Abstract
A surface plasmon resonance biosensor method was developed to measure zilpaterol residues in sheep urine. A CM-5 sensor chip previously reacted with ethylenediamine to produce an aminoethyl group was coupled with 4-carboxybutyl zilpaterol activated using EDC/NHS. Five polyclonal and four monoclonal antibodies were screened for their suitability to detect low levels of zilpaterol using the biosensor technology. Total binding was greater for polyclonal than monoclonal antibodies, but a less diluted antibody solution was required for polyclonal antibodies. A fixed antibody concentration and various concentrations of zilpaterol were injected to obtain a standard curve for each antibody to allow for B0 and IC50 determination. The stability of the assay was assessed by the consistency of B0 in repeated experiments extending at least six hours. A measure of non-specific binding allowed the assessment of the specificity of the antibody-immobilized ligand interaction. The effect of varying concentrations of urine on B0 and IC50 was evaluated to assess the degree of “matrix” effect that would be present in an assay. Based on these criteria the most promising antibody (2E10, a monoclonal antibody) was selected for further evaluation. This antibody had good sensitivity with IC50=4.47±0.41 ng/ml (n=11) in buffer). Both intra- and inter-assay variation studies showed excellent recovery and reproducibility for concentrations between 2 ng/ml and 8 ng/ml. A comparison of the biosensor method with a previously developed ELISA demonstrated that both methods give equivalent results (slope of the correlation plot = 1.02) with a high correlation (r2=0.91) between them.
*The use of trade, firm, or corporation names in this publication is for the information and convenience of the reader. Such use does not constitute an official endorsement or approval by the United States Department of Agriculture or the Agricultural Research Service of any product or service to the exclusion of others that may be suitable.
Introduction
Zilpaterol is a β-adrenergic agonist approved as a feed additive to improve cattle growth in Mexico and South Africa. Currently the use of zilpaterol has not been approved in the United States or the European Union (EU), hence a zero tolerance policy for this drug is followed in these countries. Zilpaterol improves feeding efficiency, decreases feed consumption, and produces leaner meat (Plascencia et al. Citation1999, Salinas-Chavira et al. Citation2004), offering an economic advantage that could lead to usage of zilpaterol in species for which no approval exists. Mitchell and Dunnavan (Citation1998) have reported that β-adrenergic agonists have been used illicitly within the US and Kuiper et al. (Citation1998) reported the illegal usage of beta-agonists in the EU. Because of a potential for illegal zilpaterol use, and because the US and the EU have not approved the use of zilpaterol in farm animals, there is a need for rapid detection methods of animals exposed to zilpaterol.
Current zilpaterol analysis requires expensive instrumentation and extensive sample clean-up. A GC-MS method has been reported to determine zilpaterol in feed and tissues (Bocca et al. Citation2003a Citationb). The determination levels for these samples were in the range of high ppb and require a derivatization step. LC-tandem-MS methods for zilpaterol analysis have also been reported for urine, feces, and tissue sample determination (Stachel et al. Citation2003, Van Hoof et al. Citation2005). This method was able to measure zilpaterol at the sub ppb level, but required multiple extractions (liquid-liquid partition and solid phase extraction).
Immunoassays provide the advantages of high throughput, rapid turnaround time, user friendliness, and field portability in some cases. The usefulness of the immunoassay has been demonstrated by extensive assay development for β-adrenergic agonists (Yamamoto and Twata Citation1982, Adam et al. Citation1990, Haasnoot et al. Citation1994, Elliott et al. Citation1998b, Shelver and Smith Citation2000 Citation2004, Shelver et al. Citation2000). Surface plasmon resonance (SPR) optical biosensor technology (Karlsson et al. Citation1991), a relatively new form of immunoassay, has been used by a number of investigators for residue analyses. Briefly, SPR utilizes the change in the angle of reflection of a reduced amount of light, produced by a change in the refractive index of the media adjacent to a gold surface. This change is produced by the binding of an antibody to an immobilized ligand covalently attached to a gold surface, thereby altering the refractive index adjacent to the reflecting surface. Free ligand in solution complexes with the antibody, decreases the amount of antibody interacting with the immobilized ligand, and thus produces a reduction in the angular change of the monitored reflection. The reduction in angular change in reflection is proportional to the ligand concentration and the result is displayed as a sensorgram. This technique has been applied to residue analysis in different classes of compounds and a variety of matrices. Some of the more recent examples are listed as follows: streptomycin in milk (Baxter et al. Citation2001); sulphamethazine in milk (Bergström et al. Citation1999); salbutamol in urine (Elliott et al. Citation1998a); streptomycin and dihydrostreptomycin in honey and meat (Ferguson et al. Citation2002); β-lactams in milk (Gustavsson & Sternesjo Citation2004); clenbuterol in urine (Haughey et al. Citation2001); ivermectin in liver (Samsonova et al. Citation2002); and 4-nonylphenols in shellfish (Samsonova et al. Citation2004). Modern instrumentation allows for automated operation and permits rapid determination of multiple samples. The SPR biosensor technique often shows better tolerance of the matrix effects that create problems for many other analytical systems. In this study, we utilize a Biacore Q SPR biosensor to evaluate nine zilpaterol antibodies. One of these antibodies was selected for development of a biosensor assay capable of detecting zilpaterol residues in ovine urine samples.
Materials and methods
Materials and instrumentation
Zilpaterol.HCl was a gift from Houchest-Russell, Clinton, NJ. Sensor chips (CM5) and HBS-EP buffer were purchased from Biacore Inc. (Uppsala, Sweden) and stored at 4°C until used. Ethanolamine, bovine serum albumin, bovine thyroglobulin, butandiol diglycidyl ether, complete Freund's adjuvant and incomplete Freund's adjuvant, dimethylaminopyridine, dimethyl sulphoxide, disuccinimidyl carbonate, ethylenediamine, human serum albumin, Tween 20, N-hydroxysuccinimide (NHS), and N-ethyl-N′-(3-dimethylaminopropyl)-carbodiimide (EDC) were purchased from Sigma-Aldrich (St Louis, MO, USA or Dorset, UK). A Biacore Q (Biacore AB) incorporated with control software was utilized for zilpaterol antibody evaluation and zilpaterol concentration determination via SPR.
Buffers
HBS-EP containing 10 mM HEPES pH 7.4, 150 mM NaCl, 3 mM EDTA, and 0.005% Surfactant P20 was used as the running buffer. HBS-EP-BSA containing 100 mg BSA per 100 ml HBS-EP was used to dilute antibodies and zilpaterol for B0 and IC50 determination. Phosphate buffer containing 10 mM phosphate (pH 7.4), 0.05% Tween 20, and 1 M NaCl was used to dilute incurred zilpaterol urine sample for ELISA and biosensor analysis.
Zilpaterol sensor chip preparation
CM-5 sensor chips were placed individually in a humidity chamber and reactions were performed at room temperature. The chip surface was wetted with 50 µl of HBS-EP and incubated at room temperature for 5 min, after which, the HPS-EP was removed. The chip surface was activated using 50 µl of a freshly prepared solution containing 200 mM EDC and 50 mM NHS at room temperature for 20 min. After removing the EDC/NHS solution, 50 µl of 1 M ethylenediamine was added to the chip surface and allowed to react for 20 min. The ethylenediamine solution was removed by blotting with tissue paper, rinsing with water which was then removed by blotting, and finally the surface was dried with N2. An activated zilpaterol solution was prepared by adding 2 mg of 4-carboxybutyl zilpaterol to a solution containing 5 mg of EDC and 2 mg of NHS in 950 µl of 10 mM sodium acetate, pH 4.5. After mixing, the pH was adjusted to 6 and 50 µl of the solution was added to the well. The reaction was allowed to run at room temperature overnight. The excess reactant solution was removed and the surface washed twice with water, dried with a stream of N2 and stored in a desiccator at 4°C until used.
Zilpaterol antibody generation
The immunogen for rabbit antisera R674 was prepared by coupling zilpaterol activated with disuccinimidyl carbonate in DMSO and dimethylaminopyrimidine with cationized human serum albumin followed by dialyzing the conjugate against saline. The rabbit was immunized every two weeks with 0.1 mg of immunogen initially emulsified with complete Freund's adjuvant and subsequently emulsified with incomplete adjuvant for a total of nine immunizations. The immunogen for rabbit antisera R698 was prepared using bovine thyroglobulin activated with butandiol diglycidylether and sodium borohydride after which the product was reacted with zilpaterol. The rabbit was immunized every two weeks with 1 mg of immunogen initially emulsified with complete Freund's adjuvant and subsequently emulsified with incomplete adjuvant for a total of 10 immunizations. The immunogen for rabbit antisera R699 was prepared similarly except human serum albumin was used in place of bovine thyroglobulin. A total of two immunizations produced adequate titer. Monoclonal antibody F140 was produced by immunizing a BALB/c mouse with a clenbuterol transferrin conjugate (Fleeker and Lovett Citation1985). The mouse's splenocytes were fused with a mouse myeloma, and the hybridomas were screened for antibodies to clenbuterol. Monoclonal antibody F140 demonstrated activity towards a variety of beta agonists and for this reason was selected for this study. Zilpaterol monoclonal antibodies 2E10, 3H5, and 7A8 were generated by the fusion of 4-carboxybutyl zilpaterol sensitized mouse splenocytes with fusion partner Sp2/0.Ag14 (Shelver et al. Citation2005). Generation of zilpaterol goat antibodies #133 and #134 were as reported except 9th boost sera were used for this study (Shelver and Smith Citation2004).
Maximum binding capacity determination
Zilpaterol antibodies (1:10 in HBS-EP) were injected over the zilpaterol sensor flow cell at 5 µl/min for 10 min and the resultant instrument responses were measured. These responses were based on the difference in the measured signal 5 sec before and 30 sec after the injection of the diluted antibodies. Sensor chips were regenerated by injecting 0.1 N NaOH at a flow rate of 20 µl/min for 1.5 min.
Antibody working dilution determination
To determine the working dilution for each antibody, the responses to a series of 1:2 dilutions of the antibodies were determined. Initial dilutions of 1:10 and 1:100 were selected for the polyclonal and monoclonal antibodies respectively since these produced saturation responses. With increasing dilution, the response became a linear function of the dilution. The dilutions that produced Response Units (RU) in the range of 250–500 were selected as the working dilutions to determine IC50.
Sensitivity optimization
The 2E10 clone was selected to optimize the assay conditions. Assay development variables such as flow rate (10–40 µl/min), contact time (1–8 min), and ratio of antibody to analyte (20–80%) were used to find the optimal assay conditions for urine sample analysis.
Urine samples
Urine samples containing zilpaterol residues were collected from sheep fed a diet containing 0.15 mg/kg/day of zilpaterol for 10 consecutive days. Urine was collected during the feeding and withdrawal periods. Samples were stored at −20°C until analysis. Blank urines were obtained prior to administration of zilpaterol and were pooled for matrix effect determination. Details of the zilpaterol feeding study, sample treatment, and analysis will be published elsewhere (Shelver and Smith, in preparation).
Biosensor analysis for zilpaterol incurred urine samples
A 1:1000 dilution of ascites fluid generated from monoclonal antibody 2E10 was mixed at a 20:80 ratio with a range of zilpaterol standards to produce a standard curve for use in determining zilpaterol concentrations in unknown samples. The solution was passed over the zilpaterol sensor chip at a flow rate of 10 µl/min for 3 min, after which the reaction was stopped and the sensor surface was regenerated by addition of 20 mM NaOH at a flow rate of 10 µl/min for 1.5 min. Concentration of zilpaterol residues in urine samples was determined from a calibration curve generated from zilpaterol in PBST at 0, 1, 2.5, 5, 10 ng/ml. If the value produced by the incurred sample was above the maximum concentration of the calibration curve, the sample was further diluted so that the value was within the standard curve. The dilutions required ranged from 1:5–1:2000. The curve was fitted with a 4 parameter logistic equation using Biacore Control Software. A calibration curve fitted to data obtained over 11 separate runs is shown in .
Matrix effects determination
Zilpaterol standard curves in buffer and in various concentration of sheep urine for different antibodies were generated. The IC50 (mid point inhibition), and B0 (no-competitor), were utilized as criteria to choose antibodies for inter- and intra-assay development. The stability of B0 from the start to finish of overnight runs was an important factor in selecting the antibody since any change in this value would be deleterious to the accuracy of the assay results.
Inter- and intra-assay variation determination
The dilution at which the urine produced a standard curve with no significant difference from the standard curve carried out in the phosphate buffer was selected as the working dilution for the inter- and intra-assay determination for 2E10. Zilpaterol was spiked to final concentrations of 1, 2, 4, 6, and 8 ng/ml in 1:5 sheep urine with phosphate buffer. Inter-assay variation was computed from the analysis of two replicates of each concentration point carried out on five different days. Intra-assay variation was measured by analysis of 12 replicates of each concentration point on a single day. Sample recoveries were extrapolated from a calibration curve with zilpaterol concentrations of 0, 1, 2.5, 5, and 10 ng/ml in sheep urine diluted 1:5 with phosphate buffer.
ELISA measurements for incurred urine samples
The procedures for ELISA measurements of raw urine samples are similar to those previously reported (Shelver et al. 2005). Briefly, ELISA plates were coated with 15 ng/well of 4-carboxybutyl-zilpaterol-BSA for 2 h at 37°C. Samples and zilpaterol standards were dissolved in phosphate buffer and co-incubated with zilpaterol antibody 2E10 (3.1 ng/well) for 90 min at 37°C. After incubation with horseradish peroxidase labeled anti-mouse-IgG (1: 25,000; incubated at 37°C for 1 h), the colour was developed using 3, 3′, 5, 5′-tetramethylbenzidine (TMB) as substrate. After 30 min, the colour development was stopped by the addition of 2 N sulfuric acid and the absorbance read at 450 nm. A zilpaterol calibration curve of 0, 0.1, 0.2, 0.5, 1, 2, 5, 10, 20, 50, 100 and 1000 ng/ml was used to determine the zilpaterol level in the urine samples.
Results and discussion
The purpose of the present study was to develop a reproducible and stable assay for zilpaterol residues using SPR optical biosensor technology. A series of eight antibodies generated toward zilpaterol and one broad-spectrum antibody generated toward clenbuterol were evaluated using multiple screening criteria to select the best candidate for zilpaterol immunoassay development.
Generation of zilpaterol sensor chip
In order to develop a zilpaterol SPR biosensor method a derivative of the analyte must be immobilized on the sensor chip in a reproducible and stable manner and the antibody must bind to the immobilized analyte derivative. The CM-5 sensor chip utilized for assay development incorporates carboxy dextran which contains free carboxyl groups on a thin surface of gold. Several attempts to prepare a derivative of zilpaterol containing a primary amino group for conjugation to the chip surface failed. One route utilized 3-bromopropyl amine to form a propylamine linker. The second route utilized the reaction of zilpaterol with acrylnitrile followed by reduction of the nitrile group to generate the primary amino group desired for coupling. The examination of the products utilizing LC-MS detected none of the desired amino derivatives.
An alternate strategy utilizing a two-step conjugation procedure was employed; first the carboxyl groups on the sensor surface were converted to β-aminoethyl carboxamide groups by reaction of the surface with ethylenediamine; second, 4-carboxybutyl-zilpaterol (Shelver and Smith Citation2004) activated by EDC was added forming the zilpaterol butylamide derivative. This approach attached the zilpaterol to the sensor chip with a long side chain allowing it to freely interact with the anti-zilpaterol antibodies. The surface proved to be highly stable allowing for at least 500 injections to be performed per chip.
Maximum binding and dilution determination
Injection of excess antibody results in a maximum detector response (Rmax) as the zilpaterol binding sites on the chip are saturated with the antibody. Each Rmax determined for the nine antibodies were shown in . In general, it was observed that polyclonal antibodies produced higher Rmax values in comparison with monoclonal antibodies; however, these antibodies required less dilution to obtain B0s of between 250 and 500 RU, where the instrument response is roughly linear with respect to antibody concentration. This rather steep decline in binding vs. concentration for polyclonal antibodies was probably produced by low affinity binding sites active only at high antibody concentration. The exception to the difference between monoclonal and polyclonal antibodies was monoclonal F140, which was produced using a clenbuterol derivative and tested in this study because of the broad spectrum of beta agonists that it is capable of binding.
Table I. Characterization of a range of polyclonal and monoclonal antibodies to zilpaterol.
Non-specific binding and initial IC50 determination
The instrument reading will decrease to a value close to zero at high zilpaterol concentrations if the antibody binding site is specific for zilpaterol. If the antibody binds to the chip at non-specific sites not displaced by zilpaterol, the instrument will display a residual response at high zilpaterol concentration. An approximation of non-specific binding is the ratio of the reading at high zilpaterol concentration to the reading at zero zilpaterol expressed as a percent. High non-specific binding is undesirable since this decreases the dynamic range to zilpaterol concentrations and reduces the precision of the assay. As shown in , monoclonal antibodies have uniformly low non-specific binding whereas polyclonal antibodies exhibit generally higher and more variable non-specific binding. Rabbit antisera #674 (IC50=1.94 ng/ml) and rabbit antisera #699 (IC50=11.2 ng/ml) were generated using a different linker than the derivative used to immobilize zilpaterol to the sensor chip, hence minimal non-specific binding was observed.
Table II. Initial evaluation of IC50 and non-specific binding for zilpaterol sensor chip with different antibodies.
High sensitivity (low IC50) provides a greater ability to detect zilpaterol residues at low ppb concentrations. Preliminary examination revealed a number of sensitivity differences between the antibodies (see ). For polyclonal antibodies, the sensitivity range was broad, ranging from an IC50 of 0.66 ng/ml to greater than 450 ng/ml. Goat antisera #133 was the most sensitive antibody when performed in HBS buffer, with an IC50 of 0.66 ng/ml, but showed an unacceptably high non-specific binding profile. Goat #134, immunized with the same immunogen as goat #133, was somewhat less sensitive with an IC50 of 1.7 ng/ml but showed minimal non-specific binding. This clearly demonstrated the importance of individually characterizing the antibodies for assay development, since animal differences can be extreme. Rabbit antisera #698 was not sensitive toward zilpaterol, with an IC50 of >300 ng/ml; consequently, its non-specific binding could not be determined. The rank of sensitivity for the monoclonal antibodies was 3H5 > 2E10 > 7A8 > F140 with IC50s of 4.8, 16.9, 31.3, and 56.8 ng/ml respectively. The sensitivity of the monoclonal antibodies 3H5, 2E10, and 7A8 paralleled the sensitivity reported (Shelver et al. Citation2005) and these antibodies showed no cross reactivity toward other beta agonists. The previous work reported the on/off rates determined by an independent method.
Stability determination
In automated procedures it is important that the system remain stable throughout the entire assay procedure. To test this characteristic, the percentage change in B0 after a number of cycles typical of the number used under actual assay conditions was measured. The results are shown in and indicate a variety of different stabilities among the various antibodies tested. The monoclonal antibodies were generally quite stable, although antibody F140 showed a 12% change and 7A8 a 7.5% change that were larger than desirable. The monoclonal 2E10 showed negligible change. The goat polyclonal antibody G134 showed extreme instability with over 40% change and was unacceptable for this type of analysis. The polyclonal antibodies R674, R698, and R699 changed less than 10% but more than the 5% would be deemed an acceptable margin for this type of analysis.
Table III. Stability of antibodies for extended period of runs.
Sensitivity optimization
The operating parameters of the instrument can alter the assay performance since antibody concentration, contact time, and flow rate alter the dynamics of the equilibrium between antibody and immobilized hapten. In general, a decrease in the percentage of antibody increased the sensitivity (decreased IC50) with a concurrent decrease of B0 (indicating decreased antibody binding to the sensor chip). For example, a change in the amount of antibody 2E10 (1:1000) from 40% antibody to 25% antibody changed the IC50 from 50.4 ng/ml to 6 ng/ml with B0 changed from 310 RU to 203 RU. Consequently the optimum amount of antibody is a compromise between dynamic range and sensitivity. An increase change in contact time produced a decrease in sensitivity that plateaued at longer contact times. For example, for 25% 2E10 antibody with contact times of 2, 4, and 8 min the IC50s were 6, 10, and 9.6 ng/ml respectively. With flow rate and exposure time changed to keep the total amount of antibody constant, the flow rate changed B0 to a greater extent than IC50. For example, for 25% antibody at a flow rate of 10 µl/min with a contact time of 3 min, B0 was 437 RU and IC50 4.62 ng/ml, but a flow rate of 30 µl/min with a contact time 1 min gave B0 of 180 RU and IC50 4.46 ng/ml. A similar pattern was observed with other antibodies (data not shown). The optimum conditions selected for 2E10 were a compromise to maximize sensitivity and dynamic range and minimize analysis time and consumption of antibody.
Matrix interference determination
An additional requirement is that the antibody used in the analysis should show minimal interference from the sample matrix as this effect has been shown to deleteriously affect the quality of the analysis. The antibodies showed a great deal of difference in their susceptibility to interference from urine. This interference was predominately an alteration in B0. Although goat antisera #133 was the most sensitive antibody among the nine that were tested, it was subject to considerable matrix interference. Even at 1:5 urine dilution the RU of B0 was only 70% of the original RU of B0 determined in HBS-EP buffer with a negligible change in IC50. Similar results were observed for goat antisera G134. Rabbit sera R674 tolerated the urine matrix with minimal change of B0 and IC50. shows the calibration curve of rabbit sera R674 with different urine dilutions and the calibration curves essentially overlap. Rabbit sera R699 also showed minimal matrix effect, although the IC50 showed slight increases as the urine concentration increased; IC50 values with buffer, urine 1:5, urine 1:2 and undiluted urine were 11.21, 10.92, 13.09, and 13.63 ng/ml, respectively. Monoclonal antibodies also showed differences in urine susceptibility, predominately in terms of the B0. Antibody 2E10 displayed minimal changes, while 3H5 experienced dramatic changes.
Figure 2. Matrix effects of rabbit antiserum #674 with buffer (circle), urine (open circle), urine 1:2 (triangle), urine 1:5 (open triangle), and urine 1:10 (square). The solid line indicates the fit by the 4 parameter logistic equation. (b) Matrix effects of monoclonal antibody 2E10 with buffer (circle), urine (open circle), urine 1:2 (triangle), urine 1:5 (open triangle), urine 1:10 (square), and urine 1:20 (open square). Data represents the mean of two repeated measurements.
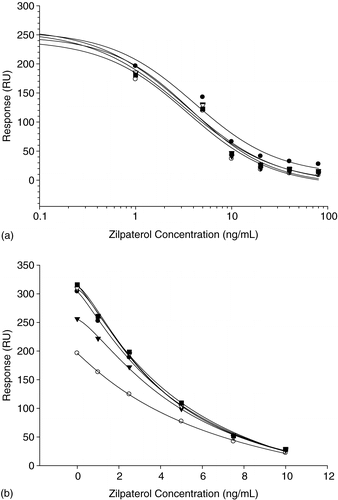
Inter- and intra-assay variation
Sheep urine, diluted 1:5 in phosphate buffer, was chosen to perform the inter- and intra-assay variation tests for 2E10 (see ). This dilution was selected because the resulting zilpaterol standard curves had B0 and IC50 values essentially identical to standard curves run in buffer. The similarity of the standard curves constructed in diluted urine versus buffer strongly indicated that minimal matrix effects were present. The recoveries and the variation measured were considered acceptable with the exception of 1 ng/ml. The inter-assay variation for sheep urine was approximately 21% and recovery was approximately 85% at 1 ng/ml concentration. As this data was obtained at the lower end of the straight-line portion of the calibration curve, this was the probable cause of the greater variance and high recoveries experienced. The zilpaterol biosensor assay was shown to be accurate and repeatable between 2 and 8 ng/ml of zilpaterol. Both inter- and intra-assay variation for spike levels of 2–8 ng/ml were well below 10%.
Table IV. Intra- and inter-assay variation of sheep urine spiked with zilpaterol with zilpaterol sensor chip and monoclonal antibody 2E10.
For this analysis the biosensor required more antibody in comparison to ELISA. For ELISA, the antibody needed was >1:10,000 for monoclonal antibodies 2E10, 3H5, and 7A8, 1:8000 for polyclonal antibody G133, and 1:16000 for polyclonal antibody G134, while the biosensor requires antibody in the dilution of 1:1000 – 1:3000 for monoclonal antibodies and around 1:200 for polyclonal antibodies. The volume of diluted antibody utilized in a biosensor assay (30–40 µl/sample) is generally about half of what is consumed in an ELISA (50 µl/sample). The monoclonal antibody 2E10 showed an IC50 of 0.31 ng/ml for ELISA compared with 4.47 ng/ml for the biosensor method. The rationale for these differences could be in fundamental processing differences in the assays (equilibrium vs. kinetic) or in the chemistry involved in the immobilizing methodology involved in the assays.
Comparison of ELISA with biosensor analysis for the measurement of zilpaterol in incurred samples demonstrated that the methods produce similar results (see ). Both ELISA and biosensor can tolerate matrix interference and require minimal sample treatment. As expected the biosensor was less susceptible to matrix interference tolerating a 1:5 urine dilution compared to ELISA that required a 1:10 dilution. In both cases, the urine samples only required dilution with phosphate buffer prior to measurement. The correlation between the two methods was high, with an r2=0.91, while the slope was close to 1, demonstrating that either method can serve as a good screening tool for zilpaterol urine measurement.
Figure 3. Correlation between ELISA and biosensor measurements for incurred zilpaterol sheep urine samples (n=60, range 0.9–5920 ng/ml).
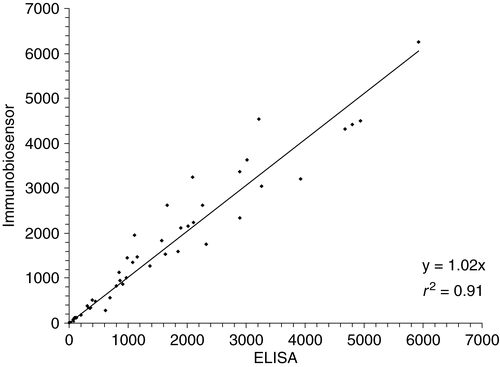
In conclusion, nine zilpaterol antibodies have been evaluated for their sensitivity, tolerance toward sheep urine, and stability for extended period of assay (over 6 h). A stable, reproducible, and sensitive method of analysing zilpaterol in urine samples using biosensor technology has been developed. This technology allows for the automated and rapid analysis of samples for zilpaterol content. Although the ELISA analysis was more sensitive, direct comparison of the two methods using incurred samples gave equivalent results. The biosensor analysis is convenient, readily automated, and an excellent method of analysis.
Acknowledgments
The authors wish to acknowledge Amy McGarvey for technical support and Imelda Traynor for helpful discussions.
Additional information
Notes on contributors
Weilin L. Shelver‡
‡Collaborator, via a fellowship under the OECD co-operative Research Program: Biological Resource Management for Sustainable Agriculture Systems.Notes
*The use of trade, firm, or corporation names in this publication is for the information and convenience of the reader. Such use does not constitute an official endorsement or approval by the United States Department of Agriculture or the Agricultural Research Service of any product or service to the exclusion of others that may be suitable.
References
- Adam , A , Ong , H , Sondag , D , Rapaille , A , Marleau , S , Bellemare , M , Raymond , P , Giroux , D , Lee , JK and Beaulieu , N . 1990 . Radioimmunoassay for albuterol using a monoclonal antibody: application for direct quantification in horse urine . J Immunoassay , 11 : 329 – 435 .
- Baxter , GA , Ferguson , JP , O'Connor , MC and Elliott , CT . 2001 . Detection of streptomycin residues in whole milk using an optical immunobiosensor . J Agric Food Chem , 49 : 3204 – 3207 .
- Bergström , C , Sternesjö , Å , Bjurling , P and Löfås , S . 1999 . Design and use of a general capturing surface in optical biosensor analysis of sulphamethazine in milk . Food Agric Immunol , 11 : 329 – 338 .
- Bocca , B , Fiori , M , Cartoni , C and Brambilla , G . 2003a . Simultaneous determination of zilpaterol and other beta agonists in calf eye by gas chromatography/tandem mass spectrometry . J AOAC Int , 86 : 8 – 14 .
- Bocca , B , Di Mattia , M , Cartoni , C , Fiori , M , Felli , M , Neri , B and Brambilla , G . 2003b . Extraction, clean-up and gas chromatography-mass spectrometry characterization of zilpaterol as feed additive in fattening cattle . J Chromatography B , 783 : 141 – 149 .
- Elliott , CT , Baxter , GA , Hewitt , SA , Arts , CJM , van Baak , M , Hellenas , K and Johannson , A . 1998a . Use of biosensors for rapid drug residue analysis without sample deconjugation or clean-up: A possible way forward . Analyst , 123 : 2469 – 2473 .
- Elliott , CT , Thompson , CS , Arts , CJ , Crooks , SR , van Baak , MJ , Verheij , ER and Baxter , GA . 1998b . Screening and confirmatory determination of ractopamine residue in calves treated with growth promoting doses of the β-agonist . Analyst , 123 : 1103 – 1107 .
- Ferguson , JP , Baxter , GA , McEvoy , JD , Stead , S , Rawlings , E and Sharman , M . 2002 . Detection of streptomycin and dihydrostreptomycin residues in honey and meat samples using an optical biosensor . Analyst , 127 : 951 – 956 .
- Fleeker , JR and Lovett , LJ . 1985 . Enzyme immunoassay for screening sulfamethazine residues in swine blood . J AOAC , 68 : 172 – 174 .
- Gustavsson , E and Sternesjo , A . 2004 . Biosensor analysis of beta-lactams in milk: Comparison with microbiological, immunological, and receptor-based screening methods . J AOAC Int , 87 : 614 – 620 .
- Haasnoot , W , Stouten , P , Lommen , A , Cazemier , G , Hooijerink , D and Schilt , R . 1994 . Determination of fenoterol and ractopamine in urine by enzyme immunoassay . Analyst , 119 : 2675 – 2680 .
- Haughey , SA , Baxter , GA , Elliott , CT , Persson , B , Jonson , C and Bjurling , P . 2001 . Determination of clenbuterol residues in bovine urine by optical immunobiosensor assay . J AOAC Int , 84 : 1025 – 1030 .
- Karlsson , R , Michaelsson , A and Mattsson , L . 1991 . Kinetic analysis of monoclonal antibody-antigen interactions with a new biosensor based analytical system . J Immunolog Methods , 145 : 229 – 240 .
- Kuiper , HA , Noordam , MY , van Dooren-Flipsen , MMH , Schilt , R and Roos , AH . 1998 . Illegal use of β-adrenergic agonists: European community . J Animal Sci , 76 : 195 – 207 .
- Mitchell , GA and Dunnavan , G . 1998 . Illegal use of β-adrenergic agonists in the United States . J Animal Sci , 76 : 208 – 211 .
- Plascencia , A , Torrentera , N and Zinn , RA . 1999 . Influence of the β-agonist, zilpaterol, on growth performance and carcass characteristics of feed lot steers . Proceedings, Western Section, American Society of Animal Science , 50 : 331 – 334 .
- Salinas-Chavira , J , Ramirez , RG , Dominguez-Muňoz , M , Palomo-Cruz , R and Lopez-Acuňa , VH . 2004 . Influence of zilpaterol hydrochloride on growth and carcass characteristics of pelibuey lambs . J Appl Animal Res , 26 : 13 – 16 .
- Samsonova , JV , Baxter , GA , Crooks , SR , Small , AE and Elliott , CT . 2002 . Determination of ivermectin in bovine liver by optical immunobiosensor . Biosens Bioelectronics , 17 : 523 – 529 .
- Samsonova , JV , Uskova , NA , Andresyuk , AN , Franek , M and Elliott , CT . 2004 . Biacore biosensor immunoassay for 4-nonylphenols: assay optimization and application for shellfish analysis . Chemosphere , 57 : 975 – 985 .
- Shelver , WL and Smith , DJ . 2000 . Development of an immunoassay for the β-adrenergic agonist ractopamine . J Immunoassay , 21 : 1 – 23 .
- Shelver , WL , Smith , DJ and Berry , ES . 2000 . Production and characterization of a monoclonal antibody against the β-adrenergic agonist ractopamine . J Agric Food Chem , 48 : 4020 – 4026 .
- Shelver , WL and Smith , DJ . 2004 . Enzyme-linked immunosorbent assay development for the β-adrenergic agonist zilpaterol . J Agric Food Chem , 52 : 2159 – 2166 .
- Shelver , WL , Kim , H-J and Li , QX . 2005 . Development of monoclonal antibody based ELISA for the β-adrenergic agonist zilpaterol . J Agric Food Chem , 53 : 3273 – 3280 .
- Stachel , CS , Radeck , W and Gowik , P . 2003 . Zilpaterol-a new focus of concern in residue analysis . Analytica Chimica Acta , 493 : 63 – 67 .
- Van Hoof , N , Schilt , R , van der Vlis , E , Boshuis , P , Van Baak , M , Draaijer , A , De Wasch , K , Van de Hende , J , Courtheyn , D and De Brabander , H . 2005 . Detection of zilpaterol (Zilmax) in calf urine and faeces with liquid chromatography-tandem mass spectrometry . Analytica Chimica Acta , 529 : 189 – 197 .
- Yamamoto , I and Twata , K . 1982 . Enzyme immunoassays for clenbuterol, a β2-adrenergic stimulant . J Immunoassays , 3 : 155 – 171 .