Abstract
Enzyme-linked immunosorbent assay (ELISA) kits (indirect competitive and sandwich formats) to determine either β-lactoglobulin or ovomucoid were evaluated in model foods. A cut-off value was established for each kit to consider food samples as positive for milk or egg addition. Sausage and bread were positive at lower percentages of added milk using the sandwich format (0.005 and 0.05%) than the indirect competitive format (0.05 and 0.25%) and pâté was positive at 0.25% milk addition for both formats. Sausage was positive at 0.005%, and bread at 0.05% added egg for indirect competitive and sandwich formats, whereas pâté was positive at 0.25% egg only by the indirect competitive assay. The concentration of added milk and egg to give a positive result depends on heat treatment, being higher for pâté (sterilised), followed by bread (baked) and sausage (pasteurised). The particularities of each format and the heat processing applied influenced the determination by ELISA of allergenic proteins in foods.
Introduction
Food allergies represent an important health problem in industrialised countries. Although accurate statistics are unavailable, data indicate that this health issue is on the rise (Yeung, Citation2006). Many studies have shown that egg and milk allergies are the most prevalent allergies in children. In studies performed in children under 2 years of age, milk allergy represents about 2–4% and egg allergy about 3–4% (Poms, Klein, & Anklam, 2004). Although milk and egg allergies usually disappear during the first 3 years of age, some individuals continue having severe reactions to those foods in adulthood.
The most allergenic proteins in eggs are present in the white, being ovomucoid (Gal d 1), the most important allergen followed by ovalbumin (Gal d 2), ovotransferrin (Gal d 3), and lysozyme (Gal d 4). In the case of milk, the proteins that cause allergic reactions include both caseins (Bos d 8) and whey proteins, mainly β-lactoglobulin (Bos d 5) and α-lactalbumin (Bos d 4) (Bush & Hefle, Citation1996). Most of these proteins retain their allergenicity after heating or other processing (Besler, Steinhart, & Paschke, Citation2001; Monaci, Tregoat, van Hengel, & Anklam, Citation2006).
The prevention of food allergies primarily involves strict avoidance of the offending foods. This means that food processors should implement an allergen prevention plan and inform consumers about the presence of allergens in their products (Deibel et al., Citation1997). European Legislation, such as Directive 2003/89/EC and amendments (European Commission, Citation2003) requires that all ingredients added to food products have to be labelled and demands the obligatory declaration of those liable to cause allergies or intolerances in which milk and egg and products thereof are included. Furthermore, the inadvertent presence of allergens results from practices in the food industry such as the use of contaminated raw materials, inadequate cleaning of shared equipment or other sources of cross-contact (Hefle & Lambrecht, Citation2004). Therefore, reliable detection methods for food allergens are necessary to ensure its compliance with the food labelling and to improve consumer protection.
In the last few years, several immunochemical techniques have been developed to determine milk and egg proteins in food products. These techniques are mainly based on the interaction between the allergenic protein and the specific antibodies obtained against it. At present, only the ELISA technique is used in the routine analysis due to its high precision, simple handling and good potential for standardisation (de Luis, Pérez, Sánchez, Lavilla, & Calvo, Citation2007; Hefle & Lambrecht, Citation2004; Mariager, Solve, Eriksen, & Brogren, Citation1994; Poms et al., Citation2004).
According to studies performed by clinicians, it is considered that the detection limit of techniques to detect allergenic sources should be in the range between 1 and 10 ppm, so most commercial immunoassays have detection limits within this range (Poms et al., Citation2004; van Hengel, Citation2007).
However, the determination of allergenic proteins using immunoassays has some limitations related to the extraction of the target protein from the food and to interferences by other components of the matrix. Food processing also poses a great challenge with respect to allergen detection by immunoassays because it leads to partial denaturation of proteins which can affect the ability of antibodies to recognise them (de Luis et al., 2007; Julià et al., Citation2007). Simple spiking of foods with extracts of allergenic ingredients is not considered appropriate in assessing the performance of allergen detection techniques. Therefore, making model foods according to pilot plant or true industrial conditions will be the key in the evaluation of allergen detection methods until well-characterised reference materials are available (Poms, Citation2006).
On the other hand, the effectiveness of an immunoassay depends directly on the antigen selected as a target and the quality of the antibodies used but in addition to this the performance of the assay itself is also important (de Luis et al., 2007; Immer, Citation2006).
In this work, four ELISA tests based on the detection of β-lactoglobulin or ovomucoid (an indirect competitive format and a double antibody sandwich format for each protein) have been evaluated by three participating laboratories. Samples analysed were three model processed foods elaborated at a pilot plant, which contained different amounts of skimmed milk powder and egg powder.
Materials and methods
Materials
Low heat skimmed milk powder was supplied by Reny Picot (Anelo, Spain), egg powder by Huevos Mayper S.A. (Valladolid, Spain), the mixture of spices and the soya protein isolate by Anvisa (Arganda del Rey, Madrid, Spain), pea proteins by Cosucra (Fontenoy, Belgium) and soluble wheat proteins by Tate & Lyle (Zaragoza, Spain). Bovine serum albumin was from Sigma Chemical (Poole, UK). The rest of the ingredients were purchased from local retailers. ELISA kits (Proteon β-lactoglobulin indirect competitive and sandwich kits and Proteon ovomucoid indirect competitive and sandwich kits) were provided by ZEU-Inmunotec (Zaragoza, Spain).
Preparation of model processed foods
Three model processed foods (sausage, bread and pâté) containing low heat skimmed milk powder and egg powder as allergenic ingredients were prepared at the Pilot Plant of Food Science and Technology (University of Zaragoza). They were prepared following standard manufacturing processes. The allergenic ingredients were spiked at the ingredient stage before processing to obtain final concentrations of 0.25%, 0.5% and 1% (w/w).
Sausage was made of 5 kg of pork leg meat, 1 kg of pork fat, 450 g of a mixture of spices, 900 g of starch and 3 kg of ice water. The ingredients were mixed thoroughly and the mixture ground using a cutter. Then, different amounts of skimmed milk powder and egg powder were added and the mixture kneaded using a homogeniser. The mixture was stuffed in cellulose casings (2.8 cm diameter), placed into the oven and cooked to 75°C (internal temperature). Sausages were vacuum packed and heated at 90°C (internal temperature) for 1 min in a thermostatic water bath.
Bread was made using a bread and dough maker (Deluxe: Bread and Dough Maker, Oster, USA). An amount of 480 g of wheat flour, 10 g of margarine, 20 g of sugar, 5 g of salt, 8.8 g of yeast, skimmed milk powder and egg powder and 205 ml of water, were kneaded for 30 min and left for 1 h at room temperature for dough formation. Then, the dough was baked at 95°C (internal temperature) for 40 min.
Pâté was prepared with 1.6 kg of pork liver, 2.5 kg of pork fat, 100 g of salt, 11 g of pepper, 2.8 g of sugar, 166 g of maize flour, 166 g of margarine and 840 ml of water. Pork fat was grounded, heated in water at 85°C for 30 min and then, drained to remove water. The pork fat, the ground pork liver and the rest of ingredients were mixed thoroughly. Then, different amounts of skim milk powder and egg powder were added and the paste homogenised. A 250 g sample of the mixture was packaged in glass jars and heated at 120°C (internal temperature) for 1 h in an autoclave.
Food samples were ground and weighed into 50 ml plastic centrifugation tubes (3.00±0.01 g). To obtain model foods with final concentrations of 0.005%, 0.01%, 0.05% and 0.1%, of milk and egg powder, certain amounts of the finely ground 0.25% samples were weighed directly into centrifugation tubes containing the corresponding blank sample to give a total weight of 3.00±0.01 g. All tubes were closed and stored at –20°C before dispatching to the participants.
Extraction of test samples
A 3.00±0.01 g amount of ground sample was homogenised in 30 ml of extraction buffer composed by 0.15M NaCl, 0.0015M potassium phosphate buffer, pH 7.4 (PBS) for 1 min using a vortex mixer, and then heated at 60°C for 15 min in a thermostatic water bath. Extracts were clarified by centrifugation at 3000×g for 15 min, the supernatant was filtered through paper and analysed.
ELISA test kits
ELISA kits contained coated plates, extraction and washing buffers, standard solutions, antibody-enzyme conjugates, enzyme substrate and stopping solution. ELISA kits targeted either bovine β-lactoglobulin or hen's ovomucoid.
In the indirect competitive formats, antisera to β-lactoglobulin or ovomucoid raised in rabbits were used. In the double antibody sandwich formats, specific antibodies purified from their respective antisera using an immunosorbent of β-lactoglobulin or ovomucoid were used. Protein standards contained purified proteins diluted in PBS and the concentration was determined by spectrophotometry using an extinction coefficient at 280 nm of E1%=9.6 for β-lactoglobulin and E1%=4.6 for ovomucoid.
For the sandwich format, a volume of 100 µl per well of standard or sample solutions was added to a plate coated with antibodies against β-lactoglobulin or ovomucoid, and incubated for 30 min at room temperature. The plate was washed five times with 300 µl/well of washing buffer composed by PBS containing 0.05% Tween (PBST) and then, 100 µl/ well of a solution of peroxidase labelled antibodies was added and incubated for 30 min at room temperature. The plate was washed again and 100 µl/well of a solution of 3, 3’, 5, 5’-tetramethylbenzidine (TMB) was added and incubated for 30 min at room temperature. The reaction was stopped by adding 50 µl/well of stopping solution containing 1M sulphuric acid and the absorbance of wells was measured at 450 nm.
For the indirect competitive formats, 100 µl of standard or sample solutions and 100 µl of rabbit antiserum against β-lactoglobulin or ovomucoid were added to each well of a plate coated with β-lactoglobulin or ovomucoid, respectively, and incubated for 15 min at room temperature. The plate was washed five times with 300 µl/well of washing buffer and then incubated with 100 µl/well of anti-rabbit IgG antibodies labeled with peroxidase for 15 min at room temperature. After washing the plate, 100 µl/well of the TMB substrate was added and incubated for 15 min at room temperature. The reaction was stopped by adding 50 µl/well of stopping solution and the absorbance of wells was measured at 450 nm.
Evaluation study
Three laboratories participated in this study coordinated by the University of Zaragoza. Four trials, one for each protein (β-lactoglobulin and ovomucoid) and format (indirect competitive and sandwich) were performed. The coordinator provided the 24 pre-weighed test samples and ZEU-Inmunotec provided the four ELISA kits with instructions.
The calibration standard solutions were assayed simultaneously with the food extracts. Raw experimental absorbance data of calibration standards and test samples were sent to the coordinator. The average absorbance of duplicate wells was used for the calculation. Calibration curves were obtained using the relationship between the value of absorbance and the logarithm of the concentration of standard solutions for β-lactoglobulin indirect competitive test and ovomucoid indirect competitive and sandwich tests. For β-lactoglobulin sandwich test, calibration curves obtained used the relationship between the value of absorbance and the concentration of standard solutions (a–d). The concentration of β-lactoglobulin and ovomucoid in the test samples was calculated using the correspondent calibration curves.
Results and discussion
Single laboratory validation
A single laboratory validation of ELISA tests was performed to determine the limit of detection (LOD), the homogeneity of the food samples and the specificity of the tests. The LODs were calculated as the mean concentration value of eight replicates of the blank standard plus three times the value of its standard deviation (). The homogeneity of the final food samples was tested using the indirect competitive and sandwich formats tests for β-lactoglobulin and ovomucoid. For this purpose, five samples of each concentration and matrix were analysed in duplicate. The variation coefficient was less than 20%, which was considered acceptable for the purpose of the study.
Table 1. Limit of detection (LOD) and cut-off established for the ELISA tests to determine β-lactoglobulin or ovomucoid in foods. Calibration points correspond to the protein concentration of standards used in each ELISA test.
To verify the specificity of the ELISA tests, 15 basic ingredients were analysed. Most ingredients showed a small decrease or increase of the background level compared to the blank, for the indirect competitive and sandwich formats, respectively, indicating certain interference ( a–d). In order to overcome this problem, a cut-off value was established for each test. The cut-off value was calculated as the mean concentration value of ingredients assayed plus three times the value of its standard deviation. Therefore, food samples giving a concentration value equal or higher than the cut-off established for each test were considered as positive for milk or egg addition. The cut-off values established for each ELISA test and protein are shown in . The assumption of these cut-off values assured that the effect of potential cross-reactivity of a food ingredient in the ELISA tests is minimized. All ingredients used in the elaboration of model foods were assayed to know cross-reactivity and concentrations determined were lower than the cut-off established for each ELISA format (results were not shown).
Figure 2. Cross reactivity of basic ingredients in the double antibody sandwich (a and c) and indirect competitive (b and d) ELISA test kits for β-lactoglobulin (a and b) and ovomucoid (b and d). 1: Ovalbumin, 2: lysozyme, 3: bovine serum albumin, 4: meat proteins, 5: fish gelatine, 6: soluble wheat proteins, 7: wheat flour, 8: maize flour, 9: rice flour, 10: olive oil, 11: soya protein isolate, 12: pea proteins, 13: milk, 14: whey, 15: casein. Data are the mean values of two sample extractions assayed by duplicate and are expressed in ppm.
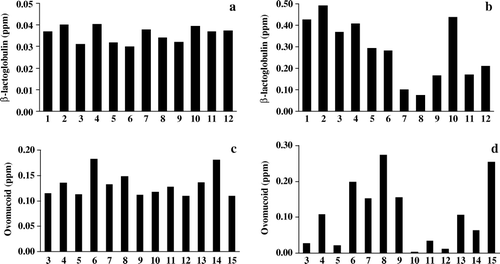
The concentration of β-lactoglobulin in skimmed milk powder was found to be 28.5 and 114.1 mg/g and the concentration of ovomucoid in egg powder of 21.0 and 32.1 mg/g for the sandwich and indirect competitive assays, respectively. These different results when using the two formats for the same protein could be attributed to differences in protein recognition by the antibodies used in each assay.
Evaluation of ELISA kits
Four trials, one for each protein and format, were performed by the three laboratories. Data obtained from the four assays for the determination of β-lactoglobulin and ovomucoid in food samples by the three laboratories were processed as described above.
Calibration curves were obtained for every ELISA plate using the protein standards indicated in . When using those standards, a linear relationship was obtained between absorbance values and protein concentration, with square coefficients of correlation ≥0.98 in all cases ( a–d). The concentration of β-lactoglobulin and ovomucoid in test samples was calculated on the basis of the calibration curve for each plate. Individual results obtained in the trials performed for the quantitation of β-lactoglobulin and ovomucoid in model foods are presented in and , respectively, and the mean values and coefficients of variation are summarised in .
Table 2. Results obtained by the three participating laboratories for the determination of β-lactoglobulin (ppm) in model processed foods added with different percentages of skimmed milk powder, using the double antibody sandwich and indirect competitive ELISA formats.
Table 3. Results obtained by the three participating laboratories for the determination of ovomucoid (ppm) in model processed foods added with different percentages of egg powder, using the double antibody sandwich and indirect competitive ELISA formats.
Results obtained by the three participants for both indirect competitive and sandwich tests showed that for the three model foods analysed, the blank food samples, without milk and egg powder added, gave concentrations of β-lactoglobulin and ovomucoid below the cut-off established for each ELISA test and, therefore, no false-positive samples were found in this work.
For the food samples, it was found that the amount of added milk and egg powder required to give a positive result was dependent on the intensity of heat treatment applied, being that amount higher for pâté (sterilised), followed by bread (baked) and sausage (pasteurized). Furthermore, for the same percentage of milk and egg powder added to samples considered as positive, β-lactoglobulin and ovomucoid estimated concentrations were higher in sausage, followed by bread and pâté when analysing by the two ELISA formats. This fact is probably due in great part to the heat processing which causes protein denaturation and aggregation and consequently a decrease in the degree of immunoreactivity depending on the treatment applied. This could also explain the low values of β-lactoglobulin and ovomucoid concentration in processed foods, which were much lower than those estimated from milk and egg powder added as ingredients.
Results obtained in this work indicate that the quantitative determination of β-lactoglobulin and ovomucoid, in processed foods depends on the ELISA format used. For the sandwich format, samples were found to be positive for β-lactoglobulin by the three laboratories at percentages of added milk of 0.005 and 0.05%, for sausage and bread, respectively, whereas for the indirect competitive format, samples were positive at percentages of added milk of 0.05 and 0.25% for the same model foods. In the case of the pâté samples, both formats gave positive results at 0.25% of milk addition ().
In the case of the ovomucoid assays, samples of sausage were positive for the three laboratories at 0.005% of added egg and samples of bread at 0.05% of added egg for both formats. For the pâté samples, only the indirect competitive assay could detect ovomucoid powder at 0.25% of egg addition ().
For the food samples with lower percentages of milk and egg addition than those indicated above, the concentrations of β-lactoglobulin and ovomucoid were found to be below the cut-off established for each ELISA format resulting in negatives, unless for bread containing 0.1% of milk powder which was positive only for laboratory 3 by the indirect competitive assay.
At higher percentages of milk and egg powder added than those indicated above, samples were positive with the exception of the 0.1% bread sample analysed using sandwich format for ovomucoid which was negative only for laboratory 2. The concentration of immunoreactive β-lactoglobulin or ovomucoid in positive samples increased gradually with the amount of added milk and egg powder. However, for sausage samples analysed by the sandwich ELISA formats, the concentration of these proteins did not increase at percentages of milk or egg powder higher than 0.25% that possibly due to a saturation effect, in spite of the fact that the calibration curve of ovomucoid was linear up to a standard concentration of 5 ppm.
The coefficients of variation for sausage and bread samples were in most cases higher for the indirect competitive than for the sandwich formats (). This higher variability may be attributed to the higher slope of the calibration curve obtained in the formats. Thus, for a certain change in the absorbance values, the variation of the concentration values is higher in the indirect competitive than in the sandwich format. For the pâté samples, the variation coefficients were very high in most samples probably due to matrix interferences produced by the high fat content of this food product.
Table 4. Mean values () (mg/kg) and coefficients of variation (CV) obtained by the participating laboratories for the determination of β-lactoglobulin and ovomucoid in model processed foods added with different percentages of milk or egg powder, using the sandwich and competitive ELISA formats.
In an interlaboratory study performed by Poms et al. (Citation2005) to determine peanut proteins added to biscuit samples at a level of 2.5, 5 and 10 mg/kg, reproducibility values reported, expressed as the interlaboratory relative standard deviation (RSDR), were 127.0%, 73.6% and 58.2%, respectively, for the same ELISA test. Sánchez, Pérez, Puyol, Calvo, and Brett (Citation2002) performed an interlaboratory study to validate an ELISA test to determine soy proteins in milk samples and reported RSDR values between 14.0 and 74.5%. In the study carried out by Matsuda et al. (Citation2006) to detect egg and milk proteins in processed foods, RSDR values for the two ELISA test used were less than 17% in all food samples analysed.
On the other hand, it is also remarkable from results obtained in our work that the concentration of β-lactoglobulin and ovomucoid in positive food samples containing the same amount of milk and egg powder was higher for the indirect competitive than for the sandwich format. Because the food extracts and the calibration standards were shared between the two tests for the same proteins, these discrepancies may be due in part to differences in the reactivity between the denatured protein and the antibodies used in each format.
Furthermore, there are some differences that could be attributed to the format used. The sandwich format requires antibodies directed against two or more distinct epitopes whereas the indirect competitive format is a technique that uses a one-epitope approach for the antibody to recognize a protein in the sample. The type of immunoassay is also important with respect to the way that a protein is presented to its specific antibodies. In the sandwich format, the immobilized antibodies serve to specifically capture the soluble antigen that is present in the sample. However, in the indirect competitive format, the antigen in the sample will compete with the antigen coating on the wells for the binding of a limited amount of antibodies and thus, the accessibility of determinants for an adsorbed protein might differ from the protein in solution (de Luis et al., 2007; Yeung, Citation2006).
Several interlaboratory studies have shown that quantitative results obtained when using different ELISA tests can vary significantly. In the work of Matsuda et al. (Citation2006), they compared two ELISA tests to determine egg proteins and two ELISA tests to determine milk proteins in model foods and found differences in the average concentration value for the same sample of up to 127 and 198%, respectively. In the interlaboratory validation study of five commercial test kits for the determination of peanut proteins in foods, the variation in the recoveries found between the different test kits had a spread of 44–191% across all concentrations (Poms et al., 2005).
Conclusions
This work was performed to compare indirect competitive and double antibody sandwich ELISA kits to determine either β-lactoglobulin or ovomucoid in three model processed foods containing milk and egg as ingredients.
The performance of each format was dependant on the target protein and the type of food sample. The detection of milk addition in food products by ELISA using β-lactoglobulin as the target protein was better when using the sandwich format than the indirect competitive format, due to its higher sensitivity and specificity.
However, in the case of the detection of egg addition in foods by ELISA using ovomucoid as the target protein, the indirect competitive format resulted better than the sandwich format because, although both formats had the same sensitivity for two of the products analysed (sausage and bread), only the indirect competitive format could detect ovomucoid in pâté.
The intensity of heat processing also had a great influence on the detection of the allergenic proteins, β-lactoglobulin and ovomucoid, in model foods. The amount of milk and egg powder necessary to give a positive result was lower in pasteurised products, followed by baked and sterilised products.
These findings underline the fact that the determination of allergenic proteins in food products is greatly influenced by the particularities of each ELISA format used as well as by heat processing conditions applied to food products. These considerations should be taken into account for a correct interpretation of results obtained when using different immunoassays to detect allergens in foods.
Acknowledgements
This work has been supported by grant PLANICYT AGL2005-05494/ALI from the Comisión Interministerial de Ciencia y Tecnología and by PM035 (2006) from the Gobierno de Aragón. Ruth de Luis and María Lavilla are recipients of a Fellowship from the Gobierno de Aragón.
References
- Besler , M. , Steinhart , H. and Paschke , A. 2001 . Stability of food allergens and allergenicity of processed foods . Journal of Chromatography , 756 : 207 – 228 .
- Bush , R.K. and Hefle , S.L. 1996 . Food allergens . Critical Reviews in Food Science and Nutrition , 36(S) : S119 – S163 .
- Deibel , K. , Trautman , T. , DeBoom , T. , Sveum , W.H. , Dunaif , G. Scott , V.N. 1997 . A comprehensive approach to reduce the risk of allergens in foods . Journal of Food Protection , 60 : 436 – 441 .
- De Luis , R. , Pérez , M.D. , Sánchez , L. , Lavilla , M. and Calvo , M. 2007 . Development of two immunoassay formats to detect β-lactoglobulin: Influence of heat treatment on β-lactoglobulin immunoreactivity and assay applicability in processed food . Journal of Food Protection , 70 : 1691 – 1697 .
- European Commission . 2003 . Directive of the European Parliament and of the Council of 10 November 2003 amending Directive 2001/13/EC as regards indication of the ingredients present in foodstuffs . Official Journal L308 , 15 – 18 .
- Hefle , S.L. and Lambrecht , D.M. 2004 . Validated enzyme-linked immunosorbent assay for casein and its application to retail and milk-allergic complaint foods . Journal of Food Protection , 67 : 1933 – 1938 .
- Immer , U. 2006 . “ Factors affecting the effectiveness of allergen detection ” . In Detecting allergens in foods , Edited by: Koppelman , S.J. and Hefle , S.L. 330 – 347 . Cambridge, MA : Woodhead .
- Julià , S. , Sánchez , L. , Pérez , M.D. , Lavilla , M. , Conesa , C. and Calvo , M. 2007 . Effect of heat treatment on hen's egg ovomucoid: An immunochemical and calorimetric study . Food Research International , 40 : 603 – 612 .
- Mariager , B. , Solve , M. , Eriksen , H. and Brogren , C.H. 1994 . Bovine β-lactoglobulin in hypoallergenic and ordinary infant formulas measured by an indirect competitive ELISA using monoclonal and polyclonal antibodies . Food and Agricultural Immunology , 6 : 73 – 83 .
- Matsuda , R. , Yoshioka , Y. , Akiyama , H. , Aburtani , K. , Watanabe , Y. Matsumoto , T. 2006 . Interlaboratory evaluation of two-linked immunosorbent assays kits for the detection of egg, milk, wheat, buckwheat and peanuts in foods . Journal of AOAC International , 89 : 1600 – 1608 .
- Monaci , L. , Tregoat , V. , van Hengel , A.J. and Anklam , E. 2006 . Milk allergens, their characteristics and their detection in food. A review . European Food Research and Technology , 223 : 149 – 179 .
- Poms , R.E. 2006 . “ Reference material and method validation in allergen detection ” . In Detecting Allergens in Foods , Edited by: Koppelman , S.J. and Hefle , S.L. 348 – 356 . Cambridge, MA : Woodhead .
- Poms , R.E. , Agazzi , M.E. , Bau , A. , Brohee , M. , Capelletti , C. Norgaard , J.V. 2005 . Inter-laboratory validation study of five commercial ELISA test kits for the determination of peanut proteins in biscuits and dark chocolate . Food Additives and Contaminants , 22 : 104 – 112 .
- Poms , R.E. , Klein , C.L. and Anklam , E. 2004 . Methods for allergen analysis in food: A review . Food Additives and Contaminants , 21 : 1 – 31 .
- Sánchez , L. , Pérez , M.D. , Puyol , P. , Calvo , M. and Brett , G. 2002 . Determination of vegetal proteins in milk powder by enzyme-linked immunosorbent assay: Interlaboratory study . Journal of AOAC International , 85 ( 6 ) : 1390 – 1397 .
- Van Hengel , A.J. 2007 . Declaration of allergens on the label of food products purchased on the European market . Trends in Food Science and Technology , 18 : 96 – 100 .
- Yeung , J. 2006 . “ Enzyme-linked immunosorbent assay (ELISAs) for detecting allergens in foods ” . In Detecting allergens in foods , Edited by: Koppelman , S.J. and Hefle , S.L. 109 – 124 . Cambridge, MA : Woodhead .