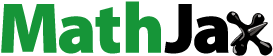
Abstract
Cryopreservation affects platelets’ function, questioning their use for cancer patients. We aimed to investigate the biochemical events that occur over time after thawing to optimize transfusion timing and evaluate the effect of platelet supernatants on tumor cell behavior in vitro. We compared fresh (Fresh-PLT) with Cryopreserved platelets (Cryo-PLT) at 1 h, 3 h and 6 h after thawing. MCF-7 and HL-60 cells were cultured with Fresh- or 1 h Cryo-PLT supernatants to investigate cell proliferation, migration, and PLT-cell adhesion. We noticed a significant impairment of hemostatic activity accompanied by a post-thaw decrease of CD42b+ , which identifies the CD62P−-population. FTIR spectroscopy revealed a decrease in the total protein content together with changes in their conformational structure, which identified two sub-groups: 1) Fresh and 1 h Cryo-PLT; 2) 3 h and 6 h cryo-PLT. Extracellular vesicle shedding and phosphatidylserine externalization (PS) increased after thawing. Cryo-PLT supernatants inhibited cell proliferation, impaired MCF-7 cell migration, and reduced ability to adhere to tumor cells. Within the first 3 hours after thawing, irreversible alterations of biomolecular structure occur in Cryo-PLT. Nevertheless, Cryo-PLT should be considered safe for the transfusion of cancer patients because of their insufficient capability to promote cancer cell proliferation, adhesion, or migration.
Plain Language Summary
What is the context?
Transfusion of Fresh platelets (Fresh-PLT) with prophylaxis purposes is common in onco-hematological patients.
Cryopreservation is an alternative storage method that allows to extend platelet component shelf life and build supplies usable in case of emergency.
It is well established that cryopreservation affects platelet function questioning their use in onco-hematological patients.
It is still unknown how platelet impairment, induced by cryopreservation, occurs over time after thawing, nor how the by-products of PLT deterioration may impact on cancer cell behavior.
What is new?
In this study, we deeply characterized the functional and morphological changes induced by cryopreservation on platelets by comparing Fresh-PLT with Cryo-PLT at 1 h, 3 h and 6 h after thawing. Afterwards, we evaluated the effect of PLT supernatants on cancer cell behavior in vitro.
The data presented show that within 3 hours after thawing Cryo-PLT undergo to irreversible macromolecular changes accompanied by increase of peroxidation processes and protein misfolding.
After thawing the clot formation is reduced but still supported at all-time points measured, combined with unchanged phosphatidylserine expression and extracellular vesicles release over time.
Cryo-PLT supernatants do not sustain proliferation and migration of cancer cells.
WHAT is the impact?
Cryo-PLT may be considered a precious back-up product to be used during periods of Fresh-PLT shortage to prevent bleeding in non-hemorrhagic patients.
It is desirable to make it logistically feasible to transfuse cryopreserved platelets within 1 hour of thawing to maintain the platelets in their best performing condition.
Introduction
Platelets are multifunctional blood components that play a pivotal role in many pathophysiological processes, especially in hemostasis and inflammation.Citation1 The number of circulating platelets may decrease consequently to severe bleeding, reduced production from the bone marrow, spleen seizure or increased consumption. All these conditions may eventually result in thrombocytopenia, which may require transfusion with platelet concentrates (PLT) in order to restore platelet count, and prevent possible bleeding.Citation2
The large majority of PLT recipients are thrombocytopenic onco-hematological patients, for whom platelets production is hampered by the disease itself or by the therapeutic treatment.Citation3,Citation4
PLT for transfusion purposes are collected from healthy volunteers by whole blood donation or by apheresis. Once collected, platelets are stored in either plasma or platelet additive solution (PAS) and maintained at room temperature (RT, 22–24°C) in gas-permeable storage bags. To reduce the risk of septic transfusion reactions, PLT storage is recommended for up to 5–7 days after collection under constant gentle agitation to prevent aggregates.Citation5 The exact shelf-life depends on the regulation by country.
RT storage makes PLT supply logistically complex and dependent on constant donor availability, which may fail during emergency periods (i.e. SARS-Cov-2 pandemic).Citation6 PLT cryopreservation represents an alternative to RT maintenance: following different freezing protocols, PLT can be stored at −80°C up to 1 year, or, as recommend by the EU Guidelines for storage longer than 1 year at ≤−150°C, in vapor-phase liquid nitrogen.Citation7 The maximal length of storage for Cryo-PLT at −80°C has not been universally established; recent studies have suggested an extended shelf-life up to 5 or 12 years.Citation8 However, the only limitations for the use of Cryo-PLT is the platelet recovery count after thawing, which must be over 50% on the pre-freezing count, and the shelf-life that is limited to 6 hours after thawing.Citation9–11
Platelets may undergo a series of biological changes during PLT concentrates preparation.Citation12–14 Metabolic changes (i.e. loss of discoidal morphology, pH variations, reduction of PLT functionality, fragmentation and altered surface antigen expression) that continue to accumulate during storage are collectively referred as “platelet storage lesions” (PSL), which are dependent from the resuspension media.Citation15–17 PSL development is associated to reduced platelets in vivo recovery, survival, and hemostatic activity after transfusion.Citation18,Citation19 It is well known that reduced platelet function is significantly associated with bleeding riskCitation20 and reduced platelet recovery in cancer patients.Citation21 The accumulation of lesions has been largely documented both for RT and Cryo-PLT, with the latter having lower functionality but also decreasing the chance of developing bacterial contamination.Citation22–24 The use of Cryo-PLT in hemorrhagic patients is widely supported,Citation25,Citation26 but transfusion for prophylactic purposes in thrombocytopenic cancer patients has raised several concerns, not only regarding reduced functionality and efficacy of transfusion outcomes, usually determined by Corrected Count Increment (CCI), but also to the possibility of inducing adverse effects.
For instance, Cryo-PLT show increased activation after thawing, which results in morphological changes and massive release of soluble bioactive molecules from α-granules.Citation27 These molecules are known to play a role in the tumor microenvironment, being associated with cell proliferation, angiogenesis and epithelial–mesenchymal transition (EMT).Citation28 Additional concerns on the prophylactic transfusion of Cryo-PLT to cancer patients are related to their possible pro-thrombotic effect and to the accumulation of undesired metabolites in the supernatants.Citation28,Citation29
Despite Cryo-PLT have been extensively characterized,Citation27,Citation30,Citation31 only a few time points after thawing have been evaluated, and only for buffy coat-derived platelet concentrates.Citation32 The effects of Cryo-PLT on cancer cell proliferation and migration are not yet known, although recent studies have shown that subpopulations of Cryo-PLT bind to cancer cells.Citation33
Identifying the exact transfusion timing and evaluating the effect of Cryo-PLT supernatants are essential steps to reduce transfusion risk in cancer patients. Through a multidisciplinary approach, we primarily focused on investigating the effect of time after thawing on the biochemical characteristics of cryopreserved platelets for transfusion timing optimization. It also encompassed a secondary objective of assessing the influence of these platelets on cancer cell behavior in vitro, providing a more comprehensive understanding of their potential implications in cancer-related processes.
Our findings would provide valuable insights into the potential of Cryopreserved platelets as a valuable resource for transfusion support in the prevention of bleeding, such as in onco-hematological patients.
Methods
Study design and platelets collection
The study was carried out by the Transfusion Medicine Unit of the Azienda USL-IRCCS di Reggio Emilia, approved on 17 December 2020 (protocol number 2020/0149618) and emended on 26 July 2021 (protocol number 2021/0094053) by the Institutional Board Review (Reggio Emilia Ethics Committee).
Five platelet donors were recruited by our Transfusion Medicine Unit at “Casa del Dono” in Reggio Emilia (Italy). All recruited donors signed an informed consent according to the Declaration of Helsinki. Platelet apheresis procedures were performed using an automated blood collection system (Mobile Collection System MCS+, Haemonetics Corp., Boston, MA, USA), according to the manufacturer’s instructions. Acid Citrate Dextrose Solution A (ACD-A, Haemonetics Corp., USA) was used as anticoagulant; plasma and platelet additive solution (T-PAS+, Terumo) were added in a ratio as 30:70. After collection, PLT units were irradiated with a delivered dose of 35 Gy (Raycell) before Cryopreservation procedure according to Italian law.Citation34
PLT units (n = 5) were Cryopreserved without the use of a controlled freezing rate protocol within 24 hours from collection according to Valeri et al.Citation35 Briefly, a sterile solution of 25% Dimethyl Sulfoxide (DMSO, Sigma Aldrich) in 0.9% Sodium Chloride (NaCl, Baxter) was added to each PLT unit to achieve a DMSO final concentration of 6%. Next, the PLT units were equally divided into three satellite 300 mL PVC made Teruflex® bags (Terumo) and subjected to centrifugation at 1349 g for 10 minutes at 20°C using Rotixa 50 RS (Hettich Zentrifugen). After removing the supernatant, a PLT pellet of approximately 10 mL ± 1,9 was stored at −80°C using the MDF-U5386S freezer (Sanyo). Cryo-PLT were stored for a mean of 200 ± 30 days before analyses.
Each satellite Cryo-PLT units was thawed at 37°C in water bath and then reconstituted with 70 mL platelet additive solution (T-PAS, Terumo). For each donation, donor plasma was also collected separately. Experimental plan is reported in .
PLT characterization
All characterization analyses were performed on both fresh (control samples, T0) and thawed products. Fresh-PLT were stored at 22–24°C, with constant agitation for not more than 5 days. Thawed, reconstituted PLT were kept at 22–24°C in agitation and samples (15 mL) were collected 1 h, 3 h and 6 h post-thaw for further analysis. Fresh-PLT (5 × 10Citation6/mL) activated with thrombin (0.5 U/mL) for 10 minutes at 37°C (Midi CO2 Incubator, ThermoScientific) were also assessed as positive control.
Platelet counts and ROTEM assay
PLT count was performed on Fresh and Cryo-PLT immediately after thawing by using a blood cell counter (XS-1000i analyzer, Sysmex). Post-thaw recovery was calculated using the following formulaCitation36:
Platelet clot viscoelastic parameters were evaluated on Fresh-PLT and thawed Cryo-PLT by thromboelastometry on ROTEM® delta (Werfen, Milano, Italy) device. A volume of 350 µL of PLT was diluted with 150 µL of autologous plasma. Intrinsic, extrinsic and contact-mediated coagulation paths were evaluated using INTEM, EXTEM and NATEM tests respectively, according to the manufacturer’s instructions. The following thromboelastometric parameters were assessed: Clotting Time (CT, sec) the time until clot formation starts; Clot Formation Time (CFT, sec) the time from CT until the clot reaches an amplitude of 20 mm; A20 (mm) value indicating the clot amplitude 20 minutes after CT; Maximum Clot Firmness (MCF, mm), defined as the maximum amplitude reached by clot formation during measurement.
Flow-cytometry
Surface receptors, activation markers (P-selectin, CD62P) and phosphatidylserine (PS) expression were analyzed with the FACSLyrics flow cytometer (BD, Bioscience). All reagents are from BD Biosciences, unless otherwise specified.
Glycoproteins expression was carried out adding 20 µL of anti-CD41a-PE, anti-CD42b-FITC, anti-CD62P-APC and 5 µL of anti-CD61-PerPC to 100 µL of platelets (5 × 10Citation6/mL).
Samples were incubated at RT in the dark for 15 minutes and then 400 µL of PBS (EuroClone) was added before acquisition.
PS exposure was assessed using AnnexinV-FITC. In brief, samples were diluted in PBS to reach 200.000 PLT/mL concentration, centrifuged at 1500 g for 5 minutes and resuspended in 200 µL of Annexin VBinding Buffer. 5 µL of Annexin V-FITC were added to 100 µL of samples, incubated in the dark for 15 minutes and then resuspended in 400 µL of Annexin V binding buffer for flow cytometer analysis.
PLT microparticles were enumerated exploiting the customized extracellular vesicles (EVs) kit for flow cytometry (BD Biosciences, #626267, Custom Kit)Citation37 according to manufacturer’s. A reagent mix was prepared for each sample as follows: 94 µL of 0.22 μm-filtered PBS, 0.5 µL of Lipophilic Cationic Dye, 0.5 µL of FITC-conjugated phalloidin, 5 µL of CD41a-PE and centrifuged at 22.000 g for 15 minutes (Centrifuge 5424, Eppendorf). The mix was added to 100 µL of PLT (150.000/µL) previously dispensed into a TrueCount™ Tube (BD). After 45 minutes of incubation (RT, in the dark), 1 mL of filtered PBS was added and vortexed (ZX3, Velp Scientifica) immediately before the acquisition.
FTIR spectroscopy
Fourier Transform InfraRed (FTIR) spectroscopy was performed at the SISSI-Bio beamlineCitation38 at Elettra Sincrotrone Trieste, in order to assess protein and lipid structure of both Fresh and Cryo-PLT. PLT samples were diluted 1:10 with 0.9% NaCl and fixed with 0.5% PFA (Promega) for 15 min, at RT. Samples were then centrifuged for 5 min at 1500 g (Centrifuge 5424, Eppendorf), resuspended in 500 µL of 0.9% NaCl and left at 4°C (Recorder fridge, Medical Project) until the analysis. Samples were deposited onto a CaF2 window, 1 mm thick and 13 mm in diameter, dried inside a laminar flow biological hood and then measured. The measurements were carried out using a Bruker Hyperion 3000 IR/VIS microscope coupled to an in-vacuum interferometer VERTEX70V (Bruker Optics – Billerica MA, US) with a single-point MCT (Mercury Cadmium Telluride) detector. The microscope apertures were closed at 50 × 50 microns in order to select small groups of PLT. A total of 1366 spectra were collected. Each spectrum is the average of 256 scans, at 2 cm−1 of spectral resolution, with a scanner velocity of 40 kHz. After the recording, spectra were corrected for the atmospheric water vapor and CO2 with a routine embedded in OPUS 8.5 SP1 (Bruker Optics – Billerica, MA – US) and imported in QUASAR (http://quasar.codes).Citation39 With the modules present in this software, data were baseline corrected, 2nd degree derivative was calculated using Savitzky-Golay filter (31 point of smoothing and 3rd degree polynomial function) and normalized with Standard Vector Normalization (SVN). Using the baseline corrected absorbance spectral data the following spectral ratio were calculated:
C=O/Lipids (Area 1760–1700 cm−1/Area 3000–2800 cm−1)
C=O/Amide I (Area 1760–1700 cm−1/Area 1715–1590 cm−1)
CH2/CH3 (Area 2860–2840 cm−1/Area 2945–2910 cm−1)
NucAcids/Amide I (Area 1278–1200 cm−1/Area 1715–1590 cm−1)
Results were then imported in Origin(Pro)2022 (Origin Lab Corporation, Northampton, MA, USA) and the averages were compared using an ANOVA, according to the class of interest, being it time (Fresh, 1 h, 3 h and 6 h) or activation status.
A Principal Component Analysis (PCA) was performed on 2nd derivative normalized spectral data considering the ranges between 3200 and 2800 cm−1 and 1800 and 900 cm−1.
ELISA and Luminex assays
TGF-β (Transforming growth factor beta) concentration (pg/mL) was quantified by means of ELISA (Transforming growth factor beta ELISA Kit, R&D system, Biotechne), following the manufacturer’s instructions. Each measure was performed on PLT supernatant and tested in triplicate against a standard curve. Samples in 96-well plates were read at 450 nm (Glomax® Discover Microplate Reader, Promega, Madison, WI, USA).
Luminex technology (Bio-Plex Pro Human Cytokine Screening Panel Kit, BIORAD) was exploited to evaluate the concentration of RANTES, PDGFB and FGF (pg/ml) in the PLT supernatants. To obtain 1 h PLT supernatants, fresh and thawed PLT samples were subjected to centrifugation at 1500 g for 20 minutes at 10°C. Subsequently, a second centrifugation at 10 000 × g for 5 minutes was performed to obtain PLT-free supernatant. Following this, the pellet was resuspended in a fresh resuspension solution and subjected to another round of centrifugation to measure the delta release at 3 hours after thawing. This identical procedure was repeated for the subsequent time point of 6 hours after thawing.
The resulting supernatant was stored at −80°C with 1:200 protease inhibitor cocktail (Abcam, MA, USA) until further analysis. Measures were performed following the manufacturer’s instructions and assessed in triplicate against a standard curve. Samples in 96-well plates were read with Bio-Plex MAGPIX® Multiplex Reader (Luminex XMAP Technology, BIORAD, Hercules, CA, USA).
Cell culture and cell proliferation analysis
The human breast cancer cell line MCF-7 was chosen as breast cancer model to mimic solid tumors in vitro.Citation40,Citation41 MCF-7 were cultured in low glucose Dulbecco’s modified Eagle’s medium (DMEM Low Glc, EuroClone) supplemented by 10% fetal bovine serum (FBS, EuroClone), 1% L-Glutamine (EuroClone) and 1% Penicillin-Streptomycin (Pen-Strep, Sigma Aldrich). Complete growth medium was renewed 2–3 times per week and cell confluency was kept approximately under 80% in T75 flasks (CytoOne).
The human myeloid leukemia cell line HL-60 was cultured in RPMI 1640 medium (EuroClone) added with 10% FBS (EuroClone) and 1% Pen-Strep (Sigma Aldrich).
PLT (1×10Citation6/mL) samples were centrifuged twice at 10 000 g for 5 minutes (Centrifuge 5424, Eppendorf) to obtain Fresh-PLT supernatant (Fresh-PLT) and Cryo-PLT supernatant (Cryo-PLT).
Cell viability of MCF-7 and HL-60 was measured by WST-1 cell proliferation assay (Cell Proliferation Reagent WST-1, Merck). Experimental conditions are detailed in . MCF-7 and HL-60 cells were seeded at a concentration of 15 000 cells/cmCitation2 and 30 000 cells/mL respectively, into 96well-plates (EuroClone); after 24 hours cell culture media were replaced with the conditioned media listed in . Proliferation was assessed after 24, 48 and 72 h of incubation (Midi CO2 Incubator, ThermoScientific) by addingWST-1 to the cells for 2 h at 37°C (Midi CO2 Incubator, ThermoScientific) prior reading at 450 nm (GloMax Discover, Promega).
Table I. Experimental culture conditions.
Cell migration and cells-platelets adhesion assay
Wound healing assay was performed to monitor MCF-7 migration. MCF-7 cells were seeded in culture medium at a concentration of 75 000 cells/cm2 and allowed to grow for 24 h to reach approximately 100% confluence. Then, the medium was removed and a linear wound was generated in the monolayer with a sterile 10 µL plastic pipette tip (ClearLine, Dominique Dutscher). Wells were washed twice with PBS to eliminate cellular debris and new medium was added following the conditions indicated in . Digital images of cells’ clear area were acquired right after wound generation (0 h) and at 24, 48, and 72 h at 4X magnification. The data were analyzed using ImageJCitation42 software and the results of five replicates for each of the four conditions were expressed as percentage of wound closure.
Platelets-cells adhesion assay was performed as previously described.Citation43 Briefly, MCF-7 and HL-60 cells were resuspended at the concentration of 1 × 106 cells/mL in culture medium containing 1 mM CaCl2 and 0.1% FBS. Subsequently, 2 × 105 cells/mL cells were incubated in agitation with 2 × 108 platelets/mL for 30 min at 37°C. Staining with anti-CD41a-PE antibody (BD Biosciences) was performed to discriminate platelets from cancer cells (30 min at RT, in the dark) before flow cytometric analysis (FACSLyrics system, BD Biosciences). MCF-7 and HL-60 cells resuspended in PBS were used as negative control (CTRL).
Statistics
Statistical analysis was performed using GraphPad (Prism 8.4.2, GraphPad Software, San Diego, CA, USA). One- way or two-way ANOVA with multiple comparisons test or Benjamini correction were performed to assess difference among samples. A P-value of <.05 was considered statistically significant. Flow cytometry data were analyzed with FacSuite Software (BD Biosciences).
Results
Cryopreservation affects coagulation capacity and activation profile of Cryo-PLT vs. Fresh-PLT
To determine platelets recovery after cryopreservation, we compared platelet count before freezing and after reconstitution of the thawed units in T-PAS as resuspension media. The mean count in fresh samples and after thawing, was 1206 ± 160 × 10Citation3/μL and 983 ± 189 × 10Citation3/μL, respectively, with a recovery of 81%.
We compared the hemostatic activity of Fresh- and Cryo-PLT after 1, 3 and 6 hours from thawing (both samples were resuspended in T-PAS) by assessing the ROTEM standard parameters (CT, CFT, MCF).
All ROTEM parameters resulted to be impaired after thawing (ANOVA overall effect, p < .01). INTEM and EXTEM analysis revealed a prolongation of the CT and CFT after cryopreservation in comparison to Fresh-PLT (). When comparing post-thawed samples, CT increased significantly after 6 hours of cryopreservation compared to 1 h and 3 h samples for INTEM and NATEM assays, respectively (). Cryopreservation also affected the strength of the PLT clot compared to Fresh-PLT, as shown by the decrease of the MCF () and of the clot amplitude at 20 min from CT (Figure SD1).
Figure 2. Cryopreservation affects coagulation capacity and activation profile of Cryo-PLT vs. Fresh-PLT. (a) clotting time (CT) (b) clot formation time (CFT) and (c) maximum clot firmness (MCF) for INTEM, EXTEM and NATEM assays in Fresh- and Cryo-PLT analyzed at different time points after reconstitution (1 h, 3 h and 6 h). Data are represented as median with interquartile ranges. (d) overlay cytogram of CD62P+ PLT in: fresh non activated samples (Fresh-NA, black), PLT activated with thrombin (Fresh-A, light blue) and Cryo-PLT after 1 h from thawing (1 h, light gray). (e) CD62P f) CD42b (g) and CD41a exposure on Fresh-(NA and A) and Cryo-PLT at different time points after thawing (1 h, 3 h and 6 h). Data are represented as percentage % of positive events and reported as mean ± SD. (h, i, j) gating strategy to discriminate CD41a+/CD42b+ (green dots) and CD41a+/CD42b− (fuchsia dots); k,l,m) CD62P expression PLT in Fresh-(NA and A) and Cryo-PLT based on gated population CD41a+/CD42b+ (green curve) and CD41a+/CD42b− (fuchsia curve).Statistical analysis was performed using one-way ANOVA with multiple comparisons *p < .05, **p < .01, ***p < .001, ****p < .0001. Black * and lines indicate significant differences from the Fresh-PLT, while gray * and lines indicate differences among time points after thawing.
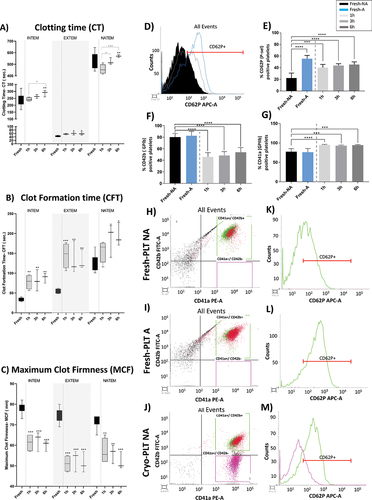
We then performed flow cytometry analysis to evaluate the exposure of CD62P activation marker after cryopreservation: obtained data were compared with the activating effect of thrombin on fresh samples. In non-activated (NA) samples, CD62P-associated median fluorescence channel (MFC) values increased from 296.2 ± 19.4 of Fresh-PLT (, black area) to 405.5 ± 41.7 of Cryo-PLT at 1 h post thaw (, light gray line) which was accompanied by an increase of CD62P-positive events in Cryo-PLT for all the after thawing time points analyzed (). However, CD62P exposure of Cryo-PLT was less evident compared to activated Fresh-PLT (Fresh-A) which showed an MFC of 593.0 ± 154.6 (, light blue) and a percentage of 56.1% ± 5.0 (, light blue) of CD62P-positive events. Of note, Cryo-PLT were unable to respond to thrombin and to induce CD62P exposure (Figure SD2).
Cryopreservation resulted in a decrease of CD42b-positive platelets and in an increase of CD41a-positive platelets compared to Fresh-PLT (). A mild decrease of CD61-positive PLT was also observed (see Table SD1), whereas agonist produced an increase of only fresh-induced samples.
Intriguingly, the decrease of CD42b-positive platelets after cryopreservation was accompanied by a CD41a-positive/CD42b-negative platelet subpopulation (, fuchsia dots), which was also negative to CD62P (, fuchsia lines). On the other hand, fresh activated PLT expressing both CD41a and CD42b (), were also positive to CD62P, while Fresh-PLT NA () were not. These data indicate that cryopreservation induces a significant change in the activation pattern of platelets which may lead to a deterioration of clotting capacity.
PS exposure and EVs shedding are linked to membrane modifications occurring over time after thawing
We evaluated the PS exposure and extracellular vesicles (EVs) release by means of flow cytometry. As reported in , Cryo-PLT samples 1 h after thawing (1 h, light gray) showed an increase of PS-positive platelets (77.0 ± 8.4%) compared to non-activated Fresh-PLT (Fresh-NA, 36.5 ± 4.2), with percentages comparable to fresh activated samples (Fresh-A, 73.0 ± 5.5). Although still statistically significant, this increase declined over time after thawing (3 h and 6 h). Similar results were obtained with the EVs quantification assay reported in , that showed a 38-fold EVs increase in 1 h post-thaw Cryo-PLT over the non-activated Fresh-PLT (Fresh-NA). Of note, cryopreservation induced a 7-fold increase of EVs also when compared with thrombin-activated Fresh-PLT (Fresh-A). Again, EVs release decreased slightly at 3 and 6 hours after thawing (Figure SD3b and Table SD2) but the differences were not significant. Thrombin-stimulated platelets showed no statistical difference before and after thawing at any of the time points evaluated for both PS exposure (Figure SD3a) and EVs formation (Figure SD3b and TableSD2).
Figure 3. PS exposure and EVs shedding are linked to membrane modifications occurring over time after thawing. (a) Annexin-V positive events recorded in Fresh-(NA and A) and Cryo-PLT at different time points after thawing (1 h, 3 h and 6 h) evaluated by flow cytometry and reported as percentage (left panel) mean ± SD (right panel) (b) extracellular vesicles enumeration (EVs/µL) derived from Fresh-PLT (NA and A) and Cryo-PLT analyzed by flow cytometry after thawing (1 h, 3 h and 6 h). One-way ANOVA with multiple comparisons was used to evaluate statistical significance: **p < .05; ****p < .0001. (c) CH2/CH3 ratio; (d) proteins/lipids (Amide I/CH2-CH3 stretching bands) ratio; (e) carbonyl/proteins (C=o/amidei) ratio (f) Carbonyl/lipids (C=o/lipids) ratio in Fresh-(NA and A) and Cryo-PLT at different time points after thawing (1 h, 3 h and 6 h) evaluated by FTIR. (g) scatter plots of the PCA scores for PC1 and PC3, representing the 43.5% and 7.4% of variance, respectively. Centroid and distribution comparison of (h) PC1 and (i) PC3 respectively. (j) loading vectors of PC1 (black) and PC3 (red). *p < .05, **p < .01, ***p < .001, black * and lines indicate significant differences calculated with Tukey method. Data are presented as box 25–75% range of the data, whiskers range within the 1st quartile, line: median line, square: mean, black diamonds: outliers. FTIR unsupervised principal component analysis (PCA).
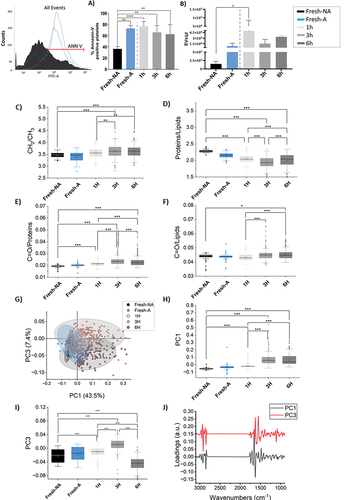
FTIR spectroscopy was performed to evaluate the influence of cryopreservation on membrane phospholipids and protein folding. After collection and pre-processing, data were analyzed using both univariate and multivariate approaches. The average absorbance spectra of the data are shown in Figure SD4 of the supplemental data. At a first glance, no major differences could be spotted among the four spectra of Fresh-PLT and Cryo-PLT at 1 h, 3 h and 6 h after thawing. Therefore, specific bands ratio were calculated (). The complete range of CH2-CH3 stretching bands (2800–3000 cm−1) was used to evaluate the lipid content, while the ratio of the single asymmetric stretching bands of CH2 and CH3 was used to evaluate the membrane relative composition, integrity and stiffness.Citation44 In the CH2/CH3 ratio increased with time after thawing; Fresh-PLT, 1 h and 3 h Cryo-PLT values were significantly different, whereas 3 h and 6 h were not. Since this ratio is associated to an average membrane composition or stiffness, this trend indicated that the membrane became stiffer at the different time points after thawing.
The proteins/lipids ratio () was used to evaluate the variation of protein material among the PLT samples with time. In this case, we observed a loss of proteins after thawing, which was noticeable up to 3 h, and then at 6 h became stable.
show the lipid and protein peroxidation status by assessing the C=O/proteins and C=O/lipids ratios, respectively. Both values increased with time after thawing, indicating a possible ongoing mild oxidative process. Again, no clear distinction could be made between 3 h and 6 h.
To highlight subtler spectral variations that were not appreciable in the absorbance data, we performed a Principal Component Analysis (PCA) on the normalized second derivative spectra.Citation45 In the score values of the acquired spectra on PC1 (x) and PC3 (y) are plotted as a scatterplot. With this representation, Fresh-PLT and Cryo-PLT at 1 h overlap, even though, from the projection representation of the same data on their respective Principal Component (), their centroid and distribution are significantly different (p ≤.001). Data at 3 h and 6 h after thawing are separated from Fresh-PLT and those at 1 h after thawing along PC1, whereas PC3 separates 3 h (upper quadrant) from 6 h (lower quadrant). In order to identify the spectral features that explain the grouping in different zones of the PCA plane of the data, loading vectors have been plotted in . The PC1 vector that represents 43.5% of the data has the strongest signals in the protein region, one positive at 1655 cm−1 and one negative at 1625 cm−1; the first can be assigned to proteins in α-helix conformation, which were found to be higher in Fresh-PLT samples and Cryo-PLT after 1 h from thawing, while the second can be assigned to proteins in β-sheet conformation, higher in Cryo-PLT samples at 3 h and 6 h. Therefore, we can hypothesize that the protein components of the platelets undergo a partial rearrangement of folding already 1 h after thawing, probably due to some oxidative processes ()
Spectral features on PC3, that explain 7.4% of the variance within the data, mainly refer to the separation between 3 h and 6 h samples. In this case, the more intense signals are in the range of lipids aliphatic chains (CH2-CH3 stretching bands, 2800–3000 cm−1), and precisely at 2918 and 2848 cm−1. These signals are similar for Fresh-PLT (NA or A) and 1 h samples, whereas at 3 h from thawing are higher and lower at 6 h. Other two strong positive peaks are in the protein region at 1625 cm−1 for the Amide I and 1550 cm−1 for the amide II. Similarly, to the assignment of this peak for PC1, these signals can be read as a hint of a lower misfolding of platelets at 3 hours from thawing (3 h) compared to those at 6 hours (6 h).
Cryopreservation induce platelet degranulation
We evaluated platelet degranulation by comparing the amount of clinically relevant soluble factors in the PLT supernatant of Fresh- and Cryo-PLT. We found a slight, but significant, increase of soluble mediators 1 h after thawing (); the most relevant increase was detected for RANTES (55% increment after thawing). On the other hand, TGF-β (Figure D) exhibits an opposite trend, with a reduction of its concentration in cryopreserved supernatants compared to Fresh-PLT. Release of soluble factors was not evident at subsequent time points (data not shown).
Cryo-PLT has lower pro-tumoral effect and adhesive capacity compared to Fresh-PLT in MCF-7 and HL-60 cell lines
We used MCF-7 and HL-60 cancer cells to investigate the effect of Fresh and Cryopreserved PLT supernatants on cancer cell proliferation by means of WST1 (see for details on culture conditions). For these experiments, we tested only 1 hour after thawing based on soluble mediators data (). Although no statistically significant differences were found between the conditions examined, shows that MCF-7 cells treated with Fresh- and Cryo-PLT supernatant had a proliferation pattern compared to the negative control (CTRL-). On the other hand, supernatant from Fresh-PLT was able to induce proliferation of HL-60 cells at 72 h, similarly to positive control (CTRL+); supernatants from Cryo-PLT instead, were always comparable to the negative control ().
Figure 5. Effect of PLT supernatants on cancer cells behavior in vitro. Cell proliferation profile of (a) MCF-7 and (b) HL-60 cell lines conditioned with the Fresh- and Cryo-PLT supernatants evaluated by WST1 assay after 24, 48 and 72 h of seeding. (c) analysis of MCF-7 cells migration by in vitro wound healing assay. Quantification of the wounded area invaded by MCF-7 and time-lapse representative microscopy images of MCF-7 cells after 24, 48 and 72 h of conditioning with PLT supernatants. Data are reported as percentage (%) of wound closure at the baseline (T = 0 h after treatment). Results are reported as mean ± SD of three measurements of the wounded area, obtained in 5 independent experiments (n = 15). The dotted lines define the area lacking cells. Scale bars, 100 μm. (d) PLT- cancer cells adhesion. Percentage of CD41a-positive cells (MCF-7 or HL-60) after being conditioned with Fresh- or Cryo- PLT supernatant. Control (CTRL) are same cells w/o any conditioning. Data are reported as mean ± SD of three independent experiments. Statistical analysis was performed using one-way ANOVA with multiple comparisons *p < .01, **p < .005.
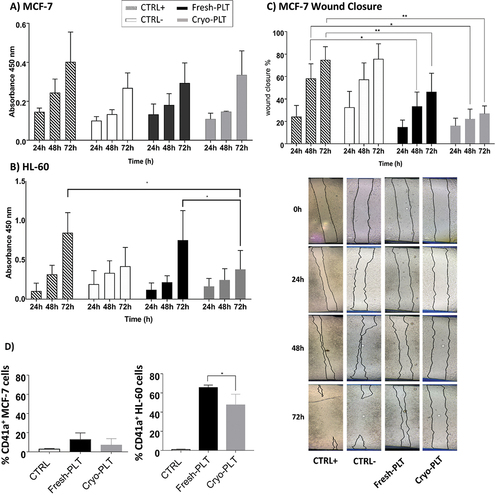
To evaluate the effect of PLT supernatants on MCF-7 cells migration, we performed a scratch wound healing assay. shows that cells treated with PLT supernatant had a slower overall wound closure rate after 72 hours than both positive and negative controls. Although not statistically significant, a slight difference between Fresh- and Cryo-PLT was found after 72 hours, with the wound closure higher upon treatment with Fresh-PLT.
To evidence PLT ability to bind cancer cells, MCF-7 and HL-60 cells were incubated for 30 minutes with Fresh or Cryo-PLT and then stained CD41a fluorescent antibody before flow cytometry acquisition. The percentage of CD41a-positive events, representing the cancer cells coated by platelets on their surface, is reported in . Cells without pre-treatment with PLT are shown as negative control (CTRL). Platelets, either fresh or thawed, were unable to adhere to MCF-7 cells, while the HL-60 displayed a high percentage of CD41a-positive events. Of note, Cryo-PLT display a reduced ability to interact with cancer cells, as demonstrated by the lower number of CD41a-poisitive events in the HL-60 region (, panel D).
Discussion
Platelets must be stored in blood banks at RT, limiting the shelf life to only 5–7 days because of the risk for bacterial growth during storage.Citation5 Platelet cryopreservation could be a valid alternative to room-stored PLT, and some in vivo studies have shown its safety and efficacy in the context of surgery.Citation26,Citation46,Citation47 However, the generation of platelet storage lesions due to either cryoprotectant toxicity, warming procedure or resuspension media can significantly affect platelet functions.Citation4 It is well established that reduced platelet function is significantly associated with bleeding risk and reduced platelet circulating time in the recipients.Citation20 Other concerns relate to the potential for the indiscriminate release of growth factors and cytokines into the circulation or an increase in the risk of thrombosis and hypercoagulability.Citation48–50
In order to identify additional key parameters of platelet functionality that may help understanding if Cryo-PLT could represent an effective alternative to Fresh-PLT for onco-hematological patients, we performed an in-depth biochemical characterization of Cryo-PLT. We considered the events that occur over time after thawing and that may affect platelet morphology, coagulation capacity, functionality and responsiveness to physiological stimuli. Furthermore, we evaluated the extent of platelet interaction with cancer cells and the effect of PLT supernatants on cancer cell behavior in vitro.
Dynamic information on the speed of coagulation initiation, kinetics of clot growth and clot strength were obtained by ROTEM analysis reporting an alteration of the coagulation process. In particular, clotting capacity and efficiency were reduced by cryopreservation for all the coagulation pathways investigated (): the decrease of the clot strength and stability was accompanied by an increase of the time necessary to generate a stable clot evidenced by increase of the CFT and decrease of the clot amplitude. However, the overall clotting capacity did not change over time after reconstitution and always remained within the clinically accepted range.Citation51 Nevertheless, our data partially contradict previous studies demonstrating a faster CT and pro-coagulant phenotype of Cryo-PLT,Citation11,Citation52,Citation53 reporting that prolonged storage of post-thawed platelets only slightly reduces hemostatic function in vitro.Citation11,Citation32,Citation54,Citation55 Conflicting results have been published for clot formation time. Some groups have found a decrease in this parameter after cryopreservation, while others have found no difference among different platelet frozen methods.Citation55–57 Such discrepancies could be explained, among others, by the agonist used to perform hemostatic assay, e.g. kaolin or tissue factor, the differences in the methods of preparation, platelet concentrationCitation58,Citation59 and resuspension media.Citation60 Specifically, the effects of additive solutions and the substances they contain can affect the preservation and physicochemical properties of platelets.Citation17,Citation60 Our study focuses on 100% platelet additive solution as a resuspension medium whereas the vast majority of available studies focus on plasma-based solutions.Citation47,Citation61 Johnson et al. compared Cryo-PLT reconstituted in plasma and platelet additive solution demonstrating that plasma-reconstituted Cryo-PLT shows a more pro-coagulant behavior.Citation36,Citation56
The reduced clotting activity was also accompanied by a glycoprotein pattern modifications occurred after thawing (). According to what was first reported by Valeri and colleagues,Citation62 our data confirmed that cryopreservation induces a loss of CD42b exposure, a key receptor of the hemostatic and coagulation cascade.Citation63 This decrease is accompanied by a reduced responsiveness to thrombin activation, as depicted in Figure SD2. These results align with similar findings observed in cryopreserved platelet concentrates derived from buffy-coat and resuspended in a solution containing 30% plasma and 70% additive solution.Citation33 The CD41a+/CD42b− platelet sub-population observed in Cryo-PLT was negative for P-selectin (), confirming the hypothesis that CD42b exposure is necessary to switch to the active phenotype.Citation62 Based on CD42b/CD62P staining, Johnson and colleagues recently reported that only the 23 ± 7% of cryopreserved platelet would be defined as pro-coagulant.Citation31 Accordingly, we found only a 25% of CD42b+/CD62P+ activated platelets in the Cryo-PLT at 1 h after thawing. Finally, we saw a higher exposure of CD62P in thawed platelets that, coupled with the ROTEM results discussed earlier, may be explained by an increased degranulation and release of mediators from the α-granules rather than by a higher coagulation activity.Citation64
It has been reported that platelet morphology is significantly affected after cryopreservation.Citation56,Citation57,Citation64,Citation65
However, most of the evidences regard structural changes and do not investigate how cryopreservation quantitatively alter the biological components of PLT.Citation64,Citation66,Citation67 Here, we obtained important clues on the macromolecular changes induced by cryopreservation on membrane lipids, protein content and increasing of peroxidation processes. Indeed, FTIR data demonstrate how time after thawing affects membranes’ functional characteristics and integrity. Intriguingly, these alterations proceeded over time after thawing with the maximum morphological deterioration occurring within the first 3 hours (). We found morphological changes in the plasma membrane composition, with increased membrane stiffness (CH2/CH3 ratio) and loss of protein content (protein/lipid ratio) after cryopreservation. On the other hand, both C=O/lipids and C=O/proteins ratios increased over time after thawing possibly because of peroxidation, which may eventually be responsible of impaired platelet function.Citation44,Citation68 The PCA results () also revealed that the thawing process impairs protein folding and membrane phospholipids structure. In particular, protein structure seems to be maintained within the first hour after thawing, as inferred by signals of α-helix bands, which is stronger for Fresh and Cryo-PLT at 1 h; however, 3 hours after thawing the strongest signal was related to the β-structures, the accumulation of which is a marker of protein misfolding and cell function impairmentCitation44.
According to previous studies,Citation27,Citation57,Citation69 we found that cryopreservation produced an increase secretion of platelet derived EVs accompanied by an increase of PS externalization compared to fresh components. Both increases declined over time after thawing (, 3 h and 6 h). We hypothesize that the decrease of PS-positive platelet counts and the EVs release of post-thawed Cryo-PLT, mirror the increased peroxidation process and protein misfolding and account for the alteration of membrane integrity and stiffness, which occur irreversibly 3 hours after thawing, and for the reduced clotting capacity of Cryo-PLT, despite the strong EVs release at short times. Altough, PS externalization has been largely recognized as a unique marker of pro-coagulant platelets among the subpopulation of activated platelets, recently 3 surface markers have been recommended to be used in order to differentiate pro-coagulant platelets from apoptotic platelets.Citation31,Citation70,Citation71 Accordingly, the higher exposure of PS found in Cryo-PLT is probably caused by the reduced viability as confirmed by the fact that Cryo-PLT are less responsive to physiological agonist treatment (, Fresh-A vs. 1 h).Citation72,Citation73
Several studies suggest that EVs support blood coagulation,Citation74–76 however recent investigations have challenged earlier work regarding the pro-coagulant activity of EVs. A recent study by Berckmans et al. revaluated their previous findings on the coagulant properties of EVs in blood.Citation77 In comparison to their earlier study, they could detect a fibrinolytic activity associate to EVs, thereby pointing to anti-coagulant functions. These conflicting results may be explained by the existence of different EVs subsets or by numerous subpopulations of EVs (from platelets or other cellular lineages) present in the blood, associated with either pro- or anti-coagulant activity.Citation31,Citation78 It can also be hypothesized that it is the plasma rather than the platelets themselves that retain the main effect and that EVs are only effective in the presence of plasma. Thus, although the promotion of coagulation was the first function attributed to EVs, extensive research dedicated to understanding their role is needed.
The membrane impairment induced by cryopreservation, together with the analysis on glycoproteins exposure, might also explain the results obtained on soluble mediators releases from the α-granules. Among all the biomolecules assessed (see Materials and Methods for details), the main differences between Fresh- and Cryo-PLT were found after 1 h form thawing for FGF, PDGF-BB, RANTES (CCL5) and TGF- β. Subsequently, the residual concentrations of these molecules dropped below the limit of detection (see Material and Methods for details). Again, these data must be read according to the type of solution in which the platelets are reconstituted after thawing or the platelet number. Cardigan and colleagues showed that parameters related to platelet activation (e.g., TGF-β, CD62P) and cytokine release markers (e.g., IL-8) can change depending on PAS composition and that modification of the quality and concentration of the different components of PAS can improve both the storage and safety profiles of platelet concentrates.Citation79 In a previous study on cryopreserved platelet concentrates for non-transfusion purposes,Citation80 we observed that the release of growth factors did not correlate with platelet numbers, suggesting that it is more likely dependent on donor variability and specific factors related to the freezing and thawing process. This observation underscores the complexity of the interactions between cryopreservation, platelet concentrations, and the release of soluble mediators. All the evaluated factorsCitation26,Citation81,Citation82are involved in cancer progression, although a bidirectional effect on tumor progression has been reported for RANTES and TGF-β.Citation83 Nevertheless, it has been demonstrated that TGF-β, when originating from platelets, is the solely responsible for inducing an invasive epithelial–mesenchymal transition to metastasis.Citation84,Citation85
Based on these data and knowing that platelets play a key role in tumor progression and metastasis,Citation40,Citation86 we evaluated the capability of Cryo-PLT to stimulate in vitro models of cancer. We found that Cryo-PLT have lower pro-tumoral effect compared to Fresh-PLT evidenced by cell migration impairment (), decrease of cell proliferation () and lower ability to interact and aggregate with HL-60 (). On the other hand, although Fresh-PLT are more prone to induce cell growth, they showed only moderate stimulation of proliferative capacity compared to the positive control (). Thus indicating that viability is necessary for effective and functional platelet–tumor interaction. Accordingly, the reduced amount of TGF-β in Cryo-PLT could explain the reduced migration capability of Cryo-PLT compared to Fresh-PLT. Our data is partially in agreement with those of Pu et al.,Citation28 reporting an inhibitor effect on cell growth by platelet supernatants; these authors attributes this effect to the accumulation of toxic metabolites over time after collection. Collectively, these pieces of evidence suggest that Cryo-PLT are not more prone to stimulate neither solid nor liquid tumor cells. Therefore, platelet releasate of Cryo-PLT does not seem to affect cancer cells behavior.
With our study, we proved that cryopreserved platelets from apheresis donation, collected and resuspended in platelet additive solution, undergo marked morphological changes that impair their integrity. We showed indeed that, after thawing, Cryo-PLT progressively accumulate features ascribable to oxidative stress and PSL with reduced functionality, and increase of PS externalization, peroxidation processes and protein misfolding.
Despite platelets and their soluble factors are known to play a key role in tumor progression and metastasis,Citation40,Citation86 our results on the effect of Cryo-PLT on in vitro models of cancer cells suggest that this product can be considered safe for the transfusion of onco-hematological patients with not sufficient capability to promote a higher grade of proliferation, cell adhesion or migration. Nevertheless, Cryo-PLT may thus be considered a precious back-up product to be use during periods of Fresh-PLT shortage to prevent bleeding in non-hemorrhagic patients. It is desirable to make it logistically feasible to transfuse cryopreserved platelets within one hour of thawing to maintain the platelets in their best performing condition.
We are aware that our study present some limitations. Despite the efforts to control for confounding variables, there is a possibility that unaccounted or unknown factors could have influenced the observed results, aside from the freezing process itself. These factors may include variations in sample collection, processing techniques and storage conditions that were not directly accounted for in our study design. We also acknowledge that the generalizability of our study is limited due to the specific conditions, the small number of test replicates and the parameters used, which may be affected by unidentified confounding factors.
Finally, our investigation is limited to in-vitro experiments, lacking of information on the transfusion outcome with Cryo-PLT on patients, such as the determination of the Corrected Count Increment (CCI).Citation87 Although the use of the CCI to assess the efficacy of platelet transfusion is controversial,Citation88,Citation89 we acknowledge that the persistence of platelets after transfusion is a prerequisite for their functionality.Citation20,Citation21 The CCI was indeed used as primary outcome to evaluate the efficacy of prophylactic transfusion with buffy coat derived cryo-PLT in three hematological patientsCitation90 and in a small cohort of women with gynecological malignancies treated with autologous cryo-PLT.Citation91 While existing in vivo studies have demonstrated the hemostatic effectiveness of Cryo-PLT without evidence of an increase in post-transfusion thrombosis,Citation25,Citation92,Citation93 and clinical trials have been conducted to assess the safety and efficacy of Cryo-PLT in different clinical settings,Citation46,Citation47,Citation50 we recognize the need for further in vivo studies or clinical trials to determine the clinical relevance of prophylactic Cryo-PLT transfusion in non-actively bleeding thrombocytopenic patients
List of Abbreviation | ||
1-3-6h | = | Thawed samples at 1-3-6h after reconstitution |
A | = | Thrombin Activation |
A20 | = | Amplitude of clot firmness at 20 min after CT |
ACD-A | = | Acid Citrate Dextrose Solution A |
CCI | = | Corrected Count Increment |
CFT | = | Clot Formation Time |
Cryo-PLT | = | Cryopreserved Platelet |
CT | = | Clotting Time |
CTRL - | = | Negative Control |
CTRL + | = | Positive Control |
DMEM | = | Dulbecco’s Modified Eagle’s Medium |
DMSO | = | Dimethyl Sulfoxide |
EDTA | = | Ethylenediaminetetraacetic Acid |
EV | = | Extracellular Vesicles |
FBS | = | Fetal Bovine Serum |
FGF | = | Fibroblast Growth Factor |
Fresh-PLT | = | Fresh Platelet |
FTIR | = | Fourier Transform Infrared |
GF | = | Grow Factor |
HLA | = | Human Leukocyte Antigen |
HPA | = | Human Platelet Antigen |
MCF | = | Maximum Clot Formation |
MCT | = | Mercury Cadmium Telluride |
NA | = | No thrombin activation |
NK-cell | = | Natural Killer cell |
PBS | = | Phosphate Buffer Saline |
PC | = | Principal Component |
PCA | = | Principal Component Analysis |
PCA | = | Principal Component Analysis |
PDGF-ββ | = | Platelet Derived Growth Factor |
Pen-Strep | = | Penicillin-Streptomycin |
PFA | = | Paraformaldehyde |
PLT | = | Platelet |
PS | = | Phosphatidylserine |
PSL | = | Platelet Storage Lesion |
RANTES | = | Regulated on Activation, Normal T cell Expressed and Secreted |
RPMI | = | Roswell Park Memorial Institute Medium |
RT | = | Room Temperature |
SI | = | Supplemental Information |
SVN | = | Standard Vector Normalization |
TGF-β | = | Tumor Growth Factor |
T-PAS | = | Platelet Addition Solution |
VWF | = | Von Willebrand Factor |
Authors contribution
LM conceived and supervised the study. GG and AR performed experiments, analyzed the data and wrote the initial draft of the manuscript; DS and CM supervised experimental plan. EDB revised the manuscript and clinical support; BI and PB performed cryopreservation; EQ prepared protocol for Review Board approval; DB, LV, and GB performed FTIR experiments and analyzed the data; RB supervised and funded the study. All authors took part in the revision and editing of the final version of the manuscript, and have read and agreed to the final version.
Supplemental Material
Download PDF (791.5 KB)Acknowledgments
Authors wish to thank the personnel at the “Casa del Dono Reggio Emilia” donor center, who helped enroll platelet donors for conducting this study. D.E.B thanks the European Union’s Horizon Europe Marie Sklodowska-Curie grant (Grant agreement No. 101106307).
Disclosure statement
No potential conflict of interest was reported by the author(s).
Data availability statement
The data that support the findings of this study are available from the corresponding authors, DS and CM, upon reasonable request.
Supplementary material
Supplemental data for this article can be accessed online at https://doi.org/10.1080/09537104.2023.2281943.
Additional information
Funding
References
- Smock KJ, Perkins SL. Thrombocytopenia: an update. Int J Lab Hematol. 2014;36:269–14. doi:10.1111/ijlh.12214.
- Humbrecht C, Kientz D, Gachet C. Platelet transfusion: current challenges. Transfus Clin Biol. 2018;25:151–64. doi:10.1016/j.tracli.2018.06.004.
- Estcourt LJ. Why has demand for platelet components increased? a review. Transfus Med. 2014;24(5):260–8. doi:10.1111/tme.12155.
- Schiffer CA, Bohlke K, Delaney M, Hume H, Magdalinski AJ, McCullough JJ, Omel JL, Rainey JM, Rebulla P, Rowley SD, et al. Platelet transfusion for patients with cancer: American society of clinical oncology clinical practice guideline update. J Clin Oncol. 2018;36:283–99. doi:10.1200/jco.2017.76.1734.
- Kogler VJ, Stolla M. There and back again: the once and current developments in donor-derived platelet products for hemostatic therapy. Blood. 2022;139:3688–98. doi:10.1182/blood.2021014889.
- Schiroli D, Merolle L, Molinari G, Di Bartolomeo E, Seligardi D, Canovi L, Pertinhez TA, Mancuso P, Giorgi Rossi P, Baricchi R, et al. The impact of COVID-19 outbreak on the transfusion medicine unit of a Northern Italy hospital and cancer centre. Vox Sang. 2022;117:235–42. doi:10.1111/vox.13174.
- European Directorate for the Quality of Medicines. HealthCare. Guide to the preparation, use and quality assurance of blood components. Recommendation No R(95). 21st ed. Strasbourg (France): Council of Europe; 2023.
- Noorman F, Rijnhout TW, de Kort B, Hoencamp R. Frozen for combat: quality of deep‐frozen thrombocytes, produced and used by the Netherlands armed forces 2001–2021. Transfusion. 2023;63(1):203–16. doi:10.1111/trf.17166.
- Valeri CR, Srey R, Lane JP, Ragno G. Effect of WBC reduction and storage temperature on PLTs frozen with 6 percent DMSO for as long as 3 years. Transfusion. 2003;43:1162–7. doi:10.1046/j.1537-2995.2003.00468.x.
- Handin RI, Valeri CR. Improved viability of previously frozen platelets. Blood. 1972;40(4):509–13. doi:10.1182/blood.V40.4.509.509.
- Johnson L, Vekariya S, Tan S, Padula MP, Marks DC. Extended storage of thawed platelets: refrigeration supports postthaw quality for 10 days. Transfusion. 2020;60:2969–81. doi:10.1111/trf.16127.
- Rinder HM, Snyder EL. Activation of platelet concentrate during preparation and storage. Blood Cells. 1992;18:445–56. discussion 57-60.
- Krailadsiri P, Seghatchian J, Williamson LM. Platelet storage lesion of WBC‐reduced, pooled, buffy coat‐derived platelet concentrates prepared in three in‐process filter/storage bag combinations. Transfusion. 2001;41(2):243–50. doi:10.1046/j.1537-2995.2001.41020243.x.
- Das S, Kumar MH. Comparative analysis of platelet storage lesion in whole-blood-derived platelets stored in autologous plasma versus platelets stored in platelet additive solution using flow cytometric assay of CD62 and Annexin V. Glob J Transfus Med. 2019;4:21–7. doi:10.4103/GJTM.GJTM_24_19.
- Ng MSY, Tung JP, Fraser JF. Platelet storage lesions: what more do we know now? Transfus Med Rev. 2018;32:144–54. doi:10.1016/j.tmrv.2018.04.001.
- Paglia G, Sigurjónsson ÓE, Rolfsson Ó, Valgeirsdottir S, Hansen MB, Brynjólfsson S, Gudmundsson S, Palsson BO. Comprehensive metabolomic study of platelets reveals the expression of discrete metabolic phenotypes during storage. Transfusion. 2014;54:2911–23. doi:10.1111/trf.12710.
- Vit G, Klüter H, Wuchter P. Platelet storage and functional integrity. J Lab Med. 2020;44(5):285–93. doi:10.1515/labmed-2020-0067.
- Rinder HM, Murphy M, Mitchell JG, Stocks J, Ault KA, Hillman RS. Progressive platelet activation with storage: evidence for shortened survival of activated platelets after transfusion. Transfusion. 1991;31:409–14. doi:10.1046/j.1537-2995.1991.31591263195.x.
- Valeri CR, MacGregor H, Giorgio A, Ragno G. Circulation and hemostatic function of autologous fresh, liquid-preserved, and cryopreserved baboon platelets transfused to correct an aspirin-induced thrombocytopathy. Transfusion. 2002;42:1206–16. doi:10.1046/j.1537-2995.2002.00195.x.
- Larsen JB, Hojbjerg JA, Hvas AM. The role of platelets in cancer-related bleeding risk: a systematic review. Semin Thromb Hemost. 2020;46:328–41. doi:10.1055/s-0039-3402429.
- Akkök CA, Brinch L, Lauritzsen GF, Solheim BG, Kjeldsen-Kragh J. Clinical effect of buffy-coat vs. apheresis platelet concentrates in patients with severe thrombocytopenia after intensive chemotherapy. Vox Sang. 2007;93:42–8. doi:10.1111/j.1423-0410.2007.00917.x.
- Jimenez-Marco T, Castrillo A, Hierro-Riu F, Vicente V, Rivera J. Frozen and cold-stored platelets: reconsidered platelet products. Platelets. 2022;33:27–34. doi:10.1080/09537104.2021.1967917.
- Dumont LJ, Slichter SJ, Reade MC. Cryopreserved platelets: frozen in a logjam? Transfusion. 2014;54(8):1907–10. doi:10.1111/trf.12758.
- Rajashekaraiah V, Rajanand MC. Platelet storage: progress so far. J Thromb Thrombolysis. 2023;55:9–17. doi:10.1007/s11239-022-02716-3.
- Gerber B, Alberio L, Rochat S, Stenner F, Manz MG, Buser A, Schanz U, Stussi G. Safety and efficacy of cryopreserved autologous platelet concentrates in HLA-alloimmunized patients with hematologic malignancies. Transfusion. 2016;56:2426–37. doi:10.1111/trf.13690.
- Slichter SJ, Jones M, Ransom J, Gettinger I, Jones MK, Christoffel T, Pellham E, Bailey SL, Corson J, Bolgiano D, et al. Review of in vivo studies of dimethyl sulfoxide cryopreserved platelets. Transfus Med Rev. 2014;28:212–25. doi:10.1016/j.tmrv.2014.09.001.
- Johnson L, Tan S, Jenkins E, Wood B, Marks DC. Characterization of biologic response modifiers in the supernatant of conventional, refrigerated, and cryopreserved platelets. Transfusion. 2018;58:927–37. doi:10.1111/trf.14475.
- Pu F, Li X, Wang S, Huang Y, Wang D. Platelet supernatant with longer storage inhibits tumor cell growth. Transfus Apher Sci. 2021;60:103042. doi:10.1016/j.transci.2020.103042.
- Palacios-Acedo AL, Mège D, Crescence L, Dignat-George F, Dubois C, Panicot-Dubois L. Platelets, thrombo-inflammation, and cancer: collaborating with the enemy. Front Immunol. 2019;10:1805. doi:10.3389/fimmu.2019.01805.
- Kelly K, Cancelas JA, Szczepiorkowski ZM, Dumont DF, Rugg N, Dumont LJ. Frozen platelets-development and future directions. Transfus Med Rev. 2020;34:286–93. doi:10.1016/j.tmrv.2020.09.008.
- Johnson L, Lei P, Waters L, Padula MP, Marks DC. Identification of platelet subpopulations in cryopreserved platelet components using multi-colour imaging flow cytometry. Sci Rep. 2023;13:1221. doi:10.1038/s41598-023-28352-2.
- Johnson LN, Winter KM, Reid S, Hartkopf-Theis T, Marks DC. Cryopreservation of buffy-coat-derived platelet concentrates in dimethyl sulfoxide and platelet additive solution. Cryobiology. 2011;62:100–6. doi:10.1016/j.cryobiol.2011.01.003.
- Winskel-Wood B, Padula MP, Marks DC, Johnson L. The phenotype of cryopreserved platelets influences the formation of platelet-leukocyte aggregates in an in vitro model. Platelets. 2023;34:2206916. doi:10.1080/09537104.2023.2206916.
- Liumbruno G, Bennardello F, Lattanzio A, Piccoli P, Rossetti G. Recommendations for the transfusion of plasma and platelets. Blood Transfus. 2009;7:132–50. doi:10.2450/2009.0005-09.
- Valeri CR, Ragno G, Khuri S. Freezing human platelets with 6 percent dimethyl sulfoxide with removal of the supernatant solution before freezing and storage at -80 degrees C without postthaw processing. Transfusion. 2005;45:1890–8. doi:10.1111/j.1537-2995.2005.00647.x.
- Marks DC, Johnson L. Assays for phenotypic and functional characterization of cryopreserved platelets. Platelets. 2019;30(1):48–55. doi:10.1080/09537104.2018.1514108.
- Marchisio M, Simeone P, Bologna G, Ercolino E, Pierdomenico L, Pieragostino D, Ventrella A, Antonini F, Del Zotto G, Vergara D, et al. Flow cytometry analysis of circulating extracellular vesicle subtypes from fresh peripheral blood samples. Int J Mol Sci. 2020;22:48. doi:10.3390/ijms22010048.
- Birarda G, Bedolla D, Piccirilli F, Stani C, Vondracek H, Vaccari L. Chemical analyses at micro and nano scale at SISSI-Bio beamline at elettra-sincrotrone trieste. In: Biomedical vibrational spectroscopy 2022: advances in research and industry. SPIE; 2022. p. 27–39. doi:10.1117/12.2607751.
- Toplak M, Read ST, Sandt C, Borondics F. Quasar: easy machine learning for Biospectroscopy. Cells. 2021;10:2300. doi:10.3390/cells10092300.
- XX Z, Yang Y, Zhang Y, Zhang ZG, XF W, Shi YG. Platelets promote breast cancer cell MCF-7 metastasis by direct interaction: surface integrin α2β1-contacting-mediated activation of Wnt-β-catenin pathway. Cell Commun Signal. 2019;17:142. doi:10.1186/s12964-019-0464-x.
- Comşa Ş, Cimpean AM, Raica M. The story of MCF-7 breast cancer cell line: 40 years of experience in research. Anticancer Res. 2015;35:3147–54.
- Schneider CA, Rasband WS, Eliceiri KW. NIH image to ImageJ: 25 years of image analysis. Nat Methods. 2012;9:671–5. doi:10.1038/nmeth.2089.
- Shou LM, Zhang QY, Li W, Xie X, Chen K, Lian L, Li Z-Y, Gong F-R, Dai K-S, Mao Y-X, et al. Cantharidin and norcantharidin inhibit the ability of MCF-7 cells to adhere to platelets via protein kinase C pathway-dependent downregulation of α2 integrin. Oncol Rep. 2013;30:1059–66. doi:10.3892/or.2013.2601.
- Pachetti M, Zupin L, Venturin I, Mitri E, Boscolo R, D’Amico F, Vaccari L, Crovella S, Ricci G, Pascolo L, et al. FTIR spectroscopy to reveal lipid and protein changes induced on sperm by capacitation: bases for an improvement of sample selection in ART. Int J Mol Sci. 2020;21(22):8659. doi:10.3390/ijms21228659.
- Baker MJ, Trevisan J, Bassan P, Bhargava R, Butler HJ, Dorling KM, Fielden PR, Fogarty SW, Fullwood NJ, Heys KA, et al. Using Fourier transform IR spectroscopy to analyze biological materials. Nat Protoc. 2014;9(8):1771–91. doi:10.1038/nprot.2014.110.
- Reade MC, Marks DC, Bellomo R, Deans R, Faulke DJ, Fraser JF, Gattas DJ, Holley AD, Irving DO, Johnson L, et al. A randomized, controlled pilot clinical trial of cryopreserved platelets for perioperative surgical bleeding: the CLIP-I trial (Editorial, p. 2759). Transfusion. 2019;59:2794–804. doi:10.1111/trf.15423.
- McGuinness S, Charlewood R, Gilder E, Parke R, Hayes K, Morley S, Al‐Ibousi A, Deans R, Howe B, Johnson L, et al. A pilot randomized clinical trial of cryopreserved versus liquid-stored platelet transfusion for bleeding in cardiac surgery: the cryopreserved versus liquid platelet-New Zealand pilot trial. Vox Sang. 2022;117:337–45. doi:10.1111/vox.13203.
- Ward MP, EK L, AN L, Mohamed BM, Kelly T, Bates M, Clarke A, Brady N, Martin CM, Brooks RD, et al. Platelets, immune cells and the coagulation cascade; friend or foe of the circulating tumour cell? Mol Cancer. 2021;20:59. doi:10.1186/s12943-021-01347-1.
- Miao S, Shu D, Zhu Y, Lu M, Zhang Q, Pei Y, He A-D, Ma R, Zhang B, Ming Z-Y, et al. Cancer cell-derived immunoglobulin G activates platelets by binding to platelet FcγRIIa. Cell Death Dis. 2019;10(2):87. doi:10.1038/s41419-019-1367-x.
- Slichter SJ, Dumont LJ, Cancelas JA, Jones M, Gernsheimer TB, Szczepiorkowski ZM, Dunbar NM, Prakash G, Medlin S, Rugg N, et al. Safety and efficacy of cryopreserved platelets in bleeding patients with thrombocytopenia. Transfusion. 2018;58(9):2129–38. doi:10.1111/trf.14780.
- Lang T, Bauters A, Braun SL, Pötzsch B, von Pape K-W, Kolde H-J, Lakner M. Multi-centre investigation on reference ranges for ROTEM thromboelastometry. Blood Coagul Fibrinolysis. 2005;16:301–10. doi:10.1097/01.mbc.0000169225.31173.19.
- Johnson L, Reade MC, Hyland RA, Tan S, Marks DC. In vitro comparison of cryopreserved and liquid platelets: potential clinical implications. Transfusion. 2015;55:838–47. doi:10.1111/trf.12915.
- Cid J, Escolar G, Galan A, López‐Vilchez I, Molina P, Díaz‐Ricart M, Lozano M, Dumont LJ. In vitro evaluation of the hemostatic effectiveness of cryopreserved platelets. Transfusion. 2016;56:580–6. doi:10.1111/trf.13371.
- Tynngård N, Wikman A, Uhlin M, Sandgren P. Haemostatic responsiveness and release of biological response modifiers following cryopreservation of platelets treated with amotosalen and ultraviolet a light. Blood Transfus. 2020;18:191–9. doi:10.2450/2019.0061-19.
- Meinke S, Wikman A, Gryfelt G, Hultenby K, Uhlin M, Höglund P, Sandgren P. Cryopreservation of buffy coat–derived platelet concentrates photochemically treated with amotosalen and UVA light. Transfusion. 2018;58:2657–68. doi:10.1111/trf.14905.
- Johnson L, Coorey CP, Marks DC. The hemostatic activity of cryopreserved platelets is mediated by phosphatidylserine‐expressing platelets and platelet microparticles. Transfusion. 2014;54(8):1917–26. doi:10.1111/trf.12578.
- Six KR, Delabie W, Devreese KM, Johnson L, Marks DC, Dumont LJ, Compernolle V, Feys HB. Comparison between manufacturing sites shows differential adhesion, activation, and GPIbα expression of cryopreserved platelets. Transfusion. 2018;58:2645–56. doi:10.1111/trf.14828.
- Nagler M, Kathriner S, Bachmann LM, Wuillemin WA. Impact of changes in haematocrit level and platelet count on thromboelastometry parameters. Thromb Res. 2013;131(3):249–53. doi:10.1016/j.thromres.2013.01.009.
- Leyra F, Jofre C, Peña N, Olmos E, Del Campo JM, Aranzubia M, Moreno I. Prediction of platelet counts with ROTEM-sigma in cardiac surgery. Minerva Anestesiol. 2022;88:573–9. doi:10.23736/S0375-9393.22.15912-2.
- Noorman F, Hess JR. The contribution of the individual blood elements to the variability of thromboelastographic measures. Transfusion. 2018;58(10):2430–6. doi:10.1111/trf.14884.
- Waters L, Padula MP, Marks DC, Johnson L. Cryopreserved platelets demonstrate reduced activation responses and impaired signaling after agonist stimulation. Transfusion. 2017;57:2845–57. doi:10.1111/trf.14310.
- Barnard MR, MacGregor H, Ragno G, Pivacek LE, Khuri SF, Michelson AD, Valeri CR. Fresh, liquid-preserved, and cryopreserved platelets: adhesive surface receptors and membrane procoagulant activity. Transfusion. 1999;39:880–8. doi:10.1046/j.1537-2995.1999.39080880.x.
- Springer TA. von Willebrand factor, Jedi knight of the bloodstream. Blood. 2014;124:1412–25. doi:10.1182/blood-2014-05-378638.
- Six KR, Compernolle V, Feys HB. Platelet biochemistry and morphology after cryopreservation. Int J Mol Sci. 2020;21:935. doi:10.3390/ijms21030935.
- Johnson L, Tan S, Wood B, Davis A, Marks DC. Refrigeration and cryopreservation of platelets differentially affect platelet metabolism and function: a comparison with conventional platelet storage conditions. Transfusion. 2016;56:1807–18. doi:10.1111/trf.13630.
- Gläfke C, Akhoondi M, Oldenhof H, Sieme H, Wolkers WF. Cryopreservation of platelets using trehalose: the role of membrane phase behavior during freezing. Biotechnol Prog. 2012;28(5):1347–54. doi:10.1002/btpr.1600.
- Reid TJ, LaRussa VF, Esteban G, Clear M, Davies L, Shea S, Gorogias M. Cooling and freezing damage platelet membrane integrity. Cryobiology. 1999;38:209–24. doi:10.1006/cryo.1999.2164.
- Kumar S, Verma T, Mukherjee R, Ariese F, Somasundaram K, Umapathy S. Raman and infra-red microspectroscopy: towards quantitative evaluation for clinical research by ratiometric analysis. Chem Soc Rev. 2016;45(7):1879–900. doi:10.1039/C5CS00540J.
- Eker İ, Yılmaz S, Çetinkaya RA, Pekel A, Ünlü A, Gürsel O, Yılmaz S, Avcu F, Muşabak U, Pekoğlu A, et al. Generation of platelet microparticles after cryopreservation of apheresis platelet concentrates contributes to hemostatic activity. Turk J Haematol. 2017;34:64. doi:10.4274/tjh.2016.0049.
- Reddy EC, Rand ML. Procoagulant phosphatidylserine-exposing platelets in vitro and in vivo. Front Cardiovasc Med. 2020;7:15. doi:10.3389/fcvm.2020.00015.
- Josefsson EC, Ramström S, Thaler J, Lordkipanidzé M. Consensus report on markers to distinguish procoagulant platelets from apoptotic platelets: communication from the scientific and standardization committee of the ISTH. J Thromb Haemost. 2023;21:2291–9. doi:10.1016/j.jtha.2023.05.001.
- Żmigrodzka M, Guzera M, Miśkiewicz A, Jagielski D, Winnicka A. The biology of extracellular vesicles with focus on platelet microparticles and their role in cancer development and progression. Tumour Biol. 2016;37:14391–401. doi:10.1007/s13277-016-5358-6.
- Lazar S, Wurtzel JGT, Chen X, Ma P, Goldfinger LE. High-efficiency unassisted transfection of platelets with naked double-stranded miRnas modulates signal-activated translation and platelet function. Platelets. 2021;32:794–806. doi:10.1080/09537104.2020.1809642.
- Tegegn TZ, De Paoli SH, Orecna M, Elhelu OK, Woodle SA, Tarandovskiy ID, Ovanesov MV, Simak J. Characterization of procoagulant extracellular vesicles and platelet membrane disintegration in DMSO-cryopreserved platelets. J Extracellular Vesicle. 2016;5:30422. doi:10.3402/jev.v5.30422.
- Boilard E, Bellio M. Platelet extracellular vesicles and the secretory interactome join forces in health and disease. Immunol Rev. 2022;312:38–51. doi:10.1111/imr.13119.
- Sinauridze EI, Kireev DA, Popenko NY, Pichugin A, Panteleev M, Krymskaya O, Ataullakhanov F. Platelet microparticle membranes have 50- to 100-fold higher specific procoagulant activity than activated platelets. Thromb Haemost. 2007;97:425–34. doi:10.1160/TH06-06-0313.
- Berckmans RJ, Lacroix R, Hau CM, Sturk A, Nieuwland R. Extracellular vesicles and coagulation in blood from healthy humans revisited. J Extracell Vesicles. 2019;8:1688936. doi:10.1080/20013078.2019.1688936.
- Puhm F, Boilard E, Machlus KR. Platelet extracellular vesicles: beyond the blood. Arterioscler Thromb Vasc Biol. 2021;41:87–96. doi:10.1161/ATVBAHA.120.314644.
- Cardigan R, Sutherland J, Wadhwa M, Dilger P, Thorpe R. The influence of platelet additive solutions on cytokine levels and complement activation in platelet concentrates during storage. Vox Sang. 2003;84(1):28–35. doi:10.1046/j.1423-0410.2003.00257.x.
- Pulcini S, Merolle L, Marraccini C, Quartieri E, Mori D, Schiroli D, Berni P, Iotti B, Di Bartolomeo E, Baricchi R, et al. Apheresis platelet rich-plasma for regenerative medicine: an in vitro study on osteogenic potential. Int J Mol Sci. 2021;22:8764. doi:10.3390/ijms22168764.
- Katoh M, Nakagama H. FGF receptors: cancer biology and therapeutics. Med Res Rev. 2014;34:280–300. doi:10.1002/med.21288.
- Pietras K, Sjöblom T, Rubin K, Heldin CH, Ostman A. PDGF receptors as cancer drug targets. Cancer Cell. 2003;3:439–43. doi:10.1016/s1535-6108(03)00089-8.
- Morikawa M, Derynck R, Miyazono K. TGF-β and the TGF-β family: context-dependent roles in cell and tissue physiology. Cold Spring Harb Perspect Biol. 2016;8:a021873. doi:10.1101/cshperspect.a021873.
- Koupenova M, Clancy L, Corkrey HA, Freedman JE. Circulating platelets as mediators of immunity, inflammation, and thrombosis. Circ Res. 2018;122:337–51. doi:10.1161/circresaha.117.310795.
- Labelle M, Begum S, Hynes RO. Direct signaling between platelets and cancer cells induces an epithelial-mesenchymal-like transition and promotes metastasis. Cancer Cell. 2011;20:576–90. doi:10.1016/j.ccr.2011.09.009.
- He AD, Xie W, Song W, Ma Y-Y, Liu G, Liang M-L, Da X-W, Yao G-Q, Zhang B-X, Gao C-J, et al. Platelet releasates promote the proliferation of hepatocellular carcinoma cells by suppressing the expression of KLF6. Sci Rep. 2017;7:3989. doi:10.1038/s41598-017-02801-1.
- Jaime-Pérez JC, Vázquez-Hernández KE, Jiménez-Castillo RA, Fernández LT, Salazar-Riojas R, Gómez-Almaguer D. Platelet survival in hematology patients assessed by the corrected count increment and other formulas. Am J Clin Pathol. 2018;150:267–72. doi:10.1093/ajcp/aqy052.
- Apelseth TO, Bruserud O, Wentzel-Larsen T, Hervig T. Therapeutic efficacy of platelet transfusion in patients with acute leukemia: an evaluation of methods. Transfusion. 2010;50:766–75. doi:10.1111/j.1537-2995.2009.02540.x.
- Davis KB, Slichter SJ, Corash L. Corrected count increment and percent platelet recovery as measures of posttransfusion platelet response: problems and a solution. Transfusion. 1999;39:586–92. doi:10.1046/j.1537-2995.1999.39060586.x.
- Napolitano M, Mancuso S, Lo Coco L, Arfò PS, Raso S, De Francisci G, Dieli F, Caccamo N, Reinaf A, Dolceg A, et al. Buffy coat-derived platelets cryopreserved using a new method: results from in vitro studies. Transfus Apher Sci. 2018;57(4):578–81. doi:10.1016/j.transci.2018.07.020.
- Vadhan-Raj S, Kavanagh JJ, Freedman RS, Folloder J, Currie LM, Bueso-Ramos C, Verschraegen CF, Narvios AB, Connor J, Hoots WK, et al. Safety and efficacy of transfusions of autologous cryopreserved platelets derived from recombinant human thrombopoietin to support chemotherapy-associated severe thrombocytopenia: a randomised cross-over study. Lancet. 2002;359(9324):2145–52. doi:10.1016/S0140-6736(02)09090-6.
- Noorman F, van Dongen TT, Plat MJ, Badloe JF, Hess JR, Hoencamp R, Dao M. Transfusion: -80°C frozen blood products are safe and effective in military casualty care. PloS One. 2016;11:e0168401. doi:10.1371/journal.pone.0168401.
- Khuri SF, Healey N, MacGregor H, Barnard MR, Szymanski IO, Birjiniuk V, Michelson AD, Gagnon DR, Valeri CR. Comparison of the effects of transfusions of cryopreserved and liquid-preserved platelets on hemostasis and blood loss after cardiopulmonary bypass. J Thorac Cardiovasc Surg. 1999;117:172–83. discussion 83-4. doi:10.1016/s0022-5223(99)70483-6.