Abstract
The present study was to compare the stability and immunoreactivity of glycinin and β-conglycinin to hydrolysis with pepsin, trypsin or cooperation of the two enzymes for different time intervals (0.5, 1, 15, 30, 60 and 120 min) at different ratios of enzyme/substrate (1:100, 1:10, 1:1 and 10:1) in vitro. The results showed that the immunoreactivity was positively related with stability of glycinin (r=0.776, P<0.05) and β-conglycinin (r=0.851, P<0.05). B polypeptide chain of glycinin was resistant to hydrolysis with trypsin, and β subunit of β-conlycinin was not liable to hydrolysis with pepsin. The two above proteins were little or not affected by incubation time and the enzyme/substrate ratio, while others' hydrolysed degree got higher with prolonging incubation time, and the hydrolysis accelerated with increasing enzyme/substrate ratio. Our results indicated digestive enzyme, incubation time and enzyme/substrate ratio had effects on stability and immunoreactivity of allergenic proteins. The most effective hydrolysis was cooperation of the two enzymes for glycinin and trypsin for β-conglycinin.
Introduction
Soybeans are widely utilised in the food and feed industries due to their high nutritional value (Friedman & Brandon, Citation2001). However, at least 21 allergenic proteins in soybean have been identified and presented IgE binding (Babiker, Hiroyuki, Matsudom, Iwata, & Ogawaet, Citation1998). Among them are glycinin and β-conglycinin, which as two major allergens have negative effects on humans (Burks, Brooks, & Sampson, Citation1988; Duke, Citation1934; Foucard & Yman, Citation1999) and animals (Guo, Piao, Cao, Ou, & Li, Citation2008; Sun, Li, Dong, Qiao, & Ma, Citation2008; Sun, Li, Li, Dong, & Wang, Citation2008; Zhao et al., Citation2008). Glycinin, a molecular weight about 360 kDa, is a hexamer consisting of both acidic (A) and basic (B) polypeptide chains linked by a single disulfide bond with molecular weight of 34–44 kDa and 20 kDa, respectively (Kitamura & Shibasaki, Citation1975). β-conglycinin has a trimeric structure with a molecular weight of about 180 kDa and is composed of three subunits, α′, α and β, with a molecular weight of 58–77, 58–83 and 42–53 kDa, respectively (Maruyama et al., Citation2001).
To date, some light had been shed on the digestion of glycinin and β-conglycinin in calves (Lalles et al., Citation1999), rats (Perez, Millis, Lambert, Johnson, & Morgan, Citation2000) and piglets (Zhao et al., Citation2008). Although these works indicated that soybean allergens were resistant to digestion in the gastrointestinal tract, the effects of incubation time and the ratio of enzyme/protein on hydrolysis of glycinin and β-conglycinin are not clear. However, the methods in vitro may meet with the requirement which could design different incubation time and ratios of enzyme/substrate for protein hydrolysis. And the studies in vitro may avoid the trouble of separating the individual globulins in a mixture and the disturbance from other ingredients when the analysis of protein composition was made. Moreover, immunoreactivity variance of glycinin and β-conglycinin in hydrolysed fluid are nonexistent, to the best of our knowledge. In addition, hydrolysis of soybean allergens in simulated gastrointestinal fluid for humans was conducted (Astwood, Leach, & Fuchs, Citation1996; Fu, Abbot, & Hatzos, Citation2002). Piglets suffer soybean-induced allergy, but similar reports for pigs were scarce.
In this study, purified glycinin and β-conglycinin were subjected to hydrolysis with pepsin (porcine), trypsin (porcine) or cooperation of the two enzymes for different time at different ratios of enzyme/substrate in vitro. The stability and immunoreactivity of glycinin and β-conglycinin after hydrolysis were compared. Our work may provide further information on the hydrolysis of glycinin or β-conglycinin, which is an essential basis to prepare food/feed for humans or animals.
Materials and methods
Preparation of glycinin and β-conglycinin
Glycinin was isolated by hydroxyapatite-Ultrogel chromatography of a cryo-precipitated soya extract according to the method of Eldridge and Wolf (Citation1967). β-conglycinin was isolated from defatted soy flour by the method of Setsuko and Fumio (Citation1987). Protein content was determined by the Kjeldahl method and purity was analysed by sodium dodecyl sulfate polyacrylamide gel electrophoresis (SDS-PAGE) followed by Coomassie Brilliant Blue (CBB) R-250 staining (Laemmli, Citation1970). The gel was scanned by Bio Imaging System with Gene Genius (Syngene, USA) and the purity of glycinin was measured by GeneTools Analysis Software, Version 3.03.03 (Guo, Piao, Ou, Li, & Hao, Citation2007).
Production of polyclonal antibodies
Glycinin and β-conglycinin (over 95% purity) as immunogens were used to produce polyclonal antibodies according to He, Hu, and Gao (Citation2002). Polyclonal antibodies were further purified by affinity chromatography through a 1.0×10 cm Sepharose 4B (Amersham Pharmacia Biotech AB) column.
Enzymatic hydrolysis of glycinin and β-conglycinin in vitro
The method proposed by Boisen and Fermández (Citation1995) and Fu et al. (Citation2002) for the determination of protein digestibility in vitro was applied with some modifications as follows.
Pepsin hydrolysis
Glycinin and β-conglycinin (5 mg ml−1, 0.2 ml) were dissolved in phosphate buffer saline (PBS, 0.1 M, pH 6.0) and mixed by gently stirring. The pH was adjusted to 2.0 with 2 N HCl. 20 µL pepsin (Sigma P-7000) solution (0.5, 5, 50 and 500 mg pepsin ml−1, 0.03M pH=2 NaCl) were added to the sample buffer in order that the ration of enzyme to substrate was different (1:100, 1:10, 1:1 and 10:1). Then the mixtures were placed on multi-point stirrers in a thermostatic-controlled heating chamber at 37°C and the samples were stirred gently for 0.5, 1, 15, 30, 60 and 120 min. At the end of regular time intervals, pH was adjusted to 7.0 to stop the reaction by 1 N NaOH.
Trypsin hydrolysis
Glycinin and β-conglycinin (5 mg ml−1, 0.2 ml) were dissolved in phosphate buffer (0.2 M, pH 6.8) and mixed by gently stirring. The pH was adjusted to 6.8 with 1 N NaOH. 20 µL trypsin (Amresco 0458) solution (0.5, 5, 50 and 500 mg trypsin ml−1, 0.05 M pH=6.8 PBS) were added to the sample buffer in order that the ration of enzyme to substrate was different (1:100, 1:10, 1:1 and 10:1). Then the mixture was placed on multi-point stirrers in a thermostatic-controlled heating chamber at 37°C and the samples were stirred gently for 0.5, 1, 15, 30, 60 and 120 min. At the end of regular time intervals, trypsin was inactivated by heating (80°C) for 5 min.
Cooperation hydrolysis with pepsin and trypsin
Glycinin and β-conglycinin (5 mg ml−1, 0.2 ml) were dissolved in phosphate buffer (0.1 M, pH 6.0) and mixed by gently stirring. The pH was adjusted to 2.0 with 2 N HCl. 20 µL pepsin (Sigma P-7000) solution (0.5, 5, 50 and 500 mg pepsin mL−1, 0.03 M pH=2 NaCl) were added to the sample buffer in order that the ration of enzyme to substrate was different (1:100, 1:10, 1:1 and 10:1). The mixture were placed on multi-point stirrers in a thermostatic-controlled heating chamber at 37°C and the samples were stirred gently for 0.5, 1, 15, 30, 60 and 120 min. The pH was adjusted to 6.8 with 1 N NaOH. 20 µL trypsin (Amresco 0458) solution (0.5, 5, 50 and 500 mg trypsin m−1, 0.05 M pH=6.8 PBS) were added to the sample buffer in order that the ratio of enzyme to substrate was different (1:100, 1:10, 1:1 and 10:1). Then the mixture were placed on multi-point stirrers in a thermostatic-controlled heating chamber at 37°C and the samples were stirred gently for 0.5, 1, 15, 30, 60 and 120 min. At the end of regular time intervals, trypsin was inactivated by heating at 80°C for 5 min.
In addition, control solution without tested proteins or digestive enzymes were performed (reaction time zero) as the above description.
Glycinin and β-conglycinin stability assays
All the final hydrolysis samples were subjected to SDS-PAGE by using minigel apparatus (Bio-Rad Laboratories, CA, USA) with a 12% acrylamide separating and a 5% acrylamide stacking gel according to Laemmli (Citation1970) with slight modification. The samples were dissolved in 1 M Tris-HCl buffer (containing 2.7 M glycerol, 0.15 M SDS and 0.15 mM bromophenol blue, and pH 6.8). The loading was 10µg of protein per well for pure glycinin or β-conglycinin. Molecular weight markers were also loaded in a separate well. Electrophoresis was performed at 100 V for separating gel and at 200 V for stacking gel. Proteins were visualised by CBB staining.
To determine the protein stability, the band of native proteins and hydrolysed products were quantified by a densitometry using Glyko Bandscan software (Glyko, Novato, CA) (Yi et al., Citation2005; Zuo et al., Citation2007). The percentage of band intensities for every sample with three replicates versus the standard were defined as tested protein stability, considering the standard (the samples of the glycinin or β-conglycinin without enzymes) as 100% (Calinescu, Nadeau, Mulhbacher, Fairbrother, & Mateescu, Citation2007).
Immunoreactive glycinin and β-conglycinin stability assays
Immunoreactivity of part final hydrolysis samples (the ratio of enzyme to substrate=1:100) were analysed using inhibition enzyme-linked immunosorbent assay (ELISA) procedures. Immunoassay procedures and calibration curves of immunoreactive glycinin or β-conglycinin were determined as described in our previous study (Zhao et al., Citation2008). Immunoreactive stability of glycinin and β-conglycinin were calculated as the percentage of immunoreactivity for every hydrolysis sample versus the native protein.
Statistical analysis
All data were analysed by using the general linear model procedure of Statistical Package for Social Sciences version 11.5 (SPSS Inc., Chicago, IL, USA). The results of immunoreactivity were expressed as the mean values ± standard deviation (SD). Differences among means were tested by using Duncan's multiple range tests. Statements of statistical significance were based upon P≤0.05. Correlation analyses were performed by Pearson's correlation coefficient.
Results
Quality check of glycinin and β-conglycinin
Determined by the Kjeldahl method and SDS-PAGE analysis, glycinin and β-conglycinin purified through Sepharose CL-6B column were judged to be over 95%.
Comparative hydrolysed stability of different protein with the same enzyme
shows a typical SDS-PAGE analysis of protein degradation with pepsin, trypsin or cooperation of the two enzymes. summarises the hydrolysed stability of glycinin and β-conglycinin. Both pepsin and trypsin were of evident specificity. Although pepsin could hydrolyse α, α′ subunits of β-conglycinin, A and B polypeptide chains of glycinin, β subunit of β-conlycinin could not be effectively hydrolysed. However, trypsin could make β subunit of β-conlycinin be hydrolysed well, and it could not hydrolyse B polypeptide chain of glycinin. After hydrolysis with cooperation of the two enzymes, the stability of glycinin and β-conglycinin decreased significantly, especially for A polypeptide chain of glycinin (P<0.05), decomposing within 0.5 min. Hydrolysis of β subunit of β-conglycinin with trypsin or cooperation of the two enzymes produced hydrolysates with molecular weight of 20–29 kDa.
Figure 1. Enzyme hydrolysed analysis of glycinin and β-conglycinin (enzyme/protein=1:100). SDS-PAGE and Coomassie Brilliant Blue staining are used to assess hydrolysis of glycinin (panels A–C) and β-conglycinin (panels D–F) with different enzymes. Molecular weight markers (lane M) are indicated on the left-hand side of the gels (units: kD). Enzyme and allergen proteins are run alone (lanes I and P). Allergens are incubated with enzymes for times indicated above the gels.
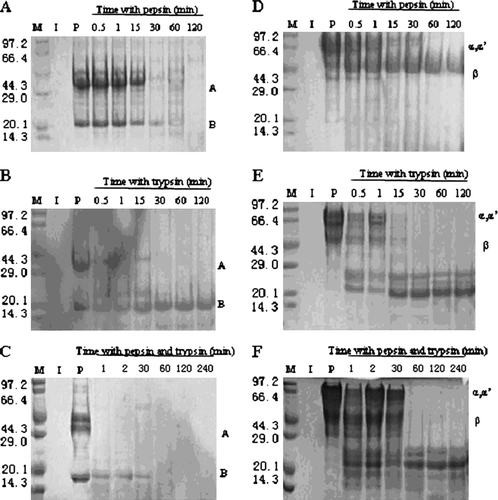
Comparative hydrolysed stability of the same protein with different enzymes
When the allergenic proteins were incubated with pepsin, trypsin or cooperation of the two enzymes, the most effective treatment was the two enzyme cooperation for glycinin, and trypsin for β-conglycinin () (P<0.05). The hydrolysed stability of β-conglycinin with pepsin was the highest (P<0.05), particularly for β subunit of β-conglycinin which was almost not affected by pepsin (98.11%). The stability of A polypeptide chain of glycinin hydrolysed with trypsin was 40%, which lied between the two enzymes cooperation and pepsin. On the other hand, B polypeptide chain of glycinin exhibited high hydrolysed stability with trypsin (98.71%). The above data was under the condition with the pepsin/protein ratio of 1:100, and other ratios had the similar rules.
Effects of incubation time on the hydrolysed stability
With prolonging incubation time, the hydrolysis extent of most substrates got higher ( and ). However, B polypeptide chain of glycinin hydrolysed with trypsin and β subunit of β-conglycinin hydrolysed with pepsin still existed after 120 min. Limited hydrolysis of β subunit of β-conglycinin was found with pepsin and incubation time have no significant effects on their stability (P>0.05). When hydrolysis with trypsin increased to certain degree, the stability of B polypeptide chain of glycinin could not increase with prolonging incubation time.
Effects of the ratio of enzyme/substrate on the hydrolysed stability
The enzyme/substrate ratio had great effects on the protein hydrolysis shown in . With the content of enzyme increasing, the protein hydrolysis accelerated except for β subunit of β-conglycinin hydrolysed with pepsin. Even if raising the concentration of pepsin, β subunit of β-conglycinin could not be hydrolysed, due to their resistance of hydrolysis with pepsin.
Comparitive immunoreactivity of glycinin or β-conglycinin
There was enzyme–time interaction for the immunoreactivity of glycinin and β-conglycinin hydrolysed with digestive enzymes shown in (P<0.001). Regarding to the variation of immunoreactivity, glycinin was more sensitive to pepsin, but β-conglycinin was more liable to trypsin. Immunoreactivity of glycinin hydrolysed with trypsin and β-conglycinin hydrolysed with pepsin still existed after 120 min, while the immunoreactivity of glycinin and β-conglycinin nearly vanished after 15–30 min in other hydrolysed fluid. Comparing with glycinin, β-conglycinin hydrolysed more slowly with cooperation of pepsin and trypsin, exhibiting 27.64% immunoreactive protein after 30 min.
Table 1. Immunoreactive stability of glycinin and β-conglycinin hydrolysed with different enzymes.
Correlations between protein stability and immunoreactivity
Strong correlations between the variations of immunoreactivity and the hydrolysed extent of glycinin and β-conglycinin were found in this study. The immunoreactivity of glycinin and β-conglycinin were highly and positively correlated (P<0.001) with the protein stability (glycinin: r=0.776; β-conglycinin: r=0.851) and the stability of their polypeptide chains (A chain: r=0.709; B chain: r=0.791) or their subunits (α,α′ subunits: r=0.700, β subunit: r=0.766).
Discussion
In this study, the polypeptides of glycinin and subunits of β-conglycinin after hydrolysis were analysed through the protein bands in gels. The stability of glycinin and β-conglycinin were expressed by the variation of their respective polypeptides and subunits.
An important finding of the current study is that B-chain of glycinin appeared to be resistant to hydrolysis with trypsin. Under the condition of neutral and slightly acid pH, glycinin has a strong tendency to aggregate upon hydrolysis by various proteases, but there is a strong substrate dependency for the aggregation of proteins upon enzymatic hydrolysis due to glycinin does not aggregate upon hydrolysis with trypsin (Kuipers Bas, Alting Arno, & Gruppen, Citation2007). This might ensure the premise of hydrolysis. Trypsin has a well-defined specificity, cleaving next to the hydrophilic amino acids (Barrett, Rawlings, & Woessner, Citation2004) or basic amino acid residues (Braudo et al., Citation2006) at the P1 position. Based on the prediction of the secondary structure of polypeptide chains (Bassüner & Finkelstein, Citation1986) and the data of the small-angle X-ray analysis (Plietz, Drescher, & Damaschun, Citation1987), it was suggested that the B-chains are buried in the interior, while the A-chains and especially their disordered segment are located in the outer part of macromolecules. This conclusion was definitely confirmed by the X-ray determination of the 3D structure of A1aB1b and A3B4 subunits of glycinin (Adachi, Takenaka, Gidamis, Mikami, & Utsumi, Citation2001; Adachi et al., Citation2003). Due to high content of basic amino acid residues, A-chains and especially their disordered C-terminal segment, located in the outer part of macromolecules, are especially susceptible to trypsin action (Braudo et al., Citation2006). The results in this study were consistent with those reports. To date, previous reports about the hydrolysis of B-chains with trypsin in vitro are nonexistent to the best of our knowledge. The limited hydrolysis of B-chain with trypsin in this work is maybe linked with interior structure of glycinin. For trypsin the total number of cleavage sites for β-conglycinin is higher than glycinin (Kuiper et al., Citation2007). This can easily explain the difference of hydrolysed degree between glycinin and β-conglycinin with trypsin.
Consistent with the results of Astwood (Citation1996) and Fu et al. (Citation2002), our data also indicated that β subunit of β-conglycinin were stable to pepsin, even if the pepsin/substrate ratio increased to 1000 times at 120 min.
Our results indicated that the hydrolysis degree increased with prolonging time. Most of glycinin and β-conglycinin disappeared within 120 min, except for β subunit of β-conglycinin with pepsin hydrolysis and B-chain of glycinin with trypsin hydrolysis. With the pepsin/substrate ratio enhancing, the hydrolysis accelerated and the time of complete proteolysis shortened in this work, which was consistent with the results of Fu et al. (Citation2002).
It is not easy to choose an optimal enzyme/substrate ratio, since humans/pigs show a wide variation in their gastric and pancreatic secretions, and it also varies with type of feed consumed. Boisen and Fernández (Citation1995) used the method in vitro simulating the protein digestive process of pigs to evaluate the protein digestibility, in which the pepsin/substrate and trypsin/substrate ratio was 1:100 and 1:20, respectively. Several enzyme/substrate ratios were selected in this study to determine whether the enzyme/substrate ratio has effect on the hydrolysed extent of soybean antigens. The apparent susceptibility of allergens to proteolysis is strongly dependent on the pepsin/allergen ratio (Javier Moreno, Citation2007). This conclusion was consistent with several reports (Fu et al., Citation2002; Takagi, Teshima, Okunuki, & Sawada, Citation2003; Venkatachalam, Teuber, Peterson, Roux, & Sathe, 2004). With the pepsin/substrate ratio increasing, the time of completing hydrolysis shortened for ovomucoid (OVM) (Takagi et al., Citation2003). Hydrolysis of glycinin and β-conglycinin with pepsin, trypsin or cooperation of the two enzymes had similar results in our study except for β subunit of β-conglycinin resistant to hydrolysis with pepsin.
The present work clearly demonstrates that the great difference for hydrolysis of glycinin and β-conglycinin with pepsin, trypsin or cooperation of the two enzymes. β-conglycinin was poorly hydrolysed with pepsin, consistent with the results of Astwood et al. (Citation1996). Compared with glycinin (pI=6.4), hydrolysed pH with pepsin is closer to the pI of β-conglycinin (pI=4.9), which was maybe a reason of leading to poor hydrolysis of β-conglycinin. Similar to hydrolysed degree of the intact protein with trypsin, immunoreactive glycinin were more stable than β-conglycinin, as the total number of cleavage sites to trypsin for β-conglycinin were higher than glycinin (Kuipers Bas et al., Citation2007).
The positive correlation between immunoreactive stability and protein stability of glycinin or β-conglycinin shows the strong link between immunoreactivity and protein. Moreover, the polypeptides and subunits of glycinin, β-conglycinin and their hydrolysed products have immunoreactivity.
Conclusion
This study demonstrated that digestive enzymes, incubation time and enzyme/substrate ratios had different effects on the stability and immunoreactivity of allergenic proteins, and the immunoreactivity was positively related with hydrolysed extent of glycinin and β-conglycinin. The most effective treatment was the two enzyme cooperation for glycinin and trypsin for β-conglycinin. B-polypeptide chain of glycinin was resistant to hydrolysis with trypsin, and β-subunit of β-conlycinin was not liable to hydrolysis with pepsin. The two above proteins were almost little or not affected by incubation time and the enzyme/substrate ratio, while others’ hydrolysed degree got higher with prolonging incubation time, and the hydrolysis accelerated with increasing enzyme/substrate ratio.
Acknowledgements
This work was financially supported by the National Natural Science Foundation of P.R. China (Grant No. 30430520).
References
- Adachi , M. , Kanamori , J. , Masuda , T. , Yagasaki , K. , Kitamura , K. , Mikami , B. and Utsumi , S. 2003 . Crystal structure of soybean 11S globulin: Glycinin A3B4 homohexamer . Proceedings of the National Academy of Sciences , 100 : 7395 – 7400 .
- Adachi , M. , Takenaka , Y. , Gidamis , AB. , Mikami , B. and Utsumi , S. 2001 . Crystal structure of soybean proglycinin. A1aB1b homotrimer . Journal of Molecular Biology , 305 : 291 – 305 .
- Astwood , J.D. , Leach , J.N. and Fuchs , R.L. 1996 . Stability of food allergens to digestion in vitro . Nature Biotechnology , 14 : 1269 – 1273 .
- Babiker , E.E. , Hiroyuki , A. , Matsudom , N. , Iwata , H. and Ogawaet , T. 1998 . Effect of polysaccharide conjugation or transglutaminase treatment on the allergenicity and functional properties of soy protein . Journal of Agriculture and Food Chemistry , 46 : 866 – 871 .
- Barrett , A.J. , Rawlings , N.D. , Woessner , J.F. 2004 Handbook of proteolytic enzymes , 2nd ed London : Elsevier Academic Press .
- Bassüner , R. and Finkelstein , A.V. 1986 . Proceedings of the 2nd international symposium on self-regulation of plant metabolism , 54 Sofia, , Bulgaria : Bulgarian Academy of Sciences .
- Boisen , S. and Fernández , J.A. 1995 . Prediction of the apparent ileal digestibility of protein and amino acids in feedstuffs and feed mixtures for pigs by in vitro analyses . Animal Feed Science and Technology , 51 : 29 – 43 .
- Braudo , E.E. , Danilenko , A.N. , Guslyannikov , P.V. , Kozhevnikov , G.O. , Artykova , G.P. , Lapteva , N.A. , Vaintraub , N.A. , Sironi , E. and Duranti , M. 2006 . Comparative effects of limited tryptic hydrolysis on physicochemical and structural features of seed 11S globulins . International Journal of Biological Macromolecules , 39 : 174 – 178 .
- Burks , A.W. , Brooks , J.R. and Sampson , H.A. 1988 . Allergenicity of major component proteins of soybean determined by enzyme linked immunosorbent assay (ELISA) and immunoblotting in children with atopic dermatitis and positive soy challenges . Journal of Allergy and Clinical Immunology , 81 : 1135 – 1142 .
- Calinescu , C. , Nadeau , É. , Mulhbacher , J. , Fairbrother , J.M. and Mateescu , M-A. 2007 . Carboxymethy1 high amylase starch for F4 fimbriae gastro-resistant oral formulation . International Journal of Pharmaceutics , 343 : 18 – 25 .
- Duke , W.W. 1934 . Soybean as a possible important source of allergy . Journal of Allergy , 5 : 300 – 302 .
- Eldridge , A.C. and Wolf , W.J. 1967 . Purication of the 11S component of soybean protein . Cereal Chemistry , 44 : 645 – 668 .
- Foucard , T. and Yman , I.M. 1999 . A study on severe food reactions in Sweden – is soy protein an underestimated cause of food anaphylaxis? . Allergy , 54 : 261 – 265 .
- Friedman , M. and Brandon , D.L. 2001 . Nutritional and health benefits of soy proteins . Journal of Agriculture and Food Chemistry , 49 : 1069 – 1077 .
- Fu , T-J. , Abbott , U.R. and Hatzos , C. 2002 . Digestibility of food allergens and non-allergenic proteins in simulated gastric fluid and simulated intestinal fluid—a comparative study . Journal of Agriculture and Food Chemistry , 50 : 7154 – 7160 .
- Guo , P.F. , Piao , X.S. , Cao , Y.H. , Ou , D.Y. and Li , D.F. 2008 . Recombinant soybean protein β-conglycinin α′-subunit expression and induced hypersensitivity reaction in rats . International Archives of Allergy and Immunology , 145 : 102 – 110 .
- Guo , P.F. , Piao , X.S. , Ou , D.Y. , Li , D.F. and Hao , Y. 2007 . Characterization of the antigenic specificity of soybean protein β-conglycinin and its effects on growth and immune function in rats . Archives of Animal Nutrition , 61 : 189 – 200 .
- He , Z. , Hu , G. and Gao , Y. 2002 . “ Polyclone antibodies preparation technique ” . In Technique of animal immunology , Edited by: He , Z. , Hu , G. and Wang , C. 71 – 76 . Changchun : Jilin Science and Technology Publishing House .
- Javier Moreno , F. 2007 . Gastrointestinal digestion of food allergens: Effect on their allergenicity . Biomedicine & Pharmacotherapy , 61 : 50 – 60 .
- Kitamura , K. and Shibasaki , K. 1975 . Isolation and some physicochemical properties of acidic subunits of soyabean 11S globulin . Agricultural Biology and Chemistry , 39 : 945 – 951 .
- Kuipers Bas , J.H. , Alting Arno , C. and Gruppen , H. 2007 . Comparison of the aggregation behavior of soy and bovine whey protein hydrolysates . Biotechnology Advances , 25 : 606 – 610 .
- Laemmli , U.K. 1970 . Cleavage of structural proteins during the assembly of the head of bacteriophage T4 . Nature , 227 : 680 – 685 .
- Lalles , J.P. , Tukur , H.M. , Salgado , P. , Mills , ENC. , Morgan , M.R.A. and Quilien , L. 1999 . Immunochemical studies on gastric and intestinal digestion of soybean glycinin and β-conglycinin in vivo . Journal of Agricultural Food and Chemistry , 47 : 2797 – 2806 .
- Maruyama , N. , Adachi , M. , Takahashi , K. , Yagasaki , K. , Kohno , M. , Takenaka , Y. , Okuda , E. , Nakagawa , S. , Mikami , B. and Utsumi , S. 2001 . Crystal structures of recombinant and native soybean β-conglycinin β homotrimers . European Journal of Biochemistry , 268 : 3595 – 3604 .
- Perez , M.D. , Mills , E.N.C. , Lambert , N. , Johnson , I.T. and Morgan , M.R.A. 2000 . The use of anti-soya globulin antisera in investigating soya digestion in vivo . Journal of the Science of Food and Agriculture , 80 : 513 – 521 .
- Plietz , P. , Drescher , B. and Damaschun , G. 1987 . Relationship between the amino acid sequence and the domain structure of the subunits of the 11S seed globulins . International Journal of Biological Macromolecules , 9 : 161 – 165 .
- Setsuko , I. and Fumio , Y. 1987 . Determination of glycinin and β-conglycinin in soybean protein by immunological methods . Journal of Agricultural Food and Chemistry , 35 : 200 – 205 .
- Sun , P. , Li , D. , Dong , B. , Qiao , S. and Ma , X. 2008 . Effects of soybean glycinin on performance and immune function in early weaned pigs . Archives of Animal Nutrition , 4 : 313 – 321 .
- Sun , P. , Li , D. , Li , Z. , Dong , B. and Wang , F. 2008 . Effects of glycinin on IgE-mediated increase of mast cell numbers and histamine release in the small intestine . Journal of Nutrition Biochemistry , 19 : 627 – 633 .
- Takagi , K. , Teshima , R. , Okunuki , H. and Sawada , J-I. 2003 . Comparative study of in vitro digestibility of food proteins and effect of preheating on the digestion . Biological & Pharmaceutical Bulletin , 26 : 969 – 973 .
- Venkatachalam , M. , Teuber , S.S. , Peterson , W.R. , Roux , K.H. and Sathe , S.K. 2006 . Antigenic stability of pecan [Carya illinoinensis (Wangenh.) K. Koch] proteins: Effects of thermal treatments and in vitro digestion . Journal of Agricultral Food and Chemistry , 54 : 1449 – 1458 .
- Yi , K. , Guo , C. , Chen , D. , Zhao , B. , Yang , B. and Ren , H. 2005 . Cloning and functional characterization of a formin-like protein (AtFH8) from Arabidopsis . Plant Physiology , 6 ( 138 ) : 1071 – 1082 .
- Zhao , Y. , Qin , G. , Sun , Z. , Zhang , X. , Bao , N. , Wang , T. , Zhang , B. , Zhang , B. , Zhu , D. and Sun , L. 2008 . Disappearance of immunoreactive glycinin and β-conglycinin in the digestive tract of piglets . Archives of Animal Nutrition , 4 : 322 – 330 .
- Zuo , T. , Liu , R. , Zhang , H. , Chang , X. , Liu , Y. , Wang , L. , Zheng , P. and Liu , Y. 2007 . FOXP3 is a novel transcriptional repressor for the breast cancer oncogene SKP2 . Journal of Clinical Investigation , 117 : 3765 – 3773 .