tween-20 prior to incubation with appropriate secondary antibodies for 1 h at room temperature. The membranes were washed again and imaged using a LI-COR Odyssey infrared imaging system.
Author contributions
P.K.S. performed experiments, analyzed and interpreted data, and drafted the manuscript; C.D. designed experiments, analyzed and interpreted data; H.R.V. performed experiments; A.Y.T. interpreted the data and edited the manuscript; and S.P.K. designed experiments and provided overall supervision.
Acknowledgments
We would like to thank Ms Monica Wright for animal breeding, genotyping, and blood drawing.
Disclosure statement
No potential conflict of interest was reported by the author(s).
Additional information
Funding
References
- Yanagi S, Inatome R, Takano T, Yamamura H. Syk expression and novel function in a wide variety of tissues. Biochem Biophys Res Commun. 2001;288(3):495–7. doi:10.1006/bbrc.2001.5788.
- Tsygankov AY. Non-receptor protein tyrosine kinases. Front Biosci. 2003;8(6):s595–635. doi:10.2741/1106.
- Geahlen RL. Syk and pTyr’d: signaling through the B cell antigen receptor. Biochim Biophys Acta. 2009;1793(7):1115–27. doi:10.1016/j.bbamcr.2009.03.004.
- Mocsai A, Ruland J, Tybulewicz VL. The SYK tyrosine kinase: a crucial player in diverse biological functions. Nat Rev Immunol. 2010;10(6):387–402. doi:10.1038/nri2765.
- Bhavaraju K, Kim S, Daniel JL, Kunapuli SP. Evaluation of [3-(1-methyl-1H-indol-3-yl-methylene)-2-oxo-2, 3-dihydro-1H-indole-5-sulfonamide] (OXSI-2), as a Syk-selective inhibitor in platelets. Eur J Pharmacol. 2008;580(3):285–90. doi:10.1016/j.ejphar.2007.11.009.
- Poole A, Gibbins JM, Turner M, van Vugt MJ, van de Winkel JG, Saito T, Tybulewicz VL, Watson SP. The Fc receptor γ-chain and the tyrosine kinase Syk are essential for activation of mouse platelets by collagen. EMBO J. 1997;16(9):2333–41. doi:10.1093/emboj/16.9.2333.
- Suzuki-Inoue K, Wilde JI, Andrews RK, Auger JM, Siraganian RP, Sekiya F, Rhee SG, Watson SP. Glycoproteins VI and Ib-IX-V stimulate tyrosine phosphorylation of tyrosine kinase Syk and phospholipase Cgamma2 at distinct sites. Biochem J. 2004;378(3):1023–9. doi:10.1042/bj20031430.
- Watson AA, Christou CM, James JR, Fenton-May AE, Moncayo GE, Mistry AR, Davis SJ, Gilbert RJ, Chakera A, O’Callaghan CA. The platelet receptor CLEC-2 is active as a dimer. Biochemistry. 2009;48(46):10988–96. doi:10.1021/bi901427d.
- Coopman PJ, Mueller SC. The Syk tyrosine kinase: a new negative regulator in tumor growth and progression. Cancer Lett. 2006;241(2):159–73. doi:10.1016/j.canlet.2005.11.004.
- Hong J, Yuan Y, Wang J, Liao Y, Zou R, Zhu C, Li B, Liang Y, Huang P, Wang Z, et al. Expression of variant isoforms of the tyrosine kinase SYK determines the prognosis of hepatocellular carcinoma. Cancer Res. 2014;74(6):1845–56. doi:10.1158/0008-5472.CAN-13-2104.
- Coebergh van den Braak RRJ, Sieuwerts AM, Kandimalla R, Lalmahomed ZS, Bril SI, van Galen A, Smid M, Biermann K, van Krieken J, Kloosterman WP, et al. High mRNA expression of splice variant SYK short correlates with hepatic disease progression in chemonaive lymph node negative colon cancer patients. PLoS One. 2017;12(9):e0185607. doi:10.1371/journal.pone.0185607.
- Prinos P, Garneau D, Lucier JF, Gendron D, Couture S, Boivin M, Brosseau JP, Lapointe E, Thibault P, Durand M, et al. Alternative splicing of SYK regulates mitosis and cell survival. Nat Struct Mol Biol. 2011;18(6):673–9. doi:10.1038/nsmb.2040.
- Ni B, Hu J, Chen D, Li L, Chen D, Wang J, Wang L. Alternative splicing of spleen tyrosine kinase differentially regulates colorectal cancer progression. Oncol Lett. 2016;12(3):1737–44. doi:10.3892/ol.2016.4858.
- Denis V, Cassagnard N, Del Rio M, Cornillot E, Bec N, Larroque C, Jeanson L, Jarlier M, Combes E, Robert B, et al. Targeting the splicing isoforms of spleen tyrosine kinase affects the viability of colorectal cancer cells. PLoS One. 2022;17(9):e0274390. doi:10.1371/journal.pone.0274390.
- Rao N, Ghosh AK, Ota S, Zhou P, Reddi AL, Hakezi K, Druker BK, Wu J, Band H. The non-receptor tyrosine kinase Syk is a target of Cbl-mediated ubiquitylation upon B-cell receptor stimulation. EMBO J. 2001;20:7085–95. doi:10.1093/emboj/20.24.7085.
- Agrawal R, Carpino N, Tsygankov A. TULA proteins regulate activity of the protein tyrosine kinase Syk. J Cell Biochem. 2008;104(3):953–64. doi:10.1002/jcb.21678.
- Chen X, Ren L, Kim S, Carpino N, Daniel JL, Kunapuli SP, Tsygankov AY, Pei D. Determination of the substrate specificity of protein-tyrosine phosphatase TULA-2 and identification of Syk as a TULA-2 substrate. J Biol Chem. 2010;285(41):31268–76. doi:10.1074/jbc.M110.114181.
- Rowley RB, Bolen JB, Fargnoli J. Molecular cloning of rodent p72Syk. Evidence of alternative mRNA splicing. J Biol Chem. 1995;270(21):12659–64. doi:10.1074/jbc.270.21.12659.
- Yamashita T, Kairiyama L, Araki M, Nagasawa S. Evidence for involvement of two isoforms of Syk protein-tyrosine kinase in signal transduction through the high affinity IgE receptor on rat basophilic leukemia cells. J Biochem (Tokyo). 1998;123(6):1199–207. doi:10.1093/oxfordjournals.jbchem.a022061.
- Duta F, Ulanova M, Seidel D, Puttagunta L, Musat-Marcu S, Harrod KS, Schreiber AD, Steinhoff U, Befus AD. Differential expression of spleen tyrosine kinase Syk isoforms in tissues: effects of the microbial flora. Histochem Cell Biol. 2006;126(4):495–505. doi:10.1007/s00418-006-0188-z.
- Wang L, Duke L, Zhang PS, Arlinghaus RB, Symmans WF, Sahin A, Mendez R, Dai JL. Alternative splicing disrupts a nuclear localization signal in spleen tyrosine kinase that is required for invasion suppression in breast cancer. Cancer Res. 2003;63:4724–30.
- Burkhart JM, Vaudel M, Gambaryan S, Radau S, Walter U, Martens L, Geiger J, Sickmann A, Zahedi RP. The first comprehensive and quantitative analysis of human platelet protein composition allows the comparative analysis of structural and functional pathways. Blood. 2012;120(15):e73–82. doi:10.1182/blood-2012-04-416594.
- Zeiler M, Moser M, Mann M. Copy number analysis of the murine platelet proteome spanning the complete abundance range. Mol Cell Proteomics. 2014;13(12):3435–45. doi:10.1074/mcp.M114.038513.
- Latour S, Zhang J, Siraganian RP, Veillette A. A unique insert in the linker domain of Syk is necessary for its function in immunoreceptor signalling. EMBO J. 1998;17:2584–95. doi:10.1093/emboj/17.9.2584.
- Latour S, Chow LM, Veillette A. Differential intrinsic enzymatic activity of Syk and Zap-70 protein-tyrosine kinases. J Biol Chem. 1996;271(37):22782–90. doi:10.1074/jbc.271.37.22782.
- Hunter S, Sato N, Kim MK, Huang ZY, Chu DH, Park JG, Schreiber AD. Structural requirements of Syk kinase for Fcγ receptor– phagocytosis. Exp Hematol. 1999;27(5):875–84. doi:10.1016/S0301-472X(99)00025-9.
- Zahedi RP, Lewandrowski U, Wiesner J, Wortelkamp S, Moebius J, Schutz C, Walter U, Gambaryan S, Sickmann A. Phosphoproteome of resting human platelets. J Proteome Res. 2008;7(2):526–34. doi:10.1021/pr0704130.
- Beck F, Geiger J, Gambaryan S, Solari FA, Dell’aica M, Loroch S, Mattheij NJ, Mindukshev I, Potz O, Jurk K, et al. Temporal quantitative phosphoproteomics of ADP stimulation reveals novel central nodes in platelet activation and inhibition. Blood. 2017;129(2):e1–e12. doi:10.1182/blood-2016-05-714048.
- Bohnenberger H, Oellerich T, Engelke M, Hsiao HH, Urlaub H, Wienands J. Complex phosphorylation dynamics control the composition of the Syk interactome in B cells. Eur J Immunol. 2011;41(6):1550–62. doi:10.1002/eji.201041326.
- Paris LL, Hu J, Galan J, Ong SS, Martin VA, Ma H, Tao WA, Harrison ML, Geahlen RL. Regulation of Syk by phosphorylation on serine in the linker insert. J Biol Chem. 2010;285(51):39844–54. doi:10.1074/jbc.M110.164509.
- Makhoul S, Dorschel S, Gambaryan S, Walter U, Jurk K. Feedback regulation of Syk by protein kinase C in human platelets. Int J Mol Sci. 2019;21(1):21. doi:10.3390/ijms21010176.
- Zhou Y, Abraham S, Andre P, Edelstein LC, Shaw CA, Dangelmaier CA, Tsygankov AY, Kunapuli SP, Bray PF, McKenzie SE. Anti–miR-148a regulates platelet FcγRIIA signaling and decreases thrombosis in vivo in mice. Blood. 2015;126(26):2871–81. doi:10.1182/blood-2015-02-631135.
- Dunster JL, Unsworth AJ, Bye AP, Haining EJ, Sowa MA, Di Y, Sage T, Pallini C, Pike JA, Hardy AT, et al. Interspecies differences in protein expression do not impact the spatiotemporal regulation of glycoprotein VI mediated activation. J Thromb Haemost. 2020;18(2):485–96. doi:10.1111/jth.14673.
- Schweighoffer E, Nys J, Vanes L, Smithers N, Tybulewicz VLJ. TLR4 signals in B lymphocytes are transduced via the B cell antigen receptor and SYK. J Exp Med. 2017;214(5):1269–80. doi:10.1084/jem.20161117.
- Ali MF, Driscoll CB, Walters PR, Limper AH, Carmona. β-Glucan–activated human B Lymphocytes participate in innate immune responses by releasing proinflammatory cytokines and stimulating neutrophil chemotaxis. J Immunol. 2015;195(11):5318–26. doi:10.4049/jimmunol.1500559.
- Zimmerman GA, Weyrich AS. Signal-dependent protein synthesis by activated platelets: new pathways to altered phenotype and function. Arterioscler Thromb Vasc Biol. 2008;28(3):s17–24. doi:10.1161/ATVBAHA.107.160218.
- Nassa G, Giurato G, Cimmino G, Rizzo F, Ravo M, Salvati A, Nyman TA, Zhu Y, Vesterlund M, Lehtio J, et al. Splicing of platelet resident pre-mRnas upon activation by physiological stimuli results in functionally relevant proteome modifications. Sci Rep. 2018;8(1):498. doi:10.1038/s41598-017-18985-5.
- Reppschlager K, Gosselin J, Dangelmaier CA, Thomas DH, Carpino N, McKenzie SE, Kunapuli SP, Tsygankov AY. TULA-2 protein phosphatase suppresses activation of Syk through the GPVI platelet receptor for collagen by dephosphorylating Tyr(P)346, a regulatory site of Syk. J Biol Chem. 2016;291(43):22427–41. doi:10.1074/jbc.M116.743732.