Abstract
A sensitive enzyme-linked immunosorbent assay (ELISA) for the detection of neomycin was developed. Three conjugates were used as immunogens to immunize rabbits. Titers and IC50 values of antisera from various bleeds of rabbits were monitored to confirm final-bleeding time. Final antisera were then screened by competitive indirect ELISA (ciELISA) using homologous and heterologous coating antigen. Thereafter, the effect of different types of hapten heterology on ciELISA sensitivity was evaluated. The results showed that the degree of hapten heterology parallels the improvement of ciELISA sensitivity. The optimized ELISA gave an IC50 value of 0.098 ng/ml, and it showed negligible cross-reactivity with other aminoglycosides. The recoveries of neomycin from spiked milk at levels of 2.5–20 ng/ml ranged from 87 to 109%, and the detection limit was 0.69 ng/ml in milk.
Introduction
Neomycin is a widely used broad spectrum aminoglycoside antibiotic that inhibits the growth of both Gram-positive and Gram-negative bacteria (Waksman et al. Citation1949). It may cause ototoxicity and nephrotoxicity in cases of overdosing (Clark Citation1977). Therefore, the presence of neomycin in food is potentially hazardous to human health. For consumer protection, the European Agency for the Evaluation of Medical Products (EMEA) has established maximum residue limits (MRLs) for neomycin in edible tissues and milk: 500 µg/kg for meat, 500 µg/kg for liver, 5000 µg/kg for kidney, and 500 µg/kg for milk (EMEA Citation2002).
In order to monitor residue levels of neomycin in animal-origin food, a number of analytical methods, mainly microbiological assay (Stahl & Kratzer Citation1984; Shen & Xiao Citation1999), high-performance liquid chromatography (HPLC) (Posyniak et al. Citation2001; Kaufmamn & Maden Citation2005), and enzyme-linked immunosorbent assay (ELISA) (Haasnoot et al. Citation1999; Loomans et al. Citation2003; Jin et al. Citation2006) have been developed. For screening purposes, ELISA is advantageous to other methods because of its high sensitivity, high throughput, and rapid turnaround time. Though Haasnoot et al. (Citation1999) developed an ELISA for the detection of aminoglycosides in milk, high background levels were observed. Loomans et al. (Citation2003) and Jin et al. (Citation2006) also reported ELISAs for neomycin in milk respectively, but the sensitivities were not very high, and their methods need control milk to prepare standard solutions, which would fail to give accurate results when control milk samples were not available. The aim of our study is to develop a sensitive ELISA for the detection of neomycin in milk in which matrix interference was minimized, and no control milk samples were required for preparing standard solutions.
Hapten heterology in competitive indirect ELISA (ciELISA) is termed to indicate the differences of hapten between immunogen and coating antigen in spacer arm, structure, or linking site. It was demonstrated previously that hapten heterology is an important factor for improving the sensitivity of immunoassays (Kitagawa et al. Citation1983; Harrison et al. Citation1991; Kim et al. Citation2003). However, no study has been conducted to investigate the effect of hapten heterology on ELISA sensitivity of 2-deoxystreptamine aminoglycosides. Another aim of our study is to reveal the relationship between the hapten heterology and ELISA sensitivity of neomycin.
Material and methods
Reagents and instruments
Neomycin sulfate, streptomycin sulfate, gentamicin sulfate, kanamycin sulfate, amikacin sulfate, tobramycin sulfate and neamine were obtained from the China National Institute for the Control of Pharmaceutical and Biological products (Beijing, China). Bovine serum albumin (BSA), human serum albumin (HSA), ovalbumin (OVA), complete Freund's adjuvant (CFA), incomplete Freund's adjuvant (IFA), goat anti-rabbit IgG-horseradish peroxidase (HRP) conjugate, tetramethylbezidine (TMB) and 1-ethyl-3-(3-dimethylaminopropyl)carbodiimide (EDC) were purchased from Sigma-Aldrich (St. Louis, MO, USA). Formaldehyde, glutaraldehyde, sodium borohydride and sodium periodate were obtained from Beijing Xinjingke Biotechnology Corporation (Beijing, China). Other reagents were supplied by Beijing Regent Corporation (Beijing, China). Polystyrene microtiter plates were obtained from Beijing Wanger Biotechnology Corporation (Beijing, China). ELISA plate reader was purchased from TECAN Inc. (Durham, NC, USA).
Preparation of immunogen
Carbodiimide (EDC) coupling method
Neomycin-BSA and neomycin-HSA conjugates were synthesized according to the procedure described by Wantanabe et al. (Citation1999). Briefly, neomycin (50 mg) and BSA or HSA (20 mg) were dissolved in 2 ml of 0.01 mol/l phosphate buffer saline (PBS; pH 6.5), and EDC (20 mg) dissolved in 1 ml of PBS (pH 6.5) was added dropwise. After a reaction for 2 h at room temperature, the solution was stirred for 3 days at 4°C. Finally, the reaction product was dialyzed (over 3 days at 4°C) against PBS.
Glutaraldehyde (GDA) coupling method
Neomycin sulfate (20 mg) and BSA (20 mg) were dissolved in 10 ml of 0.01 mol/l PBS, the pH value of which was adjusted to 6.5. Then, 1.5 ml of freshly prepared 1% glutaraldehyde aqueous solution was added dropwise. After the reaction mixture was gently stirred for 15 min, sodium borohydride was added to a final concentration of 10 mg/ml, and the solution was incubated for 1 h at 4°C. Finally, the reaction product was dialyzed (over 3 days at 4°C) against PBS, and then concentrated with Amicon Ultra-15 Centrifugal Filter Units (Millipore, USA). The immunogen produced by this method was designated as neomycin-G-BSA.
Preparation of coating antigen
EDC coupling method
Neomycin-OVAE and neamine-OVA conjugates were prepared by the EDC method as indicated above.
Formaldehyde coupling method
Neomycin-F-OVA conjugate was prepared as follows: neomycin sulfate (20 mg) and OVA (20 mg) were dissolved in 10 ml of 0.01 mol/l PBS, the pH value of which was adjusted to 6.5. Then, 3 ml of freshly prepared 1% formaldehyde aqueous solution was added dropwise. After the reaction mixture was gently stirred for 5 h, the reaction product was dialyzed against PBS.
GDA coupling method
Neomycin-G-OVA and neamine-G-OVA conjugates were prepared by the GDA coupling method as described above.
Periodate coupling method
Neomycin-OVAP conjugate was prepared as follows: neomycin sulfate (40 mg) was dissolved in 1 ml of water, the pH value of which was adjusted to 3.0–4.0. Next, 0.25 ml of 0.1 mol/l sodium periodate solution was added, and the solution was reacted for 3 h at 4°C, followed by the addition of glycol (50 µl) to terminate the reaction. This solution was then added into the OVA solution (5 mg, dissolved in 1 ml of 0.01 mol/l PBS) dropwise. After the reaction mixture was gently stirred for 2 h, 50 mg of sodium borohydride was added and the solution was incubated for 1 h at 4°C. Finally, the reaction product was dialyzed against PBS.
After centrifugation to remove the precipitate, the absorbance values of immunogens and coating antigens were determined at 280 nm by ultraviolet-visible (UV-vis) spectra photometer, and the concentrations were calculated by external reference method (Zhou et al. Citation2001).
Immunization of rabbits
Four female New Zealand white rabbits were divided into three groups: two for neomycin-BSA immunogen, one for neomycin-HSA immunogen, and one for neomycin-G-BSA immunogen.
Immunogen (2 mg) for each rabbit was dissolved in 1 ml of 0.01 mol/l PBS (pH 7.4), and emulsified with 1 ml of CFA. The emulsion was injected intracutaneously in the primary immunization. For booster immunizations, 1 mg immunogen for each rabbit was dissolved in 0.5 ml of the above buffer and emulsified with 0.5 ml of IFA. The emulsion was then injected subcutaneously. The booster immunizations were repeated every 3 weeks. Rabbits were bled through an ear vein 1 week after each booster injection (after the third immunization). To obtain antisera, blood samples were stored to coagulate for 1 h at room temperature and overnight at 4°C, followed by centrifugation at 3500×g for 10 min. The supernatants were carefully collected, divided into aliquots and stored at –20°C until use.
ELISA procedure
Indirect ELISAs were performed as follows: polystyrene microtiter plate wells were coated with 100 µl of coating antigen in 0.05 mol/l carbonate/bicarbonate buffer (pH 9.6) by overnight incubation at 4°C. After coating, plates were blocked for 2 h at 37°C with 200 µl per well of blocking solution (0.01 mol/l PBS containing 0.5% casein). The solution was then discarded and plates were washed three times with washing solution (0.01 mol/l PBS containing 0.05% Tween-20). Then, 100 µl per well of antibody dilution prepared in assay buffer (0.01 mol/l PBS) was added and incubated for 1 h at 37°C. After another washing step, 100 µl per well of HRP labeled goat anti-rabbit IgG, in a dilution of 1:5000 in dilution buffer (0.01 mol/l PBS), was added, and the plates were incubated for 1 h at 37°C. The final washing procedure was followed by a color development, which was initiated by adding 100 µl of the substrate/chromogen solution (TMB/H2O2 in acetate buffer, pH 5.5). The solution was incubated for 15 min at 37°C before the enzymatic reaction was stopped by adding 2 mol/l H2SO4 (50 µl/well). The optical density (OD) of each well was measured at 450 nm by an enzyme immunoassay reader. The titer of antiserum was defined as the dilution factor which gave 2.1 times higher OD values than the pre-immune control serum. The optimum concentration of the coating antigen and antibody for ciELISA was optimized by checkerboard titration.
The ciELISA procedure was the same as the ELISA described above except that after coating, a competition step was introduced by adding 50 µl of analyte (prepared in assay buffer, 0.01 mol/l PBS) followed by 50 µl of appropriate concentration of antibody. Competition curves were obtained by plotting absorbance against the logarithm of analyte concentrations, which were fitted to a four-parameter logistic equation: , where A is the maximum absorbance at no analyte, B is the curve slop at the inflection point, C is the concentration of analyte giving 50% inhibition (IC50), and D is the minimum absorbance at infinite concentration (Li et al.
Citation2002). The IC50 value was expressed as the sensitivity of ELISA.
Screening of antisera by ELISA
The most suitable time of final-bleeding was determined according to the change of titers and IC50 values of antisera from different bleeds of rabbits. Indirect ELISA and competitive indirect ELISA were carried out using neomycin-OVAE as coating antigen to determine the titers and IC50 values, respectively. To obtain the best antiserum, the titers and IC50 values of final antisera from different rabbits were determined by indirect ELISA and competitive indirect ELISA, respectively. In these experiments, neomycin-OVAE and neomycin-G-OVA conjugates were selected as coating antigens.
Determination of the effect of hapten heterology on ELISA sensitivity
Different types of heterologous coating antigens were tested for ciELISA to evaluate the effect of hapten heterology on the sensitivity of ELISA. The concentration of each coating antigen was optimized to obtain the best sensitivity, and IC50 value was expressed as the sensitivity of ELISA.
Optimization of ELISA conditions
The ciELISA was further optimized on the basis of the antiserum and coating antigen screened by the above experiments. The assay buffer with various pH values (5.0, 6.0, 7.0, 8.0 and 9.0) and ionic strengths (0.04, 0.08, 0.15, 0.3 and 0.6 mol/l of NaCl) were tested to maximize the assay sensitivity of ELISA. The optimum condition was evaluated by IC50 value.
Determination of cross-reactivities
Several aminoglycosides (streptomycin, gentamicin, kanamycin, apramycin, tobramycin) were tested for cross-reactivity using the ciELISA procedure described above. The cross-reactivity values were calculated as follows:
Analysis of spiked milk samples
Neomycin solution (1000 ng/ml, prepared in water) was added to milk to produce spiked concentration of 2.5, 5, 10, and 20 ng/ml. The spiked milk sample (10 ml) was then transferred into a 50-ml polypropylene tube. After trichloroacetic acid (200 µl of 50%) was added, the sample was mixed briefly and centrifuged at 3500×g for 10 min to deproteinate. The upper fat layer was dislodged, and 200 µl of the clear supernatant was then diluted with the optimized assay buffer, 50 µl aliquots of which were then pipetted into the microtiter plate for analysis. The recoveries of neomycin from the spiked milk samples were calculated based on the standard curves constructed by ciELISA. The detection limit was determined as the mean background levels plus 3×standard deviation.
Results and discussion
Preparation of immunogen and coating antigen
EDC and GDA methods were used to synthesize immunogens, both using a reactive amine group on neomycin to conjugate the hapten to carrier protein. In the first method, neomycin was conjugated directly to the carboxyl of carrier protein using carbodiimide as active agent (Chu et al. Citation1976). An excess of neomycin was used to minimize intraprotein cross-linking and maximize hapten attachment. In the second method, glutaraldehyde was used as cross-linking reagent to link amine groups of both neomycin and carrier protein (Arameas et al. Citation1969). In this experiment, excess glutaraldehyde or excess reaction time will result in the appearance of yellow floccule, which may be caused by the formation of neomycin-carrier protein cross-linking polymer, therefore, 1.5 ml of 1% glutaraldehyde solution was added, and the reaction was terminated by sodium borohydride after 15 min.
Four coupling methods (EDC, formaldehyde, GDA and periodate methods) were used to couple neomycin to OVA to form different types of coating antigen. The water soluble carbodiimide EDC is one of the so-called zero-length cross-linker, since it mediates the formation of an amide linkage without leaving a spacer molecule, therefore, carbodiimide coupling method could provide zero-length spacer arm coating antigen (Chu et al. Citation1976). Conjugation reactions using formaldehyde may proceed in two ways: via immonium cation intermediate or Mannich reaction (Hermanson Citation1996). As the Mannich reaction proceeds at a slower rate at mild reaction conditions, such as room temperature and low formaldehyde concentration, formaldehyde-facilitated reaction in this study was supposed to occur primarily by the immonium ion pathway to crosslink the amines of neomycin and OVA, providing one-atom-length spacer arm coating antigen. While glutaraldehyde as a homobifunctional cross-linker could also link the amines of both neomycin and OVA, providing five-atom-length spacer arm coating antigens (Arameas et al. Citation1969). Since neomycin has vicinal hydroxyl groups (), which are prone to oxidation by oxidant, sodium periodate was used to rupture the vicinal hydroxyl structure, and oxidize them to aldehyde which could then reacted with the amine of carrier protein. As the structure of neomycin was disrupted, the linking site and spacer arm of this coating antigen also differ from immunogens, the conjugate synthesized by periodate coupling method could be considered structure, site and spacer arm heterologous coating antigen as well. Neamine, as a two ring fragment of neomycin (), has previously been used as an immunizing hapten for the production of generic antibodies against neomycin, gentamicin and kanamycin (Loomans et al. Citation2003). Herein, we used it as a hapten coupled to OVA for synthesizing structure heterologous coating antigen via carbodiimide and glutaraldehyde coupling methods.
As neomycin has no UV absorbance, the concentrations of immunogens and coating antigens were directly determined at 280 nm by UV-vis spectra photometer.
Screening of antisera by ELISA
The titers and IC50 values of antisera from various bleeds of rabbits remained slightly changed after the fifth immunization (), therefore, final bleeding was carried out after the sixth immunization.
Figure 2. Titer (upper panel) and IC50 (lower panel) change curves of antisera from various bleeds of rabbits. Neomycin-OVAE was used as coating antigen at the concentration of 1 µg/ml. The titer of antiserum was defined as the dilution factor which gave 2.1 times OD values than negative serum. Each point represents the average of three well replicates.
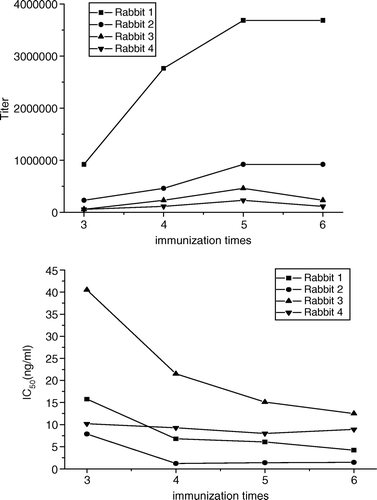
The results of titers and IC50 values of final antisera to neomycin-OVAE and neomycin-G-OVA are shown in . The titers of antisera against neomycin-BSA were significantly higher than that of antiserum against neomycin-HSA, while the IC50 values were lower, which indicated that BSA may be a better carrier protein than HSA. Neomycin-BSA with zero-length spacer arm and neomycin-G-BSA with five-atom-length spacer arm were used as immunogens to evaluate the effect of spacer arm length of immunogen on antibody production. The results showed that neomycin-BSA would be a better immunogen compared with neomycin-G-BSA. For the four antisera, both higher titers and higher IC50 values were obtained using homologous coating antigen than that using heterologous coating antigen. This was probably caused by the recognition of antibodies to the spacer arm or cross-linking sites between neomycin and carrier protein, which indicates that the displacement of antibodies binding to homologous coating antigens could not be accomplished completely by neomycin. Despite homologous or heterologous coating antigen, Serum 2, which was obtained from the second rabbit using neomycin-BSA as immunogen, showed the highest sensitivity, therefore, it was selected for the subsequent experiments.
Table I. Titers and IC50 values of final antisera to neomycin-OVAE and neomycin-G-OVA.
Evaluation of the effect of hapten heterology on ELISA sensitivity
Before the IC50 values of different heterologous coating antigens were determined, the concentration of antiserum and coating antigens was optimized to give the best ELISA sensitivity. The B0 values were controlled at 1.5–2.0 to minimize the analysis errors. The IC50 values of ciELISAs under optimum concentration of antiserum and coating antigens are shown in . Both neomycin-F-OVA and neomycin-G-OVA, which are heterologous in spacer arm, showed better sensitivity than the completely homologous coating antigen (neomycin-OVAE). The three standard curves of ELISAs using neomycin-OVAE, neomycin-F-OVA and neomycin-G-OVA as coating antigens are shown in , respectively. Compared to the neomycin-F-OVA and neomycin-G-OVA standard curves, the neomycin-OVAE standard curve has more shallow slope and higher background value. As the immunogen and the coating antigen neomycin-OVAE were both synthesized by EDC coupling method, the antibodies may recognize the cross-linking site of coating antigen, and may also combine with some by-products of EDC reaction which exist in both immunogen and coating antigen. Spacer arm heterologous coating antigens, however, exclude the possibility of the recognition of polyclonal antibodies to cross-linking site or by-products, and, thus, lead to better ELISA sensitivity. This phenomenon was also previously demonstrated in estrogen ELISA development (Van Weemen & Schuurs Citation1975). Kim et al. (Citation2003) reported that hapten of shorter spacer arm demonstrated higher sensitivity due to the masking of hapten molecule. In contrast, our study results indicated that the sensitivity of ELISA using neomycin-F-OVA as coating antigen did not show significant difference with that of neomycin-G-OVA. This suggests that the length of spacer arm is not an important factor for improving the sensitivity of neomycin ELISA, which may be explained by the extended structure of neomycin. The lower IC50 value with neamine-G-OVA is not surprising, since this coating antigen is heterologous in structure and spacer arm. The structure heterology may weaken the affinity of antibodies to coating antigen compared to neomycin, therefore, the binding of antibodies to coating antigen could be easier to compete for by a minute amount of neomycin, which results in better assay sensitivity (Kitagawa et al. Citation1983). However, a notable feature observed in is the low sensitivity with neamine-OVA. This may be caused by both the weak recognition of antibodies to neamine because of its structure heterology, and the strong recognition of antibodies to the cross-linking site due to its homology with immunogen. The highest sensitivity improvement was brought about by neomycin-OVAP, which is heterologous in structure as well as in site and spacer arm. Since the structure heterology of neomycin-OVAP is much less than that of neamine-G-OVA, it is supposed that the site heterology of neomycin-OVAP would also develop an important effect on the sensitivity improvement. In any case, the greatest hapten heterology brought about the highest sensitivity improvement. This result was similar to the study of Kim et al. (Citation2003) for the development of fenthion ELISA, which also showed that the highest sensitivity improvement comes from heterology in site, structure and spacer arm as well.
Figure 3. Representative standard curves of the neomycin ELISA using neomycin-OVAE (▪), neomycin-F-OVA (•) and neomycin-G-OVA (▴) as coating antigen, respectively. B and B0 are the absorbances of the sample with/without neomycin, respectively. Each point represents the average of three well replicates, and error bars represent standard deviations.
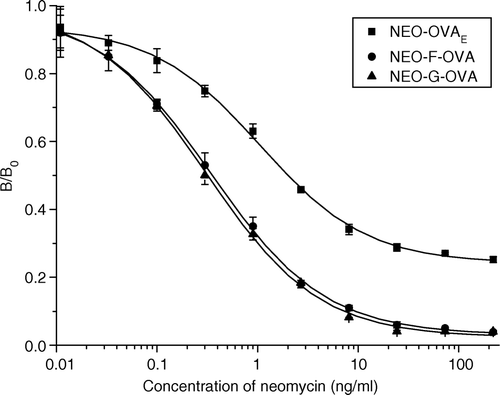
Table II. B0 and IC50 values of ciELISA under optimum concentration of antiserum and coating antigens.
Concluding the above results, hapten heterology significantly improved neomycin ELISA sensitivity, and the improvement parallels the degree of hapten heterology. This could be attributed to the fact that hapten heterology eliminates the strong affinity of polyclonal antibodies to spacer arm or cross-linking site, or that hapten heterology results in a weaker recognition of antibodies to coating antigen compared to target analyte.
Optimization of ELISA conditions
Neomycin is a water-soluble antibiotic and has several amines and hydroxyl groups in its structure, so it is expected that the interaction between neomycin and antibody could be easily influenced by pH and the ion strength of assay buffer. The results showed that the assay buffer of pH 7.0 and 0.15 mol/l NaCl gave the lowest IC50 value of 0.098 ng/ml.
Assay specificity
The neomycin ELISA was very specific and the cross-reactivity values with several frequently used aminoglycosides were <0.1%. The specificity of this ELISA can be explained by the differences in molecular structure of aminoglycosides. All consist of two or more aminosugars linked by glycosidic bonds to a cyclitol component, which is either streptidine (found in streptomycin) or 2-deoxystreptamine (found in neomycin, gentamicin and kanamycin). The kind of 2-deoxystreptamine aminoglycosides can be further divided into three subclasses according to the pattern of substitution on cyclitol. The neomycin subclass is 4,5-disubstituted deoxystreptamines, while the gentamicin and kanamycin subclasses are 4,6-disubstituted deoxystreptamines. The aminosugars attached to cyclitol also differ in numbers and structure (Stead Citation2000). Therefore, the great structure differences result in the high assay specificity of neomycin ELISA. Previous studies showed that antibodies raised to immunogens containing specific aminoglycoside antibiotics were also fairly specific (Haasmoot et al. 1999; Jin et al. 2005, Citation2006). However, Loomans et al. (Citation2003) developed polyantibodies able to recognize getamicin, kanamycin and neomycin using neamine as hapten. This means that generic antibodies can be raised by utilizing the common structure of aminoglycosides as hapten.
Recovery of neomycin-spiked milk samples
A study conducted by Haasnoot et al. (Citation1999) showed that the recovery of neomycin from direct dilution of milk samples was only 73 and 47% at 2.5 and 10 ng/ml spiked level respectively, therefore, we used trichloroacetic acid to deproteinate and to increase the recovery of neomycin from milk. Compared with the direct dilution method, the use of trichloroacetic acid did not add an additional step for sample preparation. The extract was directly diluted with the optimized assay buffer without the need to adjust pH values. The influence of dilution folds on ELISA performance was also investigated. As shown in , the absorbance at each concentration in 50-fold diluted milk solution was almost the same as that in the assay buffer. Therefore, 50-fold dilution was sufficient to eliminate the matrix effect on assay performance. On the basis of the standard curves constructed with standard solutions in assay buffer, the recoveries of neomycin from spiked milk at levels of 2.5, 5, 10, 20 ng/ml ranged from 87 to 109% (). The detection limit, which was defined as the mean background level plus 3×SD was 0.69 ng/ml in milk (n=20).
Figure 4. Influence of matrix in milk on the neomycin ELISA standard curve. The dilution times of milk in assay buffer (PBS, pH 7.0) were as follows: control (▪), 50-fold (•), 20-fold (▴), and 10-fold (▾). Each point represents the average of three well replicates, and error bars represent standard deviations.
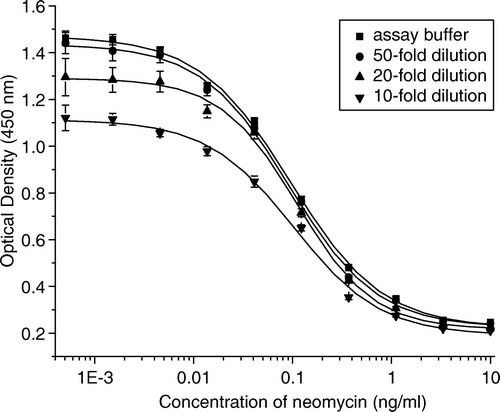
Table III. Recoveries of neomycin from spiked milk (n=4).
In conclusion, we prepared a high affinity antibody and developed a highly sensitive ELISA for neomycin. The IC50 value of this optimized ELISA is 0.098 ng/ml. Matrix interference is minimized by only diluting sample extracts. The developed ELISA is capable of monitoring neomycin residue levels in biological matrixes, even though lower MRLs far below those currently established by EMEA are regulated. In addition, the effect of hapten heterology on ELISA sensitivity will also provide a good guideline for the subsequent immunochromatographic assay study in which sensitivity improvement is generally of much concern.
References
- Arameas , S and Ternynck , T . 1969 . The cross-linking of proteins with glutaraldehyde and its use for the preparation of immunosorbents . Immunochemistry , 6 : 53 – 66 .
- Chu , FS , Fred Chi , C and Hinsdill , RD . 1976 . Production of antibody against ochratoxin A . Appl Environ Microbiol , 31 : 831 – 835 .
- Clark , CH . 1977 . Toxicity of aminoglycoside antibiotics . Mod Vet Pract , 53 : 594 – 598 .
- EMEA . 2002 . Emea/Mrl/816/02-Final. Available: http://www.emea.europa.eu/pdfs/vet/mrls/0816-02en.pdf via the INTERNET.
- Haasnoot , W , Stouten , P , Cazemier , G , Lommen , A , Nouws , JFM and Keukens , HJ . 1999 . Immunochemical detection of aminoglycosides in milk and kidney . Analyst , 124 : 301 – 305 .
- Harrison , RO , Goodrow , MH and Hammock , BD . 1991 . Competitive inhibition ELISA for the s-triazine herbicides: Assay optimization and antibody characterization . J Agric Food Chem , 39 : 122 – 128 .
- Hermanson , GT . 1996 . Homobifunctional cross-linkers in bioconjugate techniques , 188 – 229 . London : Academic Press .
- Jin Y , Jang JW , Han CH , Lee MH . Development of ELISA and immunochromatographic assay for the detection of gentamicin . J Agric Food Chem 53 : 7639 – 7643 .
- Jin , Y , Jang , JW , Lee , MH and Han , CH . 2006 . Development of ELISA and immunochromatographic assay for the detection of neomycin . Clin Chim Acta , 364 : 260 – 266 .
- Kaufmamn , A and Maden , K . 2005 . Determination of 11 aminoglycosides in meat and liver by liquid chromatography with tandem mass spectrometry . J AOAC Int , 88 : 1118 – 1125 .
- Kim , YJ , Cho , YA , Lee , HS and Lee , YT . 2003 . Investigation of the effect of hapten heterology on immunoassay sensitivity and development of an enzyme-linked immunosorbent assay for the organophosphorus insecticide fenthion . Anal Chim Acta , 494 : 29 – 40 .
- Kitagawa , T , Fujiwara , K , Tomonoh , S , Takahashi , K and Koida , M . 1983 . Enzyme immunoassays of kanamycin group antibiotics with high sensitivities using anti-kanamycin as a common antiserum: Reasoning and selection of a heterologous enzyme label . J Biochem , 94 : 1165 – 1172 .
- Li JS , Qiu YM , Wang C . 2002 . Immunoassay methods . In : Veterinary drug residue analysis . 1st ed . Shanghai, , China : Shanghai Science and Technology Publisher . pp 184 – 185 .
- Loomans , EEMG , Wiltenburg , JV , Koets , M and Amerongen , AV . 2003 . Neamin as an immunogen for the development of a generic ELISA detecting gentamicin, kanamycin, and neomycin in milk . J Agric Food Chem , 51 : 587 – 593 .
- Posyniak , A , Zmudzki , J and Niedzielska , J . 2001 . Sample preparation for residue determination of gentamicin and neomycin by liquid chromatography . J Chromatogr A , 914 : 59 – 66 .
- Shen , C and Xiao , XL . 1999 . Studies on elimination of neomycin residues in chicken . Chinese J Vet Drug , 33 : 3 – 5 .
- Stahl , G and Kratzer , D . 1984 . Microbiological determination of neomycin in feeds and formulated products . J AOAC Int , 67 : 863 – 865 .
- Stead , DA . 2000 . Current methodologies for the analysis of aminoglycosides . J Chromatogr B , 747 : 69 – 93 .
- Van Weemen , BK and Schuurs , A . 1975 . The influence of heterologous combinations of antiserum and enzyme-labeled estrogen on the characteristics of estrogen enzyme immunoassay . Immunochemistry , 12 : 667 – 670 .
- Waksman , SA and Lechevaller , HA . 1949 . Neomycin, a new antibiotic active against streptomycin resistant bacteria, including tuberculosis organisms . Science , 109 : 305 – 307 .
- Wantanabe , H , Satake , A , Kido , Y and Tsuji , A . 1999 . Production of monoclonal antibody and development of enzyme-linked immunosorbent assay for kanamycin in biological matrices . Analyst , 124 : 1611 – 1615 .
- Zhou SW , Tang AF , Zhou SX , Zhao G . 2001 . Methods for determination of protein concentration In : Experimental guidelines of animal biochemistry . 2nd ed . Beijing, , China : China Agricultural Press . pp 55 – 56 .