Abstract
In the present study, we questioned whether an oral formulation made from soybean seeds and containing the mucosal adjuvant, cholera toxin (CT), could induce any detectable antibody responses against soy proteins. Groups of mice were repeatedly gavaged with formulations containing high (15 mg) and low (1 mg) doses of soybean seed protein extracts, along with CT as an oral adjuvant. While robust mucosal and systemic antibody responses against CT were observed, no detectable IgA, IgM, IgG or IgE anti-soy protein antibodies could be found. Furthermore, mice in all treatment groups remained healthy and continued to gain weight over the course of the study. Collectively, these data demonstrate that oral soybean-based vaccine formulations do not induce detectable antibody responses to soy proteins, even when the formulations contain a potent mucosal adjuvant. These studies underscore the safety of oral vaccine formulations which might contain soy proteins as a component.
Introduction
Vaccines are often formulated with a multitude of components. The immunogen may consist of attenuated or killed pathogens, complex microbial structures, subunit proteins or other molecules (Dorner & Barrett, Citation1999). However, additional components are typical in vaccine formulations. These may include preservatives (Meyer, Ni, Hu, & Shi, Citation2007), stabilisers (Volkin, Burke, Sanyal, & Middaugh, Citation1996), irrelevant proteins (Nokleby, Citation2006), manufacturing residues (Eldred, Dean, Mcguire, & Nash, Citation2006) or adjuvants (Guy, Citation2007; Pashine, Valiante, & Ulmer, Citation2005). While some of these components contribute to the usefulness and efficacy of vaccine formulations, they also have the ability to generate side effects when administered to patients. In rare cases (Georgitis & Fasano, Citation2001; Heidary & Cohen, Citation2005; Jacobson, Citation2003; Moylett & Hanson, Citation2004), side effects to some of the components in vaccine formulations can be life threatening. As new and developmental vaccines become more complex, an understanding of the impact that these formulations have on their safety remains a priority (Bonhoeffer, Zumbrunn, & Heininger, Citation2005; Duclos, Citation2004; Martin, Nelson, Hershey, & Engler, Citation2003).
Historically, subunit protein immunogens have been acknowledged for their safety as components of vaccine formulations, when compared to attenuated or killed pathogen-based vaccines (Liljeqvist & Stahl, Citation1999). Unfortunately, these subunit proteins can be poorly immunogenic, and often require the inclusion of adjuvants (Guy, Citation2007; Pashine et al., Citation2005) or components which facilitate their delivery or stability (Volkin et al., Citation1996) to increase efficacy. Therefore, when considering subunit protein vaccines, the safety of all the components ultimately used in their formulations must be considered.
In an effort to develop a platform technology for expressing immunomodulatory proteins for oral administration, our laboratory has investigated the usefulness of transgenic soybean seeds as a method to produce subunit vaccine candidates (Garg et al., Citation2007; Piller et al., Citation2005). When formulating these immunogens into oral vaccines, it was not altogether clear whether it would be necessary to purify the transgenic proteins from seeds prior to use, or whether seed preparations containing normal soy proteins might also be innocuous if present in vaccines. Soy protein is present in the diets of most individuals (Messina, Citation1999; Motil, Citation2000; Seppo et al., Citation2005; Slavin, Citation1991), and only a small percentage have adverse reactions following consumption of soy proteins (Cordle, Citation2004; Friedman & Brandon, Citation2001). Soy protein is also a staple in livestock feeds (Friedman & Brandon, Citation2001). Therefore, dietary soy proteins appear to be quite safe for most individuals or agricultural animals. However, soybean formulations used for oral vaccination would likely contain an adjuvant to boost efficacy. Therefore, one must consider the co-administration of soy proteins containing adjuvants when addressing potentially deleterious responses.
The answer to this safety question may depend upon the diet of the individual or animal under consideration. In an animal model, naïve mice never exposed to soy in their diets could respond to soy proteins when co-administered with an adjuvant (Gizzarelli et al., Citation2006). However, it is not altogether clear whether mice fed a standard laboratory diet containing up to 20% soy protein can respond to such proteins, even when adjuvant is present in soybean containing formulations (Bozelka, Carrow, Pahud, & Lehrer, Citation1987). To begin an understanding of the immunogenicity of soy proteins in oral formulations containing the potent adjuvant, cholera toxin (CT; Freytag & Clements, Citation2005), mice reared on a standard laboratory diet were repeatedly exposed to such formulations. In the present study, we found significant mucosal and systemic antibody responses against CT following gastric administration of soybean formulations containing this adjuvant. However, we observed no detectable anti-soy protein antibodies in these mice. Taken together, these studies suggest the safety of adjuvanted soy-based vaccine formulations in individuals or in livestock who routinely consume soy protein in their regular diets.
Materials and methods
Preparation of soybean seed extracts for dosing
Defatted soybean powder was prepared using protocols from previously described works reviewed by Lui (Citation1999) with modifications as follows. Soybean seeds (Glycine max Merr.) cultivator Throne were ground to a powder followed by incubation with 10 volumes of hexane at 65°C for 10 minutes with occasional rocking. The extraction process was repeated two additional times using fresh hexane. Seed material was collected by centrifugation (1200×g for 5 minutes at 23°C) and washed three times in 100% ethanol. Defatted seed material was dried for 30 minutes in a Centrivap concentrator (Labconco, Kansas City, MO, USA) at 60°C. Total soluble protein from defatted soybean powder was extracted in phosphate-buffered saline (PBS) followed by sonication and clarification as previously described. Total soluble protein in extracts were quantified by the Bradford method and doses of 200 µl with either 15 mg or 1 mg of soybean seed protein were prepared and kept at −20°C until use. On the day of vaccination, samples were thawed at room temperature, followed by boiling for 10 minutes. After samples cooled to room temperature, CT (10 µg/mouse, Sigma, St. Louis, MO, USA) was added as an adjuvant to soy protein formulations immediately before treatment.
Mice
Female C57BL/6 mice were purchased from Jackson Laboratory at 6–8 weeks of age and were maintained for four weeks with free access to food and water. All animals were fed laboratory chow (Harlan Teklad rodent diet 8604) containing approximately 18% soy protein. Water and chow was given ad libitum for the duration of study. All animal studies were approved by the University of North Carolina at Charlotte Institutional Animal Care and Use Committee.
Immunisation
Groups of mice were gavaged with 10 µg CT in PBS, soybean seed extract containing 15 mg total soluble protein in PBS, or soybean seed extract containing 15 mg or 1 mg total soluble protein with CT in PBS. Oral administration (200 µl) was performed using an 18 gauge stainless steel gavage needle (Popper & Sons, Inc., New Hyde Park, NY, USA) on days 0, 14, 28, 42 and 56. Sera and faecal collection was conducted before immunisation on day 1, day 27 (week 4) and final collection on day 70 (week 12).
Sample collection
Blood samples were collected from individual mice via saphenous vein by sterile needle puncture and diluted in PBS. Blood samples were then centrifuged and sera collected and stored at −20°C until assayed.
Fecal pellets were collected from individual mice and lyophilised for 15 hours. Samples were then weighed and processed as previously described with modifications. Briefly, PBS containing a 1:100 dilution of cocktail of protease inhibitors (DMSO, 0.1M phenylmethylsulphonyl fluoride, 0.1M iodoacetamide, 0.5 M ethylenediaminetetraactetic acid, 1 mg/ml pepstatin A (Sigma, St. Louis, MO, USA)) was added at 10 µl/mg of dry fecal material. Samples were vortexed and soaked overnight at 4°C followed by centrifugation at 16,000×g for 15 minutes. The supernatants were collected and analysed for IgA.
Detection of antigen-specific antibody responses in serum and fecal extracts
Serum and faecal antibody titres from individual mice were determined by an enzyme-linked immunosorbent assay (ELISA). For CT-specific antibody titres, CT was adsorbed onto 96-well ELISA plate (Corning Glass Works, Corning, NY, USA) and incubated overnight at 4°C. For soy-specific antibody detection, total soluble soy protein was diluted to 1 mg/ml and aliquots stored at –20°C until use. A concentration of 1 µg/ml of soy protein was adsorbed onto 96-well ELISA plates and incubated overnight at 4°C. Following incubation, plates were blocked with PBS containing 3% BSA for two hours, followed by the addition of serial dilutions of sera or faecal extracts. After an overnight incubation at 4°C, plates were washed and incubated for one hour with appropriate goat anti-mouse IgM, IgG, IgG1, IgG2 or IgA antibodies conjugated with horseradish peroxidase (Southern Biotechnology, Birmingham, AL, USA) for one hour at room temperature. For IgE detection, serial dilutions of sera from individual animals was applied to wells and incubated over night at 4°C. Biotinylated anti-mouse IgE antibody was applied to wells and incubated for one hour followed by a 30-minute incubation with strep-avidin (BD Biosciences Pharmingen, San Diego, CA, USA). Plates were washed and incubated with substrate (TMB, BioFx Laboratories, Owens Mills, MD, USA) to detect bound antibody. Enzymatic reactions were stopped by the addition of 1 M H2S04 and optical absorbances at 450 nm were determined.
Statistics
Statistical differences were determined by subjecting data to a two-way ANOVA test with Bonferroni post hoc test for comparison of means using GraphPad Prism software (GraphPad Software, San Diego, CA, USA).
Results
Mucosal antibody response to cholera toxin (CT) with no detectable antibody response to soy proteins
To investigate whether soy proteins were immunogenic when given in oral formulations containing CT adjuvant, groups of mice were fed a standard laboratory diet containing soy protein for four weeks before oral administration of adjuvanted soy protein formulations. At this point, groups of mice were gavaged with 10 µg CT in PBS, soybean seed extract containing 15 mg total soluble protein in PBS, or soybean seed extracts containing 15 mg or 1 mg total soluble protein with CT in PBS. All groups of mice were treated five times at biweekly intervals. At 28 and 70 days during this immunisation scheme, faecal samples were collected. ELISAs to detect specific IgA antibodies against CT were performed, and the results are shown in A and B. As expected, all animals receiving CT in PBS or CT containing soy protein formulations showed a significant increase in faecal IgA anti-CT antibodies when compared to animals receiving soy protein alone (p<0.01). The results in clearly demonstrate the ability of these animals to mount a mucosal antibody response against this bacterial protein.
Figure 1. Detection of IgA anti-CT antibodies in faecal material. Groups of mice were gavaged with 10 µg of CT in PBS (N=4), soybean seed extract containing 15 mg of total soluble protein in PBS (N=4), or soybean seed extract containing 15 mg (N=5) or 1 mg (N=6) of total soluble protein with CT in PBS on days 0, 14, 28, 42 and 56. Faecal IgA anti-CT antibody reactivity was determined by ELISA at day 28 (A) or day 70 (B) at the indicated serial dilution of faecal material. Results are presented as mean absorbance values for each group of animals. Standard deviations were always less than 5% of mean values.
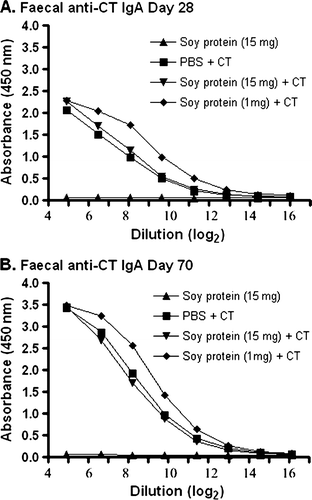
Conversely, no detectable IgA anti-soy protein antibodies were observed when the same faecal samples as those used in were assayed for anti-soy antibodies (). This was true even when the adjuvant, CT, was present in the gavaged soy protein formulations (A and B).
Figure 2. Detection of IgA anti-soy protein antibodies in fecal material. Groups of mice were gavaged with 10 µg of CT in PBS (N=4), soybean seed extract containing 15 mg of total soluble protein in PBS (N=4), or soybean seed extract containing 15 mg (N=5) or 1 mg (N=6) of total soluble protein with CT in PBS on days 0, 14, 28, 42 and 56. Faecal IgA anti-soy protein antibody reactivity was determined by ELISA at day 28 (A) or day 70 (B) at the indicated serial dilution of faecal material. Results are presented as mean absorbance values for each group of animals. Standard deviations were always less than 5% of mean values.
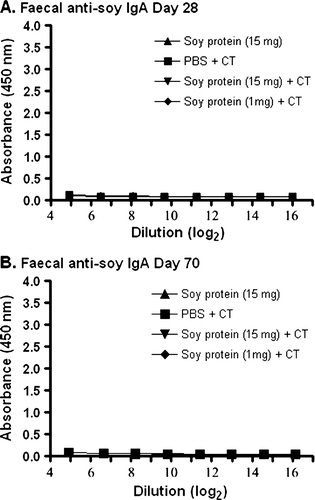
Systemic antibody response to cholera toxin (CT) with no detectable antibody response to soy proteins
To determine if IgG antibody titres were present following oral gavage, similar studies were performed on dilutions of sera from these groups of mice. As expected (A and B), all animals receiving CT in PBS or CT containing soy protein formulations showed a significant increase in total serum IgG anti-CT antibodies, when compared to animals receiving soy protein alone (p<0.01). The IgG anti-CT antibody response was predominantly of the IgG1 subclass (C), and not IgG2 (D), as would be expected based on previous studies (Marinaro et al., Citation1999). The results in clearly demonstrate the ability of these animals to mount a systemic, predominantly T helper type 2, antibody response against CT following a mucosal challenge.
Figure 3. Detection of IgG anti-CT antibodies in serum. Groups of mice were gavaged with 10 µg of CT in PBS (N=4), soybean seed extract containing 15 mg of total soluble protein in PBS (N=4), or soybean seed extract containing 15 mg (N=5) or 1 mg (N=6) of total soluble protein with CT in PBS on days 0, 14, 28, 42 and 56. Total serum IgG anti-CT antibody reactivity was determined by ELISA at day 28 (A) or day 70 (B) at the indicated serial dilution of serum. Similarly, serum IgG1 (C) or IgG2 (D) anti-CT antibody reactivity was determined by ELISA at day 70 at the indicated serial dilution of serum. Results are presented as mean absorbance values for each group of animals. Standard deviations were always less than 5% of mean values.
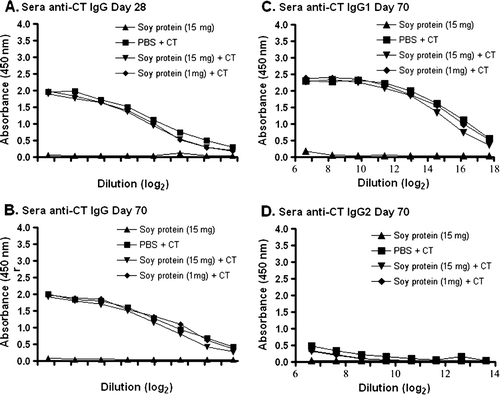
Conversely, no detectable IgG anti-soy protein antibodies were observed when the same sera samples as those used in were assayed for total IgG anti-soy antibodies (). This was true even when the adjuvant, CT, was present in the gavaged soy protein formulations (A and B).
Figure 4. Detection of IgG anti-soy protein antibodies in serum. Groups of mice were gavaged with 10 µg of CT in PBS (N=4), soybean seed extract containing 15 mg of total soluble protein in PBS (N=4), or soybean seed extract containing 15 mg (N=5) or 1 mg (N=6) of total soluble protein with CT in PBS on days 0, 14, 28, 42 and 56. Total serum IgG anti-soy protein antibody reactivity was determined by ELISA at day 28 (A) or day 70 (B) at the indicated serial dilution of serum. Results are presented as mean absorbance values for each group of animals. Standard deviations were always less than 5% of mean values.
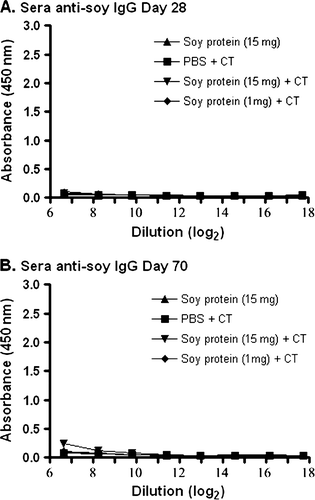
To investigate the possibility that the absence of an IgG anti-soy protein response was due to the lack of maturation of an IgM anti-soy protein antibody response, similar studies were performed on these sera. As shown in A, a detectable IgM anti-CT antibody response was observed in groups of mice exposed to this adjuvant at day 28 of the experimental protocol. However, no detectable IgM anti-soy protein antibody response was observed at day 28 (B).
IgE antibody response to cholera toxin (CT) with no detectable IgE antibody response to soy proteins
Based on the lack of an IgA anti-soy protein response in faecal material (), and the lack of IgG () and IgM () anti-soy protein responses in sera, we anticipated that it would be highly unlikely to detect an IgE anti-soy protein antibody response. Nonetheless, in an effort to be comprehensive, A shows a detectable IgE anti-CT antibody response in sera of mice exposed to this adjuvant at day 70 of the experimental protocol. However, no detectable IgE anti-soy protein antibody response in the sera was observed at day 70 (b).
Figure 5. Detection of IgM anti-CT or IgM anti-soy protein antibodies in serum. Groups of mice were gavaged with 10 µg of CT in PBS (N=4), soybean seed extract containing 15 mg of total soluble protein in PBS (N=4), or soybean seed extract containing 15 mg (N=5) or 1 mg (N=6) of total soluble protein with CT in PBS on days 0 and 14. Total serum IgM anti-CT (A) or IgM anti-soy protein (B) antibody reactivity was determined by ELISA at day 28 at the indicated serial dilution of serum. Results are presented as mean absorbance values for each group of animals. Standard deviations were always less than 5% of mean values.
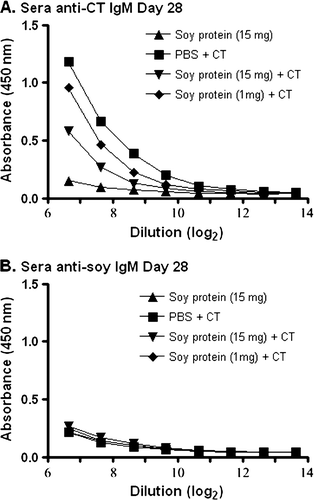
Weight gain in groups of mice gavaged with soy protein formulations containing cholera toxin (CT)
For extended immunisation regimens like the one used here, weight loss can be a sensitive indicator of the safety of a particular vaccine candidate. To determine whether the immunisation protocol affected growth rate, we monitored weight of animals through out the study. shows the mean body weight of each animal group at the beginning of the study (week 0) and the mean body weight of each animal group at the conclusion of the studies (week 12). There was no statistically significant difference in the mean body weights between any of the groups of mice at the conclusion of the study (p>0.05).
Discussion
Expression of foreign proteins in transgenic soybean seeds has been used for a variety of applications (Zeitlin et al., Citation1998), including the expression of immunogens for use as subunit vaccine candidates (Garg et al., Citation2007; Moravec et al., Citation2007; Piller et al., Citation2005). For example, the B subunit of heat labile toxin of enterotoxigenic Escherichia coli has been expressed in soybean seeds, and immunisation with this transgenic protein could stimulate partially protective immune responses in mice (Moravec et al., Citation2007). In earlier work, our laboratory expressed the K99 fimbrial subunit, FanC, of E. coli in soybean and demonstrated its immunogenicity in mice (Garg et al., Citation2007; Piller et al., Citation2005). Together, studies such as these demonstrate the feasibility of using transgenic soybean seeds as a platform technology for expressing immunogens which can be used in vaccine formulations.
In fact there appear to be some significant advantages for the use of transgenic soybean seeds to express immunogens when considering other technologies. One function of the soybean seed is to store protein. Approximately 40% of the soybean seed is protein, and this trait can be exploited to express high levels of the particular immunogen in transgenic seeds (Garg et al., Citation2007; Moravec et al., Citation2007; Piller et al., Citation2005). This high level of immunogen expression results in a very low cost of production for each dose of an immunogen. For example, if expression of an immunogen is merely 1% of the total seed protein, a 1 mg quantity would be contained in less than two soybean seeds. At current costs for soybean production (http://www.soystats.com), this represents less than 0.01 cents per 1 mg of immunogen. The fact that soybean seeds can store protein for long periods of time without degradation also suggests that long-term stability of transgenic immunogens expressed in these seeds would also be a possibility, even in the absence of refrigeration. These possible advantages of using soybean seeds to express transgenic proteins are being explored in ongoing studies in our laboratory.
The present work begins to question another potential advantage of expressing immunogens in transgenic soybean seeds. We questioned whether it might be possible to process transgenic soybean seeds into oral vaccine formulations without the necessity of isolating the immunogen from normal soy proteins. Such oral formulations would likely contain a mucosal adjuvant (Freytag & Clements, Citation2005), which raises the important consideration of immune responses developing against soy proteins. The safety of soy proteins in foodstuffs is well documented (Messina, Citation1999; Motil, Citation2000; Seppo et al., Citation2005; Slavin, Citation1991) with only a small percentage of the population having food allergies against this agricultural product (Cordle, Citation2004; Friedman & Brandon, Citation2001). In fact, soy milk formulations are so safe that they are routinely fed to infants (Badger, Ronis, Hakkak, Rowlands, & Korourian, Citation2002). However, the use of soy formulations for oral vaccination is complicated by the possibility that adjuvants might be added to boost the efficacy of immunisation at mucosal surfaces. Therefore, the more complicated question of the safety of adjuvanted formulations containing soy protein must be addressed.
In the present study, we questioned whether mice fed laboratory chow containing approximately 18% soy protein ad libitum could mount a detectable antibody response against soy proteins co-administered with the adjuvant, CT. Immunisation of naïve mice that were never exposed to soy in their diets demonstrated that these animals could respond to soy proteins when an adjuvant was present (Gizzarelli et al., Citation2006). However, most human diets (Messina, Citation1999; Motil, Citation2000; Seppo et al., Citation2005; Slavin, Citation1991), and many standard laboratory rodent chows, contain significant amounts of soy protein. One previous study suggested that mice fed normal diets did not mount a specific IgE response against soy proteins given intragastrically with pertussis toxin (Bozelka et al., Citation1987). Here, we clearly demonstrate the lack of a detectable antibody response against soy proteins in mice immunised orally with soybean formulations containing the mucosal adjuvant, CT (Freytag & Clements, Citation2005). This was true for mucosal IgA responses (), as well as systemic IgM (), IgG () and IgE () responses at 28 days and/or 70 days post-immunisation. Normal weight gain over the course of this immunisation regimen also suggests the safety of such formulations containing soy protein with an adjuvant in this mouse model ().
Figure 6. Detection of IgE anti-CT or IgE anti-soy protein antibodies in serum. Groups of mice were gavaged with 10 µg of CT in PBS (N=4), soybean seed extract containing 15 mg of total soluble protein in PBS (N=4), or soybean seed extract containing 15 mg (N=5) or 1 mg (N=6) of total soluble protein with CT in PBS on days 0, 14, 28, 42 and 56. Total serum IgE anti-CT (A) or IgE anti-soy protein (B) antibody reactivity was determined by ELISA at day 70 at the indicated serial dilution of serum. Results are presented as mean absorbance values for each group of animals. Standard deviations were always less than 5% of mean values.
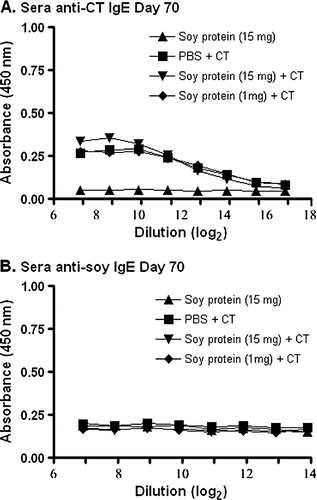
Figure 7. Total body weight comparisons at week 12. Groups of mice were weighed (week 0, open bars) and then gavaged with 10 µg of CT in PBS (N=4), soybean seed extract containing 15 mg of total soluble protein in PBS (N=4), or soybean seed extract containing 15 mg (N=5) or 1 mg (N=6) of total soluble protein with CT in PBS on days 0, 14, 28, 42 and 56. Mice were then weighed at the conclusion of the experimental protocol (week 12, closed bars). Results are presented as mean weights for each group of animals. Standard deviations were always less than 10% of mean values.
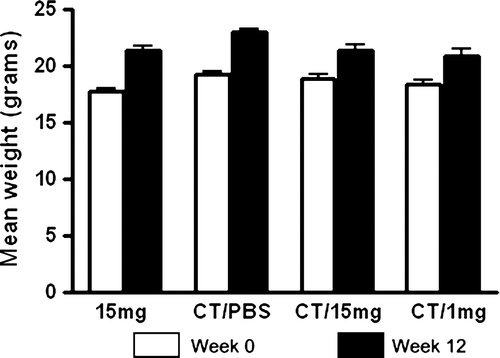
A necessary criterion for a safe vaccine formulation is that it does not stimulate deleterious immune responses. Therefore, it is necessary to investigate whether particular components present in such formulations might be deleterious. Collectively, the data presented here demonstrate that oral soybean-based vaccine formulations do not induce detectable antibody responses to soy proteins, even when the formulations contain a potent mucosal adjuvant. These studies suggest the safety of adjuvanted soy-based vaccine formulations in individuals or in livestock who routinely consume soy protein in their regular diets.
References
- Badger , T.M. , Ronis , M.J. , Hakkak , R. , Rowlands , J.C. and Korourian , S. 2002 . The health consequences of early soy consumption . Journal of Nutrition , 132 : 559S – 565S .
- Bonhoeffer , J. , Zumbrunn , B. and Heininger , U. 2005 . Reporting of vaccine safety data in publications: Systematic review . Pharmacoepidemiology and Drug Safety , 14 : 101 – 106 .
- Bozelka , B.E. , Carrow , E. , Pahud , J.J. and Lehrer , S.B. 1987 . Soy- and shrimp-specific IgE responses in orally and intraperitoneally immunized mice . International Archives of Allergy and Applied Immunology , 83 : 271 – 277 .
- Cordle , C.T. 2004 . Soy protein allergy: Incidence and relative severity . Journal of Nutrition , 134 : 1213S – 1219S .
- Dorner , F. and Barrett , P.N. 1999 . Vaccine technology: Looking to the future . Annals of Medicine , 31 : 51 – 60 .
- Duclos , P. 2004 . A global perspective on vaccine safety . Vaccine , 22 : 2059 – 2063 .
- Eldred , B.E. , Dean , A.J. , Mcguire , T.M. and Nash , A.L. 2006 . Vaccine components and constituents: Responding to consumer concerns . Medical Journal of Australia , 184 : 170 – 175 .
- Freytag , L.C. and Clements , J.D. 2005 . Mucosal adjuvants . Vaccine , 23 : 1804 – 1813 .
- Friedman , M. and Brandon , D.L. 2001 . Nutritional and health benefits of soy proteins . Journal of Agricultural and Food Chemistry , 49 : 1069 – 1086 .
- Garg , R. , Tolbert , M. , Oakes , J.L. , Clemente , T.E. , Bost , K.L. and Piller , K.J. 2007 . Chloroplast targeting of fanc, the major antigenic subunit of Escherichia coli k99 fimbriae, in transgenic soybean . Plant Cell Repots , 26 : 1011 – 1023 .
- Georgitis , J.W. and Fasano , M.B. 2001 . Allergenic components of vaccines and avoidance of vaccination-related adverse events . Current Allergy Reports , 1 : 11 – 17 .
- Gizzarelli , F. , Corinti , S. , Barletta , B. , Iacovacci , P. , Brunetto , B. Butteroni , C. 2006 . Evaluation of allergenicity of genetically modified soybean protein extract in a murine model of oral allergen-specific sensitization . Clinical and Experimental Allergy , 36 : 238 – 248 .
- Guy , B. 2007 . The perfect mix: Recent progress in adjuvant research. Nature Reviews . Microbiology , 5 : 505 – 517 .
- Heidary , N. and Cohen , D.E. 2005 . Hypersensitivity reactions to vaccine components . Dermatitis , 16 ( 3 ) : 115 – 120 .
- Jacobson , R.M. 2003 . Vaccine safety . Immunology and Allergy Clinics of North America , 23 : 589 – 603 .
- Liljeqvist , S. and Stahl , S. 1999 . Production of recombinant subunit vaccines: Protein immunogens, live delivery systems and nucleic acid vaccines . Journal of Biotechnology , 73 : 1 – 33 .
- Lui , K. 1999 . Soybeans: Chemistry, technology and utilization , Gaithersburg, MD : Aspen .
- Marinaro , M. , Boyaka , P.N. , Jackson , R.J. , Finkelman , F.D. , Kiyono , H. Jirillo , E. 1999 . Use of intranasal Il-12 to target predominantly TH1 responses to nasal and TH2 responses to oral vaccines given with cholera toxin . Journal of Immunology , 162 : 114 – 121 .
- Martin , B.L. , Nelson , M.R. , Hershey , J.N. and Engler , R.J. 2003 . Adverse reactions to vaccines . Clinical Reviews in Allergy and Immunology , 24 : 263 – 276 .
- Messina , M.J. 1999 . Legumes and soybeans: Overview of their nutritional profiles and health effects . American Journal of Clinical Nutrition , 70 ( Suppl. 3 ) : 439S – 450S .
- Meyer , B.K. , Ni , A. , Hu , B. and Shi , L. 2007 . Antimicrobial preservative use in parenteral products: Past and present . Journal of Pharmaceutical Sciences , 96 : 3155 – 3167 .
- Moravec , T. , Schmidt , M.A. , Herman , E.M. and Woodford-Thomas , T. 2007 . Production of Escherichia coli heat labile toxin (LT) B subunit in soybean seed and analysis of its immunogenicity as an oral vaccine . Vaccine , 25 : 1647 – 1657 .
- Motil , K.J. 2000 . Infant feeding: A critical look at infant formulas . Current Opinion in Pediatrics , 12 : 469 – 476 .
- Moylett , E.H. , & Hanson , I.C. 2004 . Mechanistic actions of the risks and adverse events associated with vaccine administration . Journal of Allergy and Clinical Immunology , 114 , 1010 1020 ; quiz 1021 .
- Nokleby , H. 2006 . Vaccination and anaphylaxis . Current Allergy and Asthma Reports , 6 : 9 – 13 .
- Pashine , A. , Valiante , N.M. and Ulmer , J.B. 2005 . Targeting the innate immune response with improved vaccine adjuvants . Nature Medicine , 11 ( Suppl. 4 ) : S63 – S68 .
- Piller , K.J. , Clemente , T.E. , Jun , S.M. , Petty , C.C. , Sato , S. Pascual , D.W. 2005 . Expression and immunogenicity of an Escherichia coli k99 fimbriae subunit antigen in soybean . Planta , 222 : 6 – 18 .
- Seppo , L. , Korpela , R. , Lonnerdal , B. , Metsaniitty , L. , Juntunen-Backman , K. Klemola , T. 2005 . A follow-up study of nutrient intake, nutritional status, and growth in infants with cow milk allergy fed either a soy formula or an extensively hydrolyzed whey formula . American Journal of Clinical Nutrition , 82 : 140 – 145 .
- Slavin , J. 1991 . Nutritional benefits of soy protein and soy fiber . Journal of the American Dietetic Association , 91 : 816 – 819 .
- Volkin , D.B. , Burke , C.J. , Sanyal , G. and Middaugh , C.R. 1996 . Analysis of vaccine stability . Developments in Biological Standardization , 87 : 135 – 142 .
- Zeitlin , L. , Olmsted , S.S. , Moench , T.R. , Co , M.S. , Martinell , B.J. Paradkar , V.M. 1998 . A humanized monoclonal antibody produced in transgenic plants for immunoprotection of the vagina against genital herpes . Nature Biotechnology , 16 : 1361 – 1364 .