Abstract
The effect of weight ratio of protein to sugar (0.17–7.83), temperature (40–60°C) and time (24–120 h) on the antigenicity of β-lactoglobulin (β-LG) in conjugates of whey protein isolate (WPI) with glucose was investigated. Response surface methodology was used to carry out an optimisation of the reaction conditions leading to the minimum antigenicity of β-LG. Conjugation of WPI with glucose could reduce effectively the antigenicity of β-LG. This reduction of antigenicity could be controlled by regulating three independent variables (weight ratio of WPI to glucose, temperature and time). The model for optimal reaction conditions of a lower antigenicity of β-LG was established. Weight ratio of WPI to glucose had the greatest effect on the antigenicity of β-LG. Reaction time influenced the antigenicity of β-LG to a lesser extent.
Introduction
Cow milk and its products are the main nutrients for humans, especially infants. However, cow milk is considered as an important allergen in food allergy. In many studies, cow milk protein allergy was reported to be the most prevalent for infants or young children, with an incidence of 2–6% (Hill & Hosking, Citation1996, Citation1997). All cow milk proteins may be potential allergens, of which the main allergens were found to be β-lactoglobulin (β-LG), α-lactalbumin (α-LA) and casein (Fritsche, Citation2003; Sharma, Pravindra, Betzel, & Singh, Citation2001). β-LG is a major protein in the milk (56–60% of total cow whey proteins) and is considered to be a dominant allergen. β-LG can reach the intestinal mucosa almost intact due to its resistance to pepsin hydrolysis, inducing allergenic reactions (Reddy, Kella, & Kinsella, Citation1988; Wal, Citation2001); about 82% of milk allergy patients are sensitive to β-LG (Spies, Citation1973).
Some processing approaches have been applied to reduce the allergenic potential of cow milk proteins, involving heat treatment, enzymatic hydrolysis, fermentation and glycation (Ehn, Allmere, Telemo, Bengtsson, & Ekstrand, Citation2005; Fritsche, Citation2003; Hattori et al., Citation2004). Of all these processes conjugation with reducing sugars through the Maillard reaction seems to be a promising and safe method for masking food protein allergenicity, and also be an effective means of improving functional properties of proteins. Glycation reaction has been proposed as a way to change the soy allergen structures, mask the allergenicity and enhance functional properties (Wilson, Blaschek, & Gonzalez de Mejia, Citation2005). It has been found that the antigenicity of soy protein isolate was largely reduced by glycation with both fructose and fructooligosaccharides (van de Lagemaat, Silván, Moreno, Olano, & del Castillo, Citation2007). Nakamura et al. (Citation2008) reported that the Maillard-type glycosylation of buckwheat Fag e 1 with arabinogalactan and xyloglucan brought about a drastic reduction of the allergenicity of Fag e 1. Some studies have revealed that β-LG allergenicity can be modified by conjugating with different saccharides. Morgan et al. (Citation1998) indicated that lactosylated β-LG produced by the Maillard reaction during heating changed the epitopic area. Hattori et al. (Citation2000) showed that the conjugate of β-LG and carboxymethyl dextran had improved emulsifying properties and reduced immunogenicity. β-LG conjugated with acidic oligosaccharides showed enhanced thermal stability and had reduced immunogenicity (Hattori et al., Citation2004).
The reduction in the antigenicity of β-LG depends on the type of sugar, reaction conditions and the degree of Maillard reaction. It had been confirmed from the above reports that conjugation with some sugars could reduce the antigenicity of β-LG. However, the influence of reaction conditions, such as temperature, time and weight ratio of protein to sugar on antigenicity of β-LG have not been studied systematically. In order to establish a predictive model of the effects of reaction conditions of glycation on β-LG antigenicity, response surface methodology (RSM) is employed in the present study to optimise reaction conditions. RSM has proven to be a valuable tool for simultaneous optimisation of several process parameters in biological and chemical sciences (Palasota & Deming, Citation1992; van der Ven, Gruppen, de Bont, & Voragen, Citation2002).
Whey proteins are widely used in food products as a kind of functional additive owing to their high nutritional value. β-LG is the major whey protein and it is largely responsible for the physicochemical characteristics of whey proteins. In this study, whey protein isolate (WPI) conjugates with glucose were investigated. Glucose is a common alimentary sugar in the food industry and is easy to be absorbed and utilised by the body. It has been shown that conjugation with glucose can improve the functional properties of β-LG as solubility, heat stability and emulsifying properties (Chevaliera, Choberta, Popineaub, Nicolasa, & Haertle, 2001; Nacka, Chobert, Burova, Leonil, & Haertle, Citation1998). However, fewer studies have been reported about the effect of glucose on the antigenicity of β-LG during Maillard reaction. Therefore, the purpose of this study is to investigate the effects of different dry-heating conditions of Maillard reaction (weight ratio of WPI to glucose, temperature and time) on the antigenicity of β-LG and use RSM to select optimal conditions at which a lower antigenicity of β-LG would be obtained.
Materials and methods
Materials
The antigen used for sensitisation studies and enzyme-linked immunosorbent assay (ELISA) was β-LG from bovine milk (L3908; purity, 90%; containing β-LG A and B; lyophilised power), which was purchased from Sigma Chemical Company (St. Louis, MO, USA).
WPI was obtained from Murray Goulburn Co-operative Co. Ltd (Melbourne, Australia). The powder contained total protein 85.2%, moisture 6.1%, ash 3.3% and carbohydrates 0.3%. Glucose was purchased from Beijing Chemical Reagents Company (Beijing, China). All other reagents used were of analytical grade.
Preparation of polyclonal antibodies
Antibodies were obtained from New Zealand white rabbits sensitised with bovine β-LG (Sigma, L3908) applying the immunisation protocol described in our previous study (Bu, Luo, Zheng, & Zheng, Citation2009). For the first immunisation, rabbits were injected intramuscularly with 1.0 mg β-LG per kg weight that had been dissolved in 0.9% sterile sodium chloride and emulsified with an equal amount of Freund's complete adjuvant (FCA, Sigma). Two weeks later the rabbits were given a subcutaneous injection with the same dose of antigen in the presence of incomplete Freund's adjuvant. The next two immunisations were made without adjuvant with the same dose of antigen every two weeks.
The production and titres of antibodies were controlled by the indirect ELISA method taking blood samples from the rabbit marginal vein 2 days before the subsequent scheduled immunisation. Ten days after the fourth sensitisation, blood samples were obtained from the rabbit hearts. Blood samples were incubated for 1 h at room temperature and left overnight at 4°C, then centrifuged for 20 min at 3000 g to obtain sera. The sera were stored at −20°C until the following analysis on the antigenicity of β-LG by the indirect competitive ELISA.
Preparation of the WPI–glucose (WPI–G) conjugates
The WPI–glucose (WPI–G) conjugates were prepared by Maillard reaction. WPI and glucose with varied weight ratio (w/w) based on the experimental design were dissolved in distilled water and lyophilised. The WPI–G powders were kept at different temperatures in desiccators equilibrated to 79% relative humidity using saturated solutions of KBr for various incubation times according to the experimental design. The powders were stored at −20°C until being analysed. Control samples used in this study were obtained by incubation of WPI and glucose alone under the same dry-heating conditions. WPI without dry-heating treatment was also used as a control.
Measurement of browning and free amino group content
Aqueous solutions of the reaction products (protein concentration of 1 mg/ml) were prepared and the browning of reaction products by Maillard reaction was measured by the absorbance at 420 nm.
The content of free amino groups was determined according to the trinitrobenzene sulfonic acid (TNBS) method (Adler-Nissen, Citation1979). The absorbance was measured at 420 nm, and was transformed into mmol of leucine/l using a calibration curve within the range 0.25–3.5 mmol/l.
The antigenicity analysis by the indirect competitive enzyme-linked immunosorbent assay (ELISA)
The antigenicity of β-LG in WPI and WPI–G conjugates was estimated by the indirect competitive ELISA. Microtiter plates with 96 wells (flat-bottomed; Costar, Corning, USA) were coated with 100 µl/well of antigen diluted in 50 mM carbonate buffer (pH 9.8) with 0.5 µg/ml for β-LG determined earlier by the indirect ELISA method and incubated overnight at 4°C. Sample solutions and standard antigen (β-LG) were, respectively, incubated overnight at 4°C in test tubes with an equivalent volume of rabbit anti-β-LG serum (1:6400) diluted in 10 mM phosphate-buffered saline (PBS, pH 7.4) containing 1% bovine serum albumin (BSA) and 0.1% Tween-20 (PBS-BSA-Tween-20). The plates were washed four times the next day with 10 mM PBS (pH 7.4) containing 0.05% Tween-20 (PBS-T). This washing procedure was repeated after each analytical step. After washing the plates, the wells were filled with 100 µl/well of PBS-BSA-Tween-20 to block residual free binding sites and incubated for 1 h at 37°C. The plates were washed and then 100 µl/well of reactive mixtures of samples or standard antigen and polyclonal rabbit antibodies (IgG) were added and incubated for 1 h at 37°C. After washing the plates, the wells were filled with 100 µl/well of horseradish peroxidase (HRP) conjugated goat anti-rabbit IgG (Sigma A 6154, 1:10,000) in PBS-BSA-Tween-20. After incubation for 1 h at 37°C, the plates were washed again and then 100 µl/well of 3,3′,5,5′-tetramethylenbenzidine (TMB, Amresco) substrate solutions were immediately added. The plates were incubated for 15 min at 37°C. Finally, 50 µl/well of 2 M H2SO4 were added to stop the reaction. Absorptions were determined at dual wavelengths of 450 and 630 nm by Multiskan MK3 ELISA plate reader (Thermo Labsystems, Franklin, MA, USA).
The antigenicity was calculated from standard curve of β-LG, and a linear logarithmic correlation was observed in the range: 0.01–400 µg/ml. All analyses were carried out in triplicate and the average values were converted to concentration equivalents in microgram per milliliter.
Inhibition rate (%) of the antigenicity of β-LG by Maillard reaction was calculated as follows:
Experimental design
In the experimental design, weight ratio of WPI to glucose (WR) (X 1), temperature (X 2) and time (X 3) were chosen as independent variables (k=3). The dependent Y variable is used to evaluate the antigenicity of β-LG in the conjugates of WPI with glucose. The independent variables were optimised using a central composite rotatable design (CCRD) containing five levels for each independent variable, coded as −1.682, −1, 0, +1, +1.682. Correspondence among these coded values and actual values of independent variables are given in . The complete central composite design consisted of 23 experiments for a full factorial design plus 2×3 star experiments and nine centre experiments, resulting in 23 experiments (). The centre experiments were chosen to verify any change in the estimation procedure, as a measure of precision property. Each glycation experiment was run in duplicate.
Table 1. Coded and uncoded settings of the independent variables for dry-heating according to a central composite rotatable design.
Statistical analysis
Experimental data were statistically analysed using SAS 8.2 (SAS Institute Inc., Cary, NC, USA). Response surface analysis was based on multiple regressions taking into account the main, the quadratic and the interaction effects, according to the following equation:
Results and discussion
Determination of the extent of glycation
The formation of brown colour is an indicator for the progress of a Maillard-type reaction. Determination of the browning degree was usually obtained by measuring absorbance at 420 nm (Ashoor & Zent, Citation1984). As shown in , dry-heated WPI did contribute slightly to browning, particularly at 60°C, this may be owing to the fact that traces of lactose presented in the proteins could be able to undergo Maillard reaction with whey protein (Einhorn-Stoll, Ulbrich, Sever, & Kunzek, Citation2005). In addition, glucose alone did not lead to absorbance increases (results not shown). However, an obvious increase in absorbance at 420 nm was detected for the WPI–G conjugates compared to native WPI and dry-heated WPI, which indicated that the Maillard reaction was taking place. It can be observed from that the brown colour was more intense with increasing temperature, reaction time and decreasing weight ratio of WPI to glucose, which suggested the increase of the extent of glycation.
Table 2. Changes in content of free amino groups and brown colour during the dry-heating of WPI and glucose.
Progress in protein glycation can also be confirmed by free amino group determination. For native WPI, no amino groups was modified, all results were reported relative to 100% of amino groups of native protein. It was found from that there was a small decrease in free amino group of dry-heated WPI and about an 11% loss of free amino groups was detected by dry-heating treatment at 60°C for 120 h, which could be explained by a slight Maillard reaction between whey proteins and residual lactose. Besides, it may also be attributed to structural modifications and masking effects caused by protein polymerisation (Chevaliera et al., Citation2001; Jimenez-Castano, Villamiel, Martin-Alvarez, Olano, & Lopez-Fandino, Citation2005). The percentage of free amino groups of glycated WPI decreased significantly compared to native WPI and dry-heated WPI (), which suggested the conjugation of whey protein with glucose. Moreover, the decrease of free amino group content in WPI–G conjugates with increasing temperature, reaction time and decreasing weight ratio of WPI to glucose indicated the advance of the degree of protein glycation. After glycation for 72 h at 60°C with 4:1 weight ratio of WPI to glucose, approximately 80% of free amino groups in WPI were blocked by glucose.
The antigenicity of β-lactoglobulin (bgr;-LG) in WPI–glucose (WPI–G) conjugates
Assessment on models of β-lactoglobulin (β-LG) antigenicity for three independent variables
The indirect competitive ELISA indicated that the antibody prepared against β-LG was special to β-LG, and no cross-reactivity was observed with other cow's milk proteins. The antigenicity of β-LG in WPI and WPI–G conjugates was determined by the indirect competitive ELISA method. The experimental design and results are presented in . Variations of the antigenicity of β-LG under different reaction conditions are observed. By regression analysis on 23 experiments, regression coefficients and overall performance of the initial regression analysis performed with all regression variables (, “full model”) revealed that several terms were not significant (P>0.05). In order to fit the full second-order model, non-significant terms were eliminated step by step from the regression model, starting with quadratic terms and interaction effects, ensuring that the determination coefficient (R 2) of the model did not change significantly (backward elimination). This procedure resulted in the second-order model with eight regression terms (, “fitted model”). By ANOVA, the P-value of the “fitted model” was 0.0001. In addition, the adjusted R 2 of the “fitted model” was 0.892. The very small P-value and high adjusted R 2 value indicated that the second-order model was highly significant and adequate to represent the actual relationship between responses and independent variables.
Table 3. Full factorial central composite design matrix for the antigenicity of β-LG in WPI–glucose conjugates.
Table 4. Regression coefficients for the linear regression model for prediction of the antigenicity of β-LG.
Effect of the dry-heating conditions on the antigenicity of β-lactoglobulin (β-LG)
Regression coefficients, 3D response surface plots and 2D contour plots were used to study the effects of various independent variables on the antigencity of β-LG ( and Figures ). From the analysis of regression coefficients (), both linear and quadratic effects of weight ratio of WPI to glucose and temperature were highly significant (P<0.01). For reaction time, the linear effect was significant (P<0.05) and the quadratic effect was highly significant (P<0.01). The interactive effect between temperature and time was highly significant (P<0.01). However, the interactive effects between weight ratio of WPI to glucose and temperature or time were not significant in comparison with the linear and quadratic effects. Among the three independent variables, the effect of weight ratio of WPI to glucose was the most important, and was reflected by the highest absolute regression coefficient.
Figure 1. 3D response surface plot and 2D contour plot using the b coefficients from the fitted model () for the effect of weight ratio of WPI to glucose (X 1) and temperature (X 2) on the antigenicity of β-LG (Y) at the reaction time for 72 h.
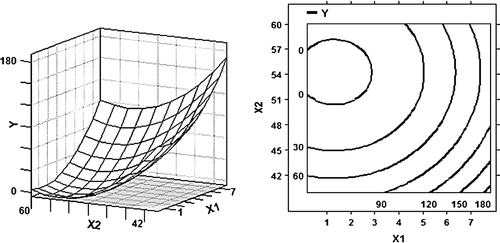
Figure 2. 3D response surface plot and 2D contour plot using the b coefficients from the fitted model () for the effect of weight ratio of WPI to glucose (X 1) and time (X 3) on the antigenicity of β-LG (Y) at a temperature of 50°C.
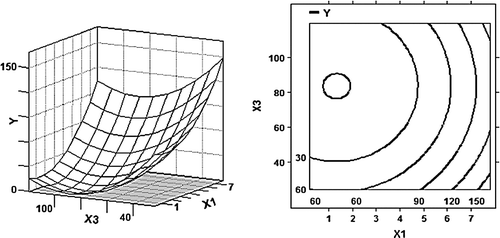
Figure 3. 3D response surface plot and 2D contour plot using the b coefficients from the fitted model () for the effect of temperature (X 2) and time (X 3) on the antigenicity of β-LG (Y) at weight ratio 4:1 of WPI to glucose.
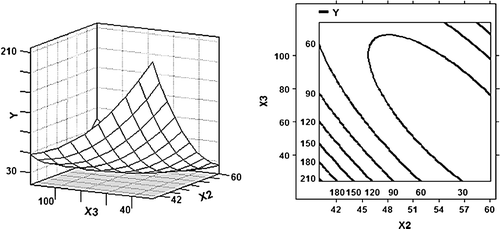
The effect of weight ratio of WPI to glucose and temperature is illustrated in 3D response surface plot and 2D contour plot (), where the reaction time variable was set on a constant value of 72 h. For weight ratio of WPI to glucose and temperature, the quadratic effects were highly significant, so their optimal values exist. It was observed from that the minimum value of the antigenicity could be attained at 50–55°C and 2–3 for weight ratio of WPI to glucose, after which the antigenicity increase again. The result indicates that the decrease of the antigenicity may be related to the quantity of sugars, the present study suggested that a moderate weight ratio of WPI to glucose during Maillard reaction can induce a lower antigenicity for β-LG. However, Hattori et al. (2000) showed that β-LG-CMD (carboxymethyl dextran) conjugates with a high saccharide content showed reduced immunogenicity. In addition, it was found that the larger the amount of polyethylene glycol (PEG), the lower the immunoreactivity of the conjugates of cow milk whey protein with PEG was (Wróblewska & Jedrychowski, Citation2002). This may be explained by the fact that the different nature of the conjugated saccharides affected the quality of the immune response (Hattori et al., Citation2004).
The effect of weight ratio of WPI to glucose and time is shown in , where the temperature variable is set on a constant value of 50°C. The optimal value of weight ratio of WPI to glucose and time exists due to their highly significant quadratic effect. From , it appears that the antigencity decreased initially with the decrease of weight ratio of WPI to glucose and the increase of reaction time and then increased. When weight ratio of WPI to glucose and reaction time are at 2–3 and 70–80 h, respectively, the antigenicity is minimal. It can be concluded from the result that the longer reaction time did not always bring lower antigenicity. This is similar to the changes of emulsifying properties of conjugates with increasing Maillard reaction time in other studies (Einhorn-Stoll et al., 2005; Miralles, Martínez-Rodríguez, Santiago, van de Lagemaat, & Heras, Citation2007). Thus, it is important to control the Maillard reaction time in order to obtain the desirable functional properties.
The effect of temperature and time is illustrated by 3D response surface plot and 2D contour plot depicted in , where weight ratio of WPI to glucose is set on a constant value of four. From , a minimum antigenicity is observed around temperature of 50°C and reaction time of 70 h. In this study, it was also detected that the antigenicity of β-LG in native WPI and dry-heated WPI at 40, 50 and 60°C for 120 h were 269.9, 273.3, 279.6 and 287.5 µg/ml, respectively. The small increase of β-LG antigenicity in dry-heated WPI compared to native WPI may be due to the exposure of some epitopes buried inside the native molecule owing to the unfolding of conformational structure (Kleber, Maier, & Hinrichs, Citation2007). However, the present study showed that the addition of glucose to WPI during dry-heating induced the decrease of β-LG antigenicity, which may suggest that glucose could shield off the epitopes in β-LG by the Maillard reaction. Furthermore, it has been demonstrated in this study that there was a considerable reduction in the antigenicity of β-LG by controlling temperature and reaction time.
The three independent variables (weight ratio of WPI to glucose, temperature and time) had different effects on the antigenicity of β-LG. The optimum values of the three independent variables to yield minimum levels of the antigenicity were as follows: WR, 2.59; temperature, 51.8°C; and time, 75.7 h. Under the above glycation reaction conditions, the antigenicity of β-LG was detected as 0.86 µg/ml, which indicated that the antigenicity of β-LG was decreased by 99.68% by the Maillard reaction compared to native WPI. Except for the antigenicity, changes the allergenicity of β-LG will be investigated due to the Maillard reaction in our future work. This is significant to develop a hypoallergenic milk product with high nutritional value by protein glycation.
The present results show that the degree of WPI glycation increased with increasing temperature, reaction time and decreasing weight ratio of WPI to glucose; however, the antigenicity of β-LG was reduced, followed by an increase in the degree of glycation. Changes in the β-LG antigenicity largely depend on the Maillard reaction conditions. In addition, the antigenicity of β-LG might be related to the conformational changes caused by Maillard reaction, which has been confirmed in the conjugation of β-LG and other sugars (Hattori et al., Citation2004). It was found that one of the mechanisms responsible for the reduced immunogenicity of β-LG-acidic oligosaccharides was the shielding of the epitopes resulting from the conjugation (Hattori et al., Citation2004). Therefore, further investigations are needed in order to elucidate the structural characteristics of WPI and glucose conjugates resulting in the decrease in a antigenicity of β-LG.
Conclusions
The conjugation with glucose could reduce the antigenicity of β-LG effectively. Under the range of conditions studied, weight ratio of WPI to glucose had the greatest effect on the antigenicity of β-LG. The Maillard reaction time influenced the antigenicity of β-LG to a lesser extent. RSM was an effective method to optimise several independent variables of the glycation reaction simultaneously, resulting in WPI–G conjugates with minimum antigenicity for β-LG. Although the antigenicity of β-LG (the main allergen of whey proteins) was not completely eliminated, it is assumed that the glycation of whey protein with glucose under optimal reaction conditions (weight ratio of WPI to glucose, temperature and time) is a promising procedure for preparing lower antigenic β-LG.
Acknowledgements
This work was supported financially by the National Natural Science Foundation of China (Award nos. 30471224 and 30871817) and the National Science and Technology Ministry of China (Award nos. 2006BAD27B04 and 2006BAD04A06).
References
- Adler-Nissen , J. 1979 . Determination of the degree of hydrolysis of food protein hydrolysates by trinitrobenzenesulfonic acid . Journal of Agricultural and Food Chemistry , 27 : 1256 – 1262 .
- Ashoor , S.H. and Zent , J.B. 1984 . Maillard browning of common amino acids and sugars . Journal of Food Science , 49 : 1206 – 1207 .
- Bu , G.H. , Luo , Y.K. , Zheng , Z. and Zheng , H. 2009 . Effect of heat treatment on the antigenicity of bovine α-lactalbumin and β-lactoglobulin in whey protein isolate . Food and Agricultural Immunology , 20 : 195 – 206 .
- Chevaliera , F. , Choberta , J.M. , Popineaub , Y. , Nicolasa , M.G. and Haertle , T. 2001 . Improvement of functional properties of β-lactoglobulin glycated through the Maillard reaction is related to the nature of the sugar . International Dairy Journal , 11 : 145 – 152 .
- Ehn , B.M. , Allmere , T. , Telemo , E. , Bengtsson , U. and Ekstrand , B. 2005 . Modification of IgE binding to β-lactoglobulin by fermentation and proteolysis of cow's milk . Journal of Agricultural and Food Chemistry , 53 : 3743 – 3748 .
- Einhorn-Stoll , U. , Ulbrich , M. , Sever , S. and Kunzek , H. 2005 . Formation of milk protein–pectin conjugates with improved emulsifying properties by controlled dry heating . Food Hydrocolloids , 19 : 329 – 340 .
- Fritsche , R. 2003 . Role of technology in dairy allergy . Australian Journal of Dairy Technology , 58 : 89 – 91 .
- Hattori , M. , Miyakawa , S. , Ohama , Y. , Kawamura , H. , Yoshida , T. , To-o , K. , Kuriki , T. and Takahashi , K. 2004 . Reduced immunogenicity of β-lactoglobulin by conjugation with acidic oligosaccharides . Journal of Agricultural and Food Chemistry , 52 : 4546 – 4553 .
- Hattori , M. , Nagasawa , K. , Ohgata , K. , Sone , N. , Fukuda , A. , Matsuda , H. and Takahashi , K. 2000 . Reduced immunogenicity of β-lactoglobulin by conjugation with carboxymethyl dextran . Bioconjugate Chemistry , 11 : 84 – 93 .
- Hill , D.J. and Hosking , C.S. 1996 . Cow milk allergy in infancy and early childhood . Clinical and Experimental Allergy , 26 : 243 – 246 .
- Hill , D.J. and Hosking , C.S. 1997 . Emerging disease profiles in infants and young children with food allergy . Pediatric Allergy and Immunology , 8 : 21 – 26 .
- Jimenez-Castano , L. , Villamiel , M. , Martin-Alvarez , P.J. , Olano , A. and Lopez-Fandino , R. 2005 . Effect of the dry-heating conditions on the glycosylation of beta-lactoglobulin with dextran through the Maillard reaction . Food Hydrocolloids , 19 : 831 – 837 .
- Kleber , N. , Maier , S. and Hinrichs , J. 2007 . Antigenic response of bovine β-lactoglobulin influenced by ultra-high pressure treatment and temperature . Innovative Food Science and Emerging Technologies , 8 : 39 – 45 .
- Morgan , F. , Bouhallab , S. , Mollé , D. , Henry , G. , Maubois , J.L. and Léonil , J. 1998 . Lactolation of β-lactoglobulin monitored by electrospray ionization mass spectrometry . International Dairy Journal , 7 : 299 – 303 .
- Miralles , B. , Martínez-Rodríguez , A. , Santiago , A. , van de Lagemaat , J. and Heras , A. 2007 . The occurrence of a Maillard-type protein-ploysaccharide reaction between β-lactoglobulin and chitosan . Food Chemistry , 100 : 1071 – 1075 .
- Nacka , F. , Chobert , J.M. , Burova , T. , Leonil , J. and Haertle , T. 1998 . Induction of new physicochemical and functional properties by the glycosylation of whey proteins . Journal of Protein Chemistry , 17 : 495 – 503 .
- Nakamura , S. , Suzuki , Y. , Ishikawa , E. , Yakushi , T. , Jing , H. , Miyamoto , T. and Hashizume , K. 2008 . Reduction of in vitro allergenicity of buckwheat Fag e 1 through the Maillard-type glycosylation with polysaccharides . Food Chemistry , 109 : 538 – 545 .
- Palasota , J.A. and Deming , S.N. 1992 . Central composite experimental designs (applied to chemical systems) . Journal of Chemistry Education , 69 : 560 – 563 .
- Reddy , M. , Kella , N.K.D. and Kinsella , J.E. 1988 . Structural and conformational basis of the resistance of β-lactoglobulin to peptic and chymotryptic digestion . Journal of Agricultural and Food Chemistry , 36 : 737 – 741 .
- Sharma , S. , Pravindra , K. , Betzel , C. and Singh , T.P. 2001 . Structure and function of proteins involved in milk allergies . Journal of Chromatograph B , 756 : 183 – 187 .
- Spies , J. 1973 . Milk allergy . Journal of Milk Food Technology , 36 : 225 – 231 .
- van de Lagemaat , J. , Silván , J.M. , Moreno , F.J. , Olano , A. and del Castillo , M.D. 2007 . In vitro glycation and antigenicity of soy proteins . Food Research International , 40 : 153 – 160 .
- van der Ven , C. , Gruppen , H. , de Bont , D.B.A. and Voragen , A.G.J. 2002 . Optimisation of the angiotensin converting enzyme inhibition by whey protein hydrolysates using response surface methodology . International Dairy Journal , 12 : 813 – 820 .
- Wal , J.M. 2001 . Structure and function of milk allergens . Allergy , 56 : 35 – 38 .
- Wilson , S. , Blaschek , K. and Gonzalez de Mejia , E. 2005 . Allergenic proteins in soybean: Processing and reduction of P34 Allergenicity . Nutrition Reviews , 63 : 47 – 58 .
- Wróblewska , B. and Jedrychowski , L. 2002 . Effect of conjugation of cow milk whey protein with polyethylene glycol on changes in their immunoreactive and allergic properties . Food and Agricultural Immunology , 14 : 155 – 162 .