Abstract
The objective of this study was to examine the effect of maternal immunisation against myostatin, a potent negative regulator of skeletal muscle growth, on the skeletal muscle growth of offspring in mice. Twenty female mice were divided into four groups and immunised against keyhole limpet haemocyanin (control) or recombinant mature myostatin (rMyo), or two different myostatin synthetic peptides conjugated to keyhole limpet haemocyanin (Myo1 and Myo2). Offspring were sacrificed at 8 weeks of age to measure carcass, muscle and fat masses. Sera collected from 3-day-old neonates of the rMyo, Myo1 and Myo2 groups showed titres against myostatin, confirming the maternal transfer of anti-myostatin antibodies to offspring. No significant effects of immunisation on offspring weight were observed. The offspring of the Myo2 group, but not the rMyo and Myo1 groups, had significantly heavier gastrocnemius and triceps muscle mass than those of the control (p<0.01). All IgGs of the three treatment groups showed affinity to mature myostatin monomer in Western blot analysis, but none of the IgGs showed affinity to myostatin dimer. The IgGs of all the treatment groups failed to suppress the biological activity of myostatin in an A204 cell-based pGL3 (CAGA)12-luciferase reporter system, demonstrating that the increase in skeletal muscle mass observed in the offspring of the Myo2 group might not result from the inhibition of myostatin's biological activity by the anti-Myo2 antibodies. In conclusion, the results of this study suggest that antigen preparation may affect the responsiveness of skeletal muscle growth of offspring when maternal immunisation against myostatin is used as a potential tool to improve skeletal muscle growth of offspring.
Keywords:
Introduction
Myostatin, a member of the TGF-β superfamily of growth factors, is a potent negative regulator of skeletal muscle growth. Myostatin gene knockout demonstrated 2–3 times greater skeletal muscle mass in mice through muscle cell hyperplasia and hypertrophy (McPherron, Lawler, & Lee, Citation1997). A natural mutation in the myostatin gene resulted in double-muscled cattle breeds, such as Belgian Blue and Piedmontese (Grobet et al., Citation1997; Kambadur, Sharma, Smith, & Bass, Citation1997; McPherron & Lee, Citation1997). In humans, as in many other vertebrate species, a natural mutation in the myostatin gene induced a hypermuscular condition (Schuelke et al., Citation2004).
Mature myostatin protein, similar to many other TGF-β members, forms a homodimer that can bind to ActRIIB receptors located on muscle cells to exert its negative regulatory role on skeletal muscle growth (Lee et al., Citation2005; Lee & McPherron, Citation2001). Studies have indicated that myostatin activity is negatively regulated by interaction with various endogenous proteins, including myostatin propeptide, follistatin, follistatin-related gene (FLRG), growth and differentiation factor-associated serum protein-1 (GASP-1) and decorin (Amthor et al., Citation2004; Hill et al., Citation2002; Hill, Qiu, Hewick, & Wolfman, Citation2003; Lee & McPherron, Citation2001; Miura et al., Citation2006; Wolfman et al., Citation2003; Zimmers et al., Citation2002). Prevention of binding of mature myostatin to ActRIIB receptors by overexpression of propeptide (Lee & McPherron, Citation2001; Yang et al., Citation2001) or overexpression of follistatin (Lee & McPherron, Citation2001) or administration of soluble forms of ActRIIB receptors (Lee et al., Citation2005) resulted in a dramatic increase in skeletal muscle mass in mice. Anti-myostatin antibodies have also been tested as a vehicle to suppress the biological activity of myostatin (Bogdanovich et al., Citation2002; Whittemore et al., Citation2003). Administration of anti-myostatin antibody to adult mice induced hypertrophy of muscle fibre (Whittemore et al., Citation2003), and in dystrophic mice the administration of anti-myostatin antibody ameliorated muscular dystrophic conditions as evidenced by an increase in body mass and cross-sectional areas of whole muscle bundles and single fibres (Bogdanovich et al., Citation2002). In addition, we recently demonstrated that in-ovo administration of monoclonal anti-myostatin antibody enhanced post-hatch muscle mass in broilers (Kim, Bobbili, Paek, & Jin, Citation2006), proving that blocking myostatin activity is potentially an effective strategy to improve the growth of meat-producing animals.
As maternal antibodies are transferred to offspring during egg formation in birds (Kramer & Cho, Citation1970) and through the placenta or milk during neonatal suckling period or both in mammalian animals (Roberts, Guenthert, & Rodewald, Citation1990; Van de Perre, Citation2003), it was hypothesised that maternal immunisation against myostatin may be a feasible means of suppressing myostatin activity in offspring to enhance skeletal muscle growth. Therefore, the objective of this study was to examine whether maternal immunisation against myostatin in mice would affect postnatal growth and skeletal muscle mass of offspring.
Materials and methods
Antigen preparation
Three different antigens were prepared to immunise mice: an E. coli.-derived recombinant active form of myostatin (rMyo) and two peptides of the active form of myostatin. The production of recombinant myostatin has been described previously (Kim et al., Citation2006). The sequences of selected peptides were MSPINMLYFNQKEQIIYGKIPAMV and VFLQKYPHTHLVHQA, representing amino acid sequences from 79 to 102 (Myo1) and from 50 to 64 (Myo2) of the active form of myostatin, respectively. The two peptides were commercially synthesised and conjugated to keyhole limpet haemocyanin (KLH). A cysteine-glycine sequence was inserted at the N-terminal side of the peptides during synthesis to make a thio-ether linkage during the conjugation to carrier proteins. LC-SMCC (Pierce, Rockford, IL, USA), a sulphhydryl- and amine-reactive heterobifunctional cross-linking agent, was used for conjugation of peptides to the carrier protein.
Animals and immunisation
Animal care and immunisation were approved by the University of Hawaii Institutional Animal Care and Use Committee. Twenty female and four male (12-weeks-old, B6ELJ-6 strain) mice were purchased and housed with 12 h/12 h light-dark cycle. Animals had free access to commercial diet and water. Female mice were randomly divided into four groups, Con, rMyo, Myo1 and Myo2, with five mice in each group and housed together in a cage by groups. The Con group was immunised with 0.2 mg of KLH, the rMyo group with 0.2 mg of recombinant active form of myostatin, the Myo1 group with 0.2 mg of Myo1-KLH conjugate, and the Myo2 group with 0.2 mg of Myo2-KLH conjugate, subcutaneously below the tail and around the neck. Antigens were prepared in 50 µl of PBS and emulsified with the same amount of Titermax® adjuvant (Bio Scientific Pty, Sydney, Australia). Booster immunisations were administered 2–3 weeks apart, two to three times after the primary immunisation. Not all females showed significant titre after the second booster, thus some females received three boosters. Blood was collected by tail scission at each immunisation and boosters, stored at 4°C overnight and centrifuged at 3000×g for serum collection. Antibody titres were determined using ELISA.
Antibody titre
Indirect ELISA was used to detect antibody titres of sera. Wells were coated with either recombinant unprocessed porcine myostatin (rupMyo), Myo1 or Myo2 conjugated to BSA at a protein concentration of 100 ng/50 µl in PBS, pH 7.4. In our previous study, the rupMyo demonstrated binding to anti-myostatin antibodies (Jin, Dunn, Borthakur, & Kim, Citation2004). The plates were washed with PBS, and blocking solution (100 µl/well; 1% of BSA in PBS) was added to the plates. After incubation for 1 h at room temperature, the plates were washed twice with PBS. Duplicates of diluted antisera (50 µl/well) were allowed to react with the antigen for 1 h at room temperature. For non-specific binding, PBS (50 µl/well) as a blank was added. The plates were washed with PBS-0.05% Tween-20 three times. Anti-mouse IgG alkaline phosphatase conjugate in PBS-0.05% Tween-20 at 1:20,000 dilution was added and incubated for 1 h at room temperature. After washing three times with PBS-0.05% Tween-20, 50 µl of 4-nitrophenyl phosphate (pNPP) were added and incubated for 30 min in the dark at room temperature. The reaction was stopped by the addition of 25 µl of 3 N NaOH, and the absorbance was measured at 405 nm.
Breeding and postnatal sample collection
Antibody titres were monitored during booster immunisations to ensure that females were bred when significant titre values were present. When significant titre values were reached during booster immunisations, female mice were bred with four males with 3 days random rotation until signs of pregnancy. Pregnant mice were housed individually. At 3 days post-partum, one pup from each litter was sacrificed for serum collection to evaluate maternal transfer of antibody to offspring. Total litter weights from each female were recorded until the pups were weaned at 3 weeks of age. After weaning, male and female offspring were housed separately, and weekly body weights of male and female litters from each female were recorded separately until sacrifice at 8 weeks of age. Mice had free access to feed and water. At sacrifice, weights of carcass, selective muscles, and epididymal or parametrial fat were recorded. At the end of the experiment, dams were subjected to a final booster immunisation, and were sacrificed 2 weeks later for sera collection to examine the binding characteristics of the antibodies.
Western blot analysis
Sera collected from female dam mice at the end of the experiment were subjected to Protein A affinity chromatography (Affi-gel protein A MAPS® II kit, Bio-Rad, Hercules, CA, USA) to purify IgGs. Binding specificities of the IgGs to mature myostatin were determined using Western transfer and immunoblotting. Proteins fractionated by SDS-PAGE were electrophoretically transferred onto polyvinylidene fluoride (PVDF) membranes while immersed in Towbin buffer (25 mM Tris, 192 mM glycine, 20% methanol, 0.1% SDS). After transfer, membranes were blocked with BM® blocking solution (Roche Applied Science, Indianapolis, IN, USA) at 4°C overnight. Membranes were then incubated with 250 times diluted sera from the immunised female mice in TBS buffer (125 mM NaCl, 25 mM Tris, pH 8.0) containing 0.5% Tween-20 for 1 h at room temperature. After washing, membranes were incubated with alkaline phosphatase conjugated anti-mouse IgG (1:20,000 times dilution; Sigma, St. Louis, MO, USA) for 1 h at room temperature. After washing, membranes were developed using nitroblue tetrazolium and bromo-chloro-indolyl phosphate (BCIP/NBT) for 2–3 min.
Luciferase gene reporter assay
The capability of IgGs to inhibit myostatin activity was measured using the pGL3-(CAGA)12-luciferase reporter assay in A204 rhabdomyosarcoma cells (ATCC HTB-82) as described by Thies et al. (Citation2001). The pGL3-(CAGA)12 plasmid was constructed as described by Dennler et al. (Citation1998) and was a kind gift from Dr. Kunihiro Tsuchida at the University of Tokushima, Japan. A204 cells were plated in a 96-well plate at 40,000 cells/100 µl/well and grown in DMEM containing 10% fetal calf serum and penicillin-streptomycin plus fungizone at 37°C with 5% CO2. After 24 h, cells were transfected by adding a mixture of 0.2 µg of pGL3-(CAGA)12 plus 0.05 µg of pRL-TK (Promega, Madison, WI, USA) plasmid DNA and 0.5 µl of Lipofectamine 2000 solution (Invitrogen, Carlsbad, CA, USA) into each wells. At 24 h after transfection, medium was replaced with serum-free medium and incubated for 9 h, then various concentrations of IgG plus 5 ng/ml myostatin (R&D Systems, Minneapolis, MN, USA) were added into each well. After 6 h of incubation, luciferase activity was measured in Veritas microplate luminometer (Turner Biosystems Inc., CA, USA) using the Dual Luciferase Assay System (Promega) following the manufacturer's recommended procedure.
Statistical analysis
The weekly body weights of the offspring and carcass characteristics were analysed by the GLM procedure using JMP® software (SAS Institute, Cary, NC, USA). The model included main effects of treatment and sex and treatment–sex interaction. Tukey HSD test was used to compare the means in treatment groups when a significant effect was observed.
Results
Serum antibody titre and growth of immunised females
Titre values of immunised females before mating are summarised in . In our previous study (Jin et al. Citation2004), a recombinant unprocessed porcine myostatin (rupMyo) demonstrated a binding affinity to a commercially produced anti-myostatin antibody, thus we used the recombinant porcine myostatin as a coating antigen. While the sera from the control animals injected with a carrier protein (KLH) showed no detectable titre values, the sera of the three treatment groups showed titre values with the highest values in the Myo1 group. To characterise the cross-reactivity of the sera of the three groups, ELISA was also performed using Myo1 and Myo2 peptides conjugated to BSA as coating antigens (). The sera of the rMyo group had an affinity to both the Myo1 and Myo2 peptide, but the sera from the Myo1 group showed no affinity to the Myo2 peptide and vice versa.
Figure 1. Sera titres of immunised female mice before mating. Alkaline phosphatase conjugated anti-mice IgG and p-nitrophenyl phosphate (PNPP) were used in indirect ELISA, and recombinant unprocessed porcine myostatin was used as a coating antigen.
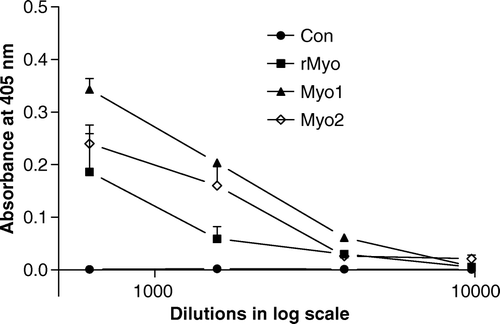
Table 1. Titre values of immunised female mice against three coating antigen.1
shows the weekly body weights of immunised female mice during the immunisation period. For 7 weeks during the immunisation regimen, no significant difference in body weight was observed among the treatment groups. It thus appears that immunisation against myostatin did not affect the body weight of immunised female mice over a 7-week period.
Table 2. Changes in body weights of immunised female mice following immunisation against myostatin antigens.
Maternal transfer of antibodies to offspring
Antibody titres of the offspring at 3 days post-partum are shown in . Significant titre values were detectable in Myo1, but the average titre values of the rMyo and Myo2 groups were not significantly different from that of the control group. Examination of the individual titre values of the offspring revealed that while titre values were observed from all the offspring of the Myo1 group, only one out of three offspring in the rMyo group and two out of four offspring in the Myo2 group showed detectable titre values in their sera. These results may indicate that not all offspring equally received anti-myostatin antibodies from their mothers.
Table 3. Titre values of newborn offspring1
Effects of maternal immunisation on body weight and muscle mass of offspring
The offspring were weaned on the third week post-partum and the littermates from each dam were separately housed according to their sex. Therefore, the entire litter from each female was weighed until they were weaned. After weaning, all males and females from each litter were weighed as a group but not individually until sacrifice at 8 weeks. shows the body weights of males and females up to 8 weeks of age. While significant sex effect was observed on body weight, no significant immunisation effect on body weight was observed until 7 weeks of age. At 8 weeks, offspring from the Myo2 group tended (p=0.056) to weigh heavier than the control group regardless of sex (21.42 vs. 20.44 g).
Table 4. Body weights of offspring of the immunised mice up to 8 weeks of age.
shows the carcass characteristics of the offspring at 8 weeks of age. Sex had significant effect on body, carcass, and muscle weights. Maternal immunisation did not affect the carcass weight and carcass percent of body weight. The offspring from the Myo2 group had significantly heavier gastrocnemius complex, triceps and fat mass than the offspring from other groups. Percentages of gastrocnemius complex and triceps to body weight of the offspring from the Myo2 group were also higher compared to those of other groups.
Table 5. Carcass, fat and individual muscle weights of offspring at sacrifice at 8 weeks.
Binding of serum IgGs to myostatin
The sera from each group of female dam mice were pooled, and IgGs were purified using protein A affinity chromatography. The binding affinity of anti-myostatin antibodies to the monomer and dimer forms of mature myostatin (R&D Systems) was examined by Western blot analysis (). IgGs from the three treatment groups showed affinity to myostatin monomer (12.5 kDa), but none of the IgGs from the three treatment groups showed affinity to the dimer form of myostatin (24 kDa).
Figure 2. Western blot analysis of binding affinity of purified IgG to myostatin. Molecular wt standard (lane 1), 200 ng of mature myostatin (R&D Systems) in non-reduced (lane 2), reduced (lane 3) condition, 4 µg of leg muscle (lane 4) and liver homogenate (lane 5) in reduced condition were fractionated on 15% SDS-PAGE and visualised with Coomassie blue stain (a) or electrophoretically transferred onto a PVDF membrane. The membranes were incubated with 2 µg/ml affinity purified IgG from control (b), rMyo (c), Myo1 (d) and Myo2 (e). Secondary anti-rabbit IgG conjugated to alkaline phosphatase was added at 1:10,000 dilution. Upper and lower arrows indicate myostatin dimer and monomer, respectively. The big protein bands above 50 kDa in lanes 2 and 3 of SDS-PAGE are BSA added as a carrier protein during myostatin preparation.
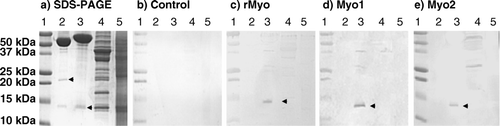
Effects of purified IgG on myostatin activity in pGL3 (CAGA)12-luciferase reporter system
The ability of affinity purified IgG to inhibit the biological activity of myostatin was examined using pGL3-(CAGA)12-luciferase reporter assay system in which various concentrations of IgGs plus 5 ng/ml myostatin were added to A204 cells transfected with the pGL3-(CAGA)12 plasmid. As shown in the , the reporter assay system was responsive to 5 ng/ml of recombinant mature myostatin. At the same time, the myostatin activity was completely inhibited by 30 ng/ml of myostatin propeptide (R&D Systems), illustrating the robustness of the system to test the signal transduction of myostatin. However, none of the IgGs from the treatment groups reduced the luciferase activity (), indicating that none of the antibodies from the treatment groups were able to modulate the biological activity of myostatin. The assay was repeated and showed similar results.
Figure 3. Effects of affinity-purified IgG on myostatin's activity in pGL3 (CAGA)12-luciferase reporter system. A204 cells were seeded (40,000 cells/100 µl/well) on a 96-well culture plate and grown in DMEM with 10% fetal calf serum, antibiotic and antimycotic. After 36 h, a transfection mixture was added containing 0.2 µg of pGL3 (CAGA)12 luc-luciferase plasmid, 0.05 µg of pRL-TK-Renilla luciferase plasmid and 0.5 µl of lipofectamine 2000 in antibiotic-free DMEM containing 10% FCS. After 24 h of transfection, the cells were serum starved for 9 h. After serum starvation, myostatin (5 ng/ml) and various dilutions of the affinity-purified IgGs were added to each well in quadruplets followed by incubation for 6–8 h. Luciferase activity was measured by Veritas microplate luminometer (Turner Biosystems Inc.) using Dual Luciferase Assay System (Promega). Figures A–D represent the ratios of firefly luciferase to renilla luciferase in affinity-purified IgG treated wells from control, rMyo, Myo-1 and Myo-2, respectively. Positive controls were maintained on 5 ng/ml myostatin and 30 ng/ml propeptide while negative controls were on 5 ng/ml myostatin, without propeptide.
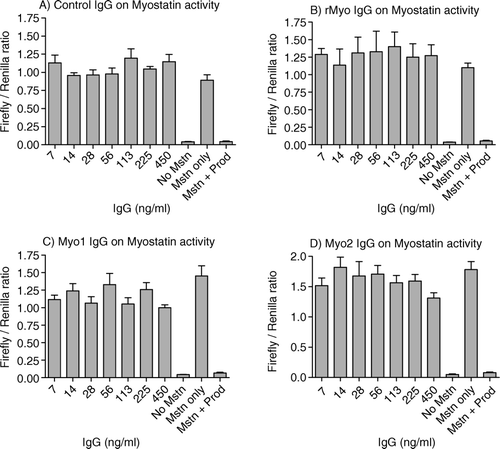
Discussion
Studies have shown that anti-myostatin antibodies are able to inhibit myostatin activity, as demonstrated by the increased muscle mass in mice when administered postnatally (Bogdanovich et al., Citation2002; Whittemore et al., Citation2003). Recently, we also observed that posthatch body mass and skeletal muscle growth of broilers can be significantly improved by in-ovo administration of monoclonal anti-myostatin antibodies into the yolk at 3 days incubation (Kim et al., Citation2006), suggesting that immunoneutralisation of myostatin is a potential means to improve the growth of meat-producing animals. As an alternate to passive immunisation against myostatin, we investigated in this study the effects of active immunisation against myostatin in female mice on body and skeletal muscle growth of their offspring. As active immunisation confers maternal transfer of antibodies to the offspring through placenta and colostrums in mice (Roberts et al., Citation1990; Van de Perre, Citation2003), we hypothesised that maternal immunisation against myostatin may enhance the skeletal muscle mass in offspring.
In this study, we used three different myostatin immunogens: rMyo, a recombinant, denatured mature myostatin protein, and two KLH conjugated myostatin peptides (Myo1 and Myo2). In selecting the Myo1 and Myo2 peptides, a predicted three-dimensional (3-D) structure of myostatin () was constructed using the SWISS-MODEL Repository (Peitsch, Citation1997) hosted by the Swiss Institute of Bioinformatics. As expected, the predicted 3-D structure of myostatin was similar to those of other known TGF-β family member proteins, such as TGF-β2 (Daopin, Li, & Davies, Citation1993; Griffith, Keck, Sampath, Rueger, & Carlson, Citation1996). The 3-D structure of the TGF-β family member proteins were shown to contain two finger regions and one heel region with the core being constituted by the cysteine knot that is formed by four disulphide bonds (Daopin et al., Citation1993; Griffith et al., Citation1996). The heel region is known to be involved in the dimer formation of TGF-β family proteins, and the tips of finger regions are known to be involved in receptor binding (Souchelnytskyi, Moustakas, & Heldin, Citation2002). According to Flanders, Roberts, Ling, Fleurdelys, and Sporn (Citation1988), antibodies generated against the C-terminal region of TGF-β1 were effective in blocking the binding of TGF-β1 to its receptor, while antibodies generated against the N-terminal region of TGF-β1 showed no blocking effect, illustrating the importance of peptide selection in generating antibodies against growth factors capable of inhibiting receptor binding. Assuming myostatin probably has similar antigenic characteristics, we selected two peptide sequences (Myo1 and Myo2) from the C-terminal region of myostatin.
Figure 4. Location of Myo1 and Myo2 peptides used as antigens in predicted 3-D model of myostatin. The model is generated using the SWISS-MODEL Repository (Peitsch, Citation1997).
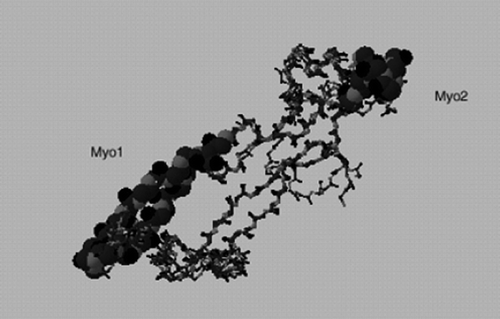
Sera titre measurements confirmed significant antibody levels in the immunised female mice. Sera of the Myo1 and Myo2 groups showed higher affinities to their respective coating antigens than to unprocessed, recombinant myostatin (rupMyo) coating antigen. There was no significant cross-reactivity of Myo1 sera to Myo2 coating antigen or Myo2 sera to Myo1 coating antigen. The rMyo serum showed an affinity to both Myo1 and Myo2 coating antigens, indicating that immunisation with mature myostatin protein (rMyo) confers antibodies against both Myo1 and Myo2 peptide fragments as well. This result is not surprising because rMyo immunogen contains both the Myo1 and Myo2 sequences. Maternal transfer of antibodies was evident in 3-day-old offspring in all three treatment groups, even though the transfer was variable in offspring from the rMyo and Myo2 groups. It is conceivable that the variability of maternal transfer of antibodies to offspring could be due to variations in titre levels of females or the variable amount of colostrum suckled by newborns or both. In a similar study, Liang, Yeh and Ou (Citation2007) recently reported the presence of anti-myostatin antibodies as long as 8 weeks post-partum in the offspring of females immunised against a myostatin peptide before pregnancy.
Despite the evident maternal transfer of anti-myostatin antibodies to the offspring, no significant effects of immunisation on the body weights of offspring were observed in the rMyo and Myo1 groups throughout the 8-week growth period except that the body weights of offspring of the Myo2 group tended to be heavier than that of the control. Interestingly, gastrocnemius and triceps muscle of the Myo2 offspring were significantly heavier compared to those of the control group, while those of the rMyo and Myo1 offspring were not. The results of Liang et al. (Citation2007) showed that maternal immunisation against a myostatin peptide in mice enhanced growth rate and body crude protein contents and decreased body fat content of offspring. When we compared the peptide sequence of their immunogen with the sequence of Myo2, we noticed that a significant portion of their peptide antigen overlapped with the Myo2. The sequence of Liang's antigen was ECEFVFLQKYP and the Myo-2 sequence was VFLQKYPHTHLVHQA, revealing seven amino acids overlapping between the two peptide antigens. It was thus suspected that the binding characteristics and capacity to suppress myostatin activity of the IgGs of the Myo2 might be different from those of the rMyo or Myo1. Our examination of the capacity to suppress the myostatin activity using the pGL3 (CAGA)12-luciferase reporter system demonstrated that IgGs of the Myo2 group and those of the rMyo and Myo1 could not suppress myostatin activity. Furthermore, in Western blot analyses, the purified IgGs from all treatment groups (rMyo, Myo-1 and Myo-2) showed no affinity to the dimer form of mature myostatin even though all of the IgGs showed affinity to the monomer form of myostatin. Considering that the homodimer of mature myostatin protein binds to ActRIIB receptors to exent its signal transduction process and resultant regulation of skeletal muscle mass (Lee & McPherron, Citation2001), the inability of the antibodies to bind the dimer form of myostatin is probably related to the inability of the antibodies to suppress the myostatin activity. In support of the above contention, in-ovo administration of a monoclonal anti-myostatin antibody having affinity to the myostatin dimer resulted in enhanced posthatch body mass and skeletal muscle growth in broilers (Kim et al., Citation2006). The current results thus indicate that the antigens used in this study could not induce the production of antibodies capable of binding to the homodimer to suppress myostatin activity. Therefore, the increased muscle growth observed in the Myo2 offspring was unlikely due to the suppression of myostatin activity via anti-Myo2 antibodies.
Currently, we have no explanation for the potential mechanisms leading to the increased muscle growth observed in the offspring of females immunised against Myo2. While the results of Western blot and pGL3 (CAGA)12-luciferase reporter assay suggest that myostatin inhibition by the anti-myostatin antibody is not the plausible mechanism, that the anti-Myo2 antibodies modulates other biochemical pathways leading to increased muscle growth cannot be ruled out. In conclusion, the results of this study suggest that antigen preparation may affect the responsiveness of skeletal muscle growth offspring when maternal immunisation against myostatin is used as a potential tool to improve skeletal muscle growth of offspring. Future studies probably need to identify myostatin epitope(s) that can produce potent anti-myostatin antibodies capable of binding myostatin dimer to suppress myostatin activity.
Acknowledgements
This study was supported by USDA-TSTAR grant (2004-34135-15196).
References
- Amthor , H. , Nicholas , G. , McKinnell , I. , Kemp , C.F. , Sharma , M. Kambadur , R. 2004 . Follistatin complexes myostatin and antagonises myostatin-mediated inhibition of myogenesis . Developmental Biology , 270 : 19 – 30 .
- Bogdanovich , S. , Krag , T.O. , Barton , E.R. , Morris , L.D. , Whittemore , L.A. Ahima , R.S. 2002 . Functional improvement of dystrophic muscle by myostatin blockade . Nature , 420 : 418 – 421 .
- Daopin , S. , Li , M. and Davies , D.R. 1993 . Crystal structure of TGF-beta 2 refined at 1.8 A resolution . Proteins , 17 : 176 – 192 .
- Dennler , S. , Itoh , S. , Vivien , D. , Ten , D.P. , Huet , S. and Gauthier , J.M. 1998 . Direct binding of Smad3 and Smad4 to critical TGF beta-inducible elements in the promoter of human plasminogen activator inhibitor-type 1 gene . The EMBO Journal , 17 : 3091 – 3100 .
- Flanders , K.C. , Roberts , A.B. , Ling , N. , Fleurdelys , B.E. and Sporn , M.B. 1988 . Antibodies to peptide determinants in transforming growth factor beta and their applications . Biochemistry , 27 : 739 – 746 .
- Griffith , D.L. , Keck , P.C. , Sampath , T.K. , Rueger , D.C. and Carlson , W.D. 1996 . Three-dimensional structure of recombinant human osteogenic protein 1: structural paradigm for the transforming growth factor beta superfamily . Proceedings of the National Academy of Sciences of the United States of America , 93 : 878 – 883 .
- Grobet , L. , Martin , L.J. , Poncelet , D. , Pirottin , D. , Brouwers , B. Riquet , J. 1997 . A deletion in the bovine myostatin gene causes the double-muscled phenotype in cattle . Nature Genetics , 17 : 71 – 74 .
- Hill , J.J. , Davies , M.V. , Pearson , A.A. , Wang , J.H. , Hewick , R.M. , Wolfman , N.M. and Qiu , Y. 2002 . The myostatin propeptide and the follistatin-related gene are inhibitory binding proteins of myostatin in normal serum . The Journal of Biological Chemistry , 277 : 40735 – 40741 .
- Hill , J.J. , Qiu , Y. , Hewick , R.M. and Wolfman , N.M. 2003 . Regulation of myostatin in vivo by growth and differentiation factor-associated serum protein-1: a novel protein with protease inhibitor and follistatin domains . Journal of Molecular Endocrinology , 17 : 1144 – 1154 .
- Jin , H.J. , Dunn , M.A. , Borthakur , D. and Kim , Y.S. 2004 . Refolding and purification of unprocessed porcine myostatin expressed in Escherichia coli . Protein Expression and Purification , 35 : 1 – 10 .
- Kambadur , R. , Sharma , M. , Smith , T.P. and Bass , J.J. 1997 . Mutations in myostatin (GDF8) in double-muscled Belgian Blue and Piedmontese cattle . Genome Research , 7 : 910 – 916 .
- Kim , Y.S. , Bobbili , N.K. , Paek , K.S. and Jin , H.J. 2006 . Production of a monoclonal anti-myostatin antibody and the effects of in ovo administration of the antibody on posthatch broiler growth and muscle mass . Poultry Science , 85 : 1062 – 1071 .
- Kramer , T.T. and Cho , H.C. 1970 . Transfer of immunoglobulins and antibodies in the hen's egg . Immunology , 19 : 157 – 167 .
- Lee , S.J. and McPherron , A.C. 2001 . Regulation of myostatin activity and muscle growth . Proceedings of the National Academy of Sciences of the United States of America , 98 : 9306 – 9311 .
- Lee , S.J. , Reed , L.A. , Davies , M.V. , Girgenrath , S. , Goad , M.E. Tomkinson , K.N. 2005 . Regulation of muscle growth by multiple ligands signaling through activin type II receptors . Proceedings of the National Academy of Sciences of the United States of America , 102 : 18117 – 18122 .
- Liang , Y.C. , Yeh , J.Y. and Ou , B.R. 2007 . Effect of maternal myostatin antibody on offspring growth performance and body composition in mice . Journal of Experimental Biology , 210 : 477 – 483 .
- McPherron , A.C. and Lee , S.J. 1997 . Double muscling in cattle due to mutations in the myostatin gene . Proceedings of the National Academy of Sciences of the United States of America , 94 : 12457 – 12461 .
- McPherron , A.C. , Lawler , A.M. and Lee , S.J. 1997 . Regulation of skeletal muscle mass in mice by a new TGF-beta superfamily member . Nature , 387 : 83 – 90 .
- Miura , T. , Kishioka , Y. , Wakamatsu , J. , Hattori , A. , Hennebry , A. Berry , C.J. 2006 . Decorin binds myostatin and modulates its activity to muscle cells . Biochemical and Biophysical Research Communications , 340 : 675 – 680 .
- Peitsch , M.C. 1997 . “ Large scale protein modeling and model repository ” . In Proceedings of the fifth international conference on intelligent systems for molecular biology , Edited by: Gaasterland , T. , Karp , P. , Karplus , K. , Ouzounis , C. , Sander , C. and Valencia , A. Vol. 5 , 234 – 236 . Menlo Park : AAAI Press .
- Roberts , D.M. , Guenthert , M. and Rodewald , R. 1990 . Isolation and characterization of the Fc receptor from the fetal yolk sac of the rat . Journal of Cell Biology , 111 : 1867 – 1876 .
- Schuelke , M. , Wagner , K.R. , Stolz , L.E. , Hubner , C. , Riebel , T. Komen , W. 2004 . Myostatin mutation associated with gross muscle hypertrophy in a child . The New England Journal of Medicine , 350 : 2682 – 2688 .
- Souchelnytskyi , S. , Moustakas , A. and Heldin , C.H. 2002 . TGF-beta signaling from a three-dimensional perspective: insight into selection of partners . Trends in Cell Biology , 12 : 304 – 307 .
- Thies , R.S. , Chen , T. , Davies , M.V. , Tomkinson , K.N. , Pearson , A.A. Shakey , Q.A. 2001 . GDF-8 propeptide binds to GDF-8 and antagonizes biological activity by inhibiting GDF-8 receptor binding . Growth Factors , 18 : 251 – 259 .
- Van de Perre , P. 2003 . Transfer of antibody via mother's milk . Vaccine , 21 : 3374 – 3376 .
- Whittemore , L.A. , Song , K. , Li , X. , Aghajanian , J. , Davies , M. Girgenrath , S. 2003 . Inhibition of myostatin in adult mice increases skeletal muscle mass and strength . Biochemical and Biophysical Research Communications , 300 : 965 – 971 .
- Wolfman , N.M. , McPherron , A.C. , Pappano , W.N. , Davies , M.V. , Song , K. Tomkinson , K.N. 2003 . Activation of latent myostatin by the BMP-1/tolloid family of metalloproteinases . Proceedings of the National Academy of Sciences of the United States of America , 100 : 15842 – 15846 .
- Yang , J. , Ratovitski , T. , Brady , J.P. , Solomon , M.B. , Wells , K.D. and Wall , R.J. 2001 . Expression of myostatin pro domain results in muscular transgenic mice . Molecular Reproduction and Development , 60 : 351 – 361 .
- Zimmers , T.A. , Davies , M.V. , Koniaris , L.G. , Haynes , P. , Esquela , A.F. Tomkinson , K.N. 2002 . Induction of cachexia in mice by systemically administered myostatin . Science , 296 : 1486 – 1488 .