Abstract
A laboratory ring trial was performed in four laboratories for determination of Cry1Ab toxin in leaf material of MON 810 maize using a standardised enzyme-linked immunoassay protocol. Statistical analysis was carried out by the ISO 5725-2 guidelines, sample standard deviation and standard error, within-laboratory and inter-laboratory SD and SE were calculated. Measured inter-laboratory average values were 12.5±4.0, 15.3±4.6 and 72.6±17.8 µg/g for three lyophilised samples, and 27.8±4.3 µg/g for a frozen sample, yet, Cry1Ab concentrations ranged 66.5–160.1% of the corresponding IA. Determined concentrations by in-house protocols were statistically not different in one laboratory and different in two laboratories from the corresponding values by the joint protocol. Results emphasise the importance of a standardised protocol among laboratories for comparable quantitative Cry1Ab toxin determination. However, even when using a standardised protocol, significant differences still occur among toxin concentrations detected in different laboratories, although with a smaller range of variation.
Introduction
Insecticidal crystal (Cry) proteins of Bacillus thuringiensis (Bt) are entomopathogenic lectins (Höfte & Whiteley, Citation1989; Schnepf et al., Citation1998). These proteins act by specifically binding to membrane receptors in the insect midgut, and cause mortality through pore formation and subsequent sepsis (Bravo, Gill, & Soberón, Citation2007; Gill, Cowles, & Pietrantonio, Citation1992; Masson et al., Citation1998). Characteristic features in the mode of action are: (1) Cry toxin proteins from various Bt strains show insect specificity at family level and (2) bacterial Cry toxin proteins (protoxins) require enzymatic cleavage to generate their active form (activated toxin), mostly by trypsin-like insect gut enzymes (Andrews, Bibilos, & Bulla, Citation1985; Bravo, Sanchez, Kouskoura, & Crickmore, Citation2002; Díaz-Mendoza, Farinós, Castañera, Hernández-Crespoand, & Ortego, Citation2007; Lambert et al., Citation1996; Milne & Kaplan, Citation1993; Miranda, Zamudio, & Bravo, Citation2001; Mohan & Gujar, Citation2003; Oppert Citation1999; Shao et al., Citation1998). Besides this cleavage, the conformation of the Cry toxin proteins is also known to play a role in activation (Choma & Kaplan, Citation1990, Citation1991). Not all relevant details regarding the mode of action of Bt toxins are known. Open questions remain concerning the role of the receptors and other mechanisms that can lead to effects in target and non-target organisms (for overview see Pigott & Ellar, Citation2007; Soberón, Gill, & Bravo, Citation2009; Then Citation2010). Bacterial Cry protoxins have been used for plant protection purposes in spray formulations for several decades. In recent decades, with the advances in molecular biology and genetic engineering, it became possible to identify, isolate and transfer the genes encoding for the production of Cry proteins from the bacillus to plants. Today, numerous cultivars of such genetically modified (GM) crop plants, like Bt maize and Bt cotton, containing inserted transgenes that encode so-called pre-activated Cry toxins, are commercially available and grown on large areas primarily in North America and South America. Pre-activated toxins (truncated forms of the bacterial protoxins, yet larger in molecular size than the activated toxins) are produced in these plants (De Maagd, Bosch, & Stiekema, Citation1999; Hilbeck & Schmidt, Citation2006; Then, Citation2010). They have two major advantages in the development of transgenic cultivars: (1) their enhanced production level in transgenic plant cells (Barton & Miller, Citation1998) and (2) their patent right status, as these toxin proteins, unlike the natural protoxins, constitute the intellectual private property of the variety owner, i.e. primarily biotech companies. For the latter reason even naturally occurring Bt strains have been GM to produce truncated toxin (Park, Bideshi, & Federici, Citation2000). The production of the pre-activated toxin is significant as the Cry toxin is readily available as a pest defence in its activated form over the entire growing season of these Bt plants (i.e. constitutive expression). Thus, in comparison to the spray formulations the activity and the structure of the toxins in Bt crops are changed and the route of exposure to the environment is different. For risk assessment and quality control of Bt plants several specific questions emerge from this background. One important issue is the exact content of the Bt toxin and the range of its variation within the plants.
As a result of the commercial cultivation of Bt plants, analytical detection of Cry toxins became of great importance for quantifying toxin in insect resistant GM Bt plants for several reasons: quality control of the product (the GM Bt plant), regulatory compliance, resistance management and environmental risk assessment (ERA). (1) Quality control/regulatory compliance of the GM plants is essential for the customer, the farmer, and similar to that of pesticides. In order to ensure that the product, Bt maize, will indeed function effectively as indicated on the label, a minimum concentration of the pesticidal compound, the Cry toxin, needs to be expressed during the critical period of pest infestation. Currently, such minimum concentration of plant-expressed Bt toxin is unknown for most if not all target pests and therefore difficult to enforce. It relies entirely on the farmer's capacity to monitor its efficacy and report any detected control failure to the producer. Partly this is due to the lack of standardised, uniform detection procedures that would yield comparable results, which this project here addresses. (2) The current insect resistance management (IRM) strategy relies on two equally important components to delay resistance evolution in the target pest insects: a significant refuge and a high dose expression of the Cry toxins in the Bt plants (Gould Citation1998; McGaughey, Gould, & Gelernter, Citation1998). The refuge is meant to maintain a sufficiently large pool of homozygote susceptible individuals of the target pest species to dilute any potentially arising heterozygote resistant individuals due to the enormous selection pressure exerted by the Bt plants through its constitutive expression of Cry toxins. While refuge requirements such as a 5 or 20% area of a maize field grown to conventional non-Bt maize is relatively simple to monitor and to enforce and lays entirely in the responsibility of the farmer (Kruger, van Rensburg, & van den Berg, 2009), enforcing and monitoring the compliance with the high dose requirement is much more difficult and largely in the responsibility of the producer. This is where it also connects to the quality control/regulatory compliance issue mentioned above. Currently, there are no minimum concentrations known that would constitute a demonstrated lower limit of a high dose. The high dose is defined very vaguely as 25 times the concentration necessary to kill a homozygote susceptible individual (e.g. Gould, Citation2000), but it is not known what actual Cry toxin concentration this translates into. This again is partly due to the fact that no established, agreed and standardised enzyme-linked immunosorbent assay (ELISA) protocols exist that demonstrably yield comparable and reproducible results. (3) Reliable and, more importantly, reproducible ELISA protocols for quantification of Cry toxins are also essential for any exposure analysis, a critical element of the mandatory ERA procedure of Bt plants. The conclusions of any ERA will remain even more speculative, if the degree of exposure remains unclear and unreliable. While in laboratory experiments, under worst case scenarios with high doses of microbial Cry toxins, adverse effects can be observed on non-target insects (e.g. Dhillon & Sharma, Citation2009; Hilbeck, Baumgartner, Fried, & Bigler, Citation1998a; Hilbeck, Moar, Pusztai-Carey, Filipini, & Bigler, Citation1998b; Hilbeck, Moar, Pusztai-Carey, Filipini, & Bigler, Citation1999; Schmidt, Braun, Whitehouse, & Hilbeck, Citation2008), the ecological meaning of these finding remains to a great deal unclear since the actual expression levels of the Cry toxins in Bt plants are not determined in a reliable and comparable way. For example, Dively et al. (Citation2004) found effects in larvae of Monarch butterflies that did not follow the expected dose–effect relationship. It is a matter of speculation if these effects were caused by susceptibility of the larvae, the structure of the truncated protein in the plants, additional external factors or insufficient determination of the content of Bt toxin in the plant material (Then, Citation2010). Another example of showing the importance of exact and comparable determination of Bt toxin exposure by transgenic plants is Perry et al. (Citation2010) which propose a model to determine relevant exposure of Bt pollen around maize fields. Lang, Brunzel, Dolek, Otto, and Theißen (Citation2011) are pointing out that the model of Perry et al. (Citation2010) has major deficiencies amongst one is that the exact exposures of Bt toxin via pollen is not sufficiently determined. Moreover, Perry et al. (2010) erroneously assume that the sensitivity of non-target butterflies Inachis io and Vanessa atalanta to MON 810 pollen is the same, which questions the validity of their mathematical model. Another example to show the importance of exact measurement of Bt toxin is Icoz, Andow, Zwahlen, and Stotzky (2009) that tried to determine the uptake of Bt toxins by various plants after growing Bt maize. They were not able to come to final conclusions and were questioning the accuracy and sensitivity of the available commercial test kits.
The availability of the appropriate Cry toxin in its purified form is essential for the analytical determination of the toxin content in any matrix. Toxin purification from B. thuringiensis has been established (Bietlot et al., Citation1989), but described only in a few cases for GM plants (Gao et al., Citation2004, Citation2006). Cry proteins are detected and measured by several instrumental methods, including high-performance liquid chromatography (Yamamoto, Citation1983), ion chromatography (Wu, Xu, & Chen, Citation2001), capillary electrophoresis (Liu & Tzeng, Citation2001; Lung, Male, Mazza, Masson, & Broussau, Citation2004) or matrix-assisted laser desorption ionisation time of flight mass spectrometry (Ranasinghe & Akhurst, Citation2002) combined with proteomics (Nguyen & Russel, Citation2010). ELISA methods are the method of choice for the analytical determination of Cry type lectins (Grothaus et al., Citation2006; Huber-Lukac, Luthy, & Braun, Citation1983; Walschus, Witt, & Wittmann, Citation2002; Wang et al., Citation2007), but other immunoanalytical formats, for example, dot blot (Tapp & Stotzky, Citation1995), lateral flow immunostrips (Ermolli et al., Citation2006a), microsphere-based immunoassay (Ermolli et al., Citation2006b; Fantozzi et al., Citation2007) or immunomagnetic electrochemical sensor (Volpe, Ammid, Moscone, Occhigrossi, & Palleschi, Citation2006) have also been reported. ELISAs are widely used for Cry toxin detection in microbial preparations (Crespo et al., Citation2008; Takahashi et al., Citation1998) and in GM plants or food (Adamczyk, Adam, & Hardee, Citation2001; Baumgarte & Tebbe, Citation2005; Bruns & Abel, Citation2003; Chen et al., Citation2009; Chilcutt & Tabashnik, Citation2004; Douville et al., Citation2005; Ezequiel, Reggiardo, Vallejos, & Permingeat, Citation2006; Harwood, Wallin, & Obrycki, Citation2005; Margarit, Reggiardo, Vallejos, & Permingeat, Citation2006; Mendelsohn, Kough, Zigfridais, & Matthewsm, Citation2003; Nguyen & Jehle, Citation2007; Sims & Berberich, Citation1996; Stave, Citation1999, Citation2002; Xie & Shu, Citation2001; Zwahlen, Hilbeck, Gugerli, & Nentwig, Citation2003). Commercial ELISA kits are available and used frequently for detection of Cry toxins from B. thuringiensis or GM plants. A common problem, however, in reported Cry toxin concentrations in GM plants is the high variability among results from different laboratories, different cultivation sites, and sometimes even within the same GM plant variety at a single location (Then & Lorch, Citation2008). Moreover, Cry toxins are known to be produced in GM plants in a tissue- and time-specific manner (Nguyen & Jehle, Citation2007; Székács, Lauber, Juracsek, & Darvas, 2010a; Székács, Lauber, Takács, & Darvas, Citation2010b). Cry1Ab toxin content often scatter over wide ranges within a single survey, concerns regarding non-uniform expression levels of the transgene and plant-to-plant variation in Cry1Ab contents were raised (Nguyen & Jehle, Citation2007; Then & Lorch, Citation2008). Therefore, it is of essential importance to develop validated quantification methods and protocols that can be used in different laboratories and yield reproducible, comparable results, at least to the degree possible. Thus, inter-laboratory studies have been carried out using ELISA systems for protein CP4 EPSPS responsible for glyphosate tolerance in GM soybean (Lipp, Anklam, & Stave, Citation2000) and for Cry3Bb1 toxin causing insect resistance in MON 88017 maize (Nguyen et al., Citation2008).
The objective of the present study was to explore the feasibility of and to establish the need for standardised ELISA protocols for the purpose of Cry1 toxin quantification in GM maize; and to test whether the measured variability of the analytical results reported in the literature is due to the ELISA method, human or instrumentation error sources, sample reproducibility or plant variability. For this purpose, an inter-laboratory ring trial test was carried out with the participation of four different collaborating laboratories in Norway, Germany, Hungary and Switzerland that routinely perform ELISAs for Cry toxin determination in their respective research programmes.
Materials and methods
Activated Cry1Ab toxin prepared from Cry1Ab protoxin from B. thuringiensis subsp. kurstaki HD-1 expressed as a single gene product in Escherichia coli by enzymatic cleavage with trypsine and purified by HPLC was obtained in lyophilised form from Marianne Pusztai-Carey (Biochemistry Department, Case Western University, Cleveland, OH, USA) and was used as analytical standard throughout the study. Standards and a standardised analytical protocol for the joint method were provided and circulated by participating laboratories. Lots of the Cry1Ab standard were distributed by Lab 3. A series of pre-diluted calibrators was prepared by Lab 1 and provided to each laboratory. Standard curves were obtained with dilution series prepared in each laboratory from the standard solution and the pre-diluted calibrators. To eliminate possible matrix effects, the dilution was carried out in assay buffer and in diluted blank leaf extracts.
Samples
Four different Bt maize samples and two corresponding isogenic maize samples were prepared and dispatched to the participating laboratories: lyophilised or frozen homogenised leaf materials of MON 810 maize prepared by Labs 2 and 4 (). Samples A and D were the fifth leaf of maize plants at V9 phenological stage grown at standard greenhouse conditions. Samples F and L were the sixth leaf of maize plants at V6 phenological stage and the ear level of maize plants at R1 phenological stage grown at field conditions, respectively. Samples A, D and L were lyophilised and homogenised, sample F was dried to air dryness, frozen and homogenised. Consequently, determined Cry1Ab concentrations were expressed as microgram, Cry1Ab per gram dry weight of plant tissue (dry weight referring to lyophilised weight for samples A, D and L, and air dryness for sample F). The laboratories processed the distributed identical samples by a pre-defined standardised joint protocol (JP) and in three laboratories by in-house methods. Samples A and B were analysed in all laboratories, sample L in Lab 1, Lab 3 and Lab 4, sample F in Lab3 and Lab 4.
Table 1. Maize samples analysed in the study.
Instrumentation and methods
The Bt-Cry1Ab-1Ac ELISA kit (Agdia Inc., Elkhart, IN, USA; #PSP 06200) was used as reference analytical method in all participating laboratories, Bt-Cry1Ab/Ac ELISA kit (Abraxis Inc., Warminster, PA, USA) was also applied in Lab 4. summarises instrumentation and experimental setup in the participating laboratories. All laboratories used aqueous sample extraction methods and ELISAs for quantitative analysis of toxin content in the samples.
Table 2. Instrumentation and experimental setup in the participating laboratories.
Sample preparation
Plant samples and bacterial preparations were subjected to a standardised sample preparation protocol. Maize leaf materials (lyophilised or dried) were measured for mass (i.e. 20 mg [±1 mg] or 40 mg [±2 mg]), and phosphate-buffered saline containing Tween 20 detergent (PBST) buffer was added in a ratio of 8:1 (2.5 or 5 ml, respectively). Leaf samples were homogenised in extraction bags (Bioreba AG, Reinach, Switzerland; #SF 90002) or in mortar with pestle. Then 1 ml of the extract was transferred into low protein binding Eppendof tubes, and was centrifuged at 12.000 rpm for 10 min at room temperature. Plant extracts were used immediately upon homogenisation or were stored for measurement at 4°C up to 2 days. For longer storage freezing to –20°C was applied.
ELISA
Extracted samples were subjected to ELISA for quantitative toxin content determination. A standardised analytical protocol (JP) had been formulated with the use of the Bt-Cry1Ab-1Ac ELISA kit by Agdia, and was performed in all participating laboratories. In addition, laboratories also carried out their own protocols using modified sample preparation or a different ELISA kit. Lab 1 performed Agdia ELISA using bicarbonate-buffered saline containing Tween 20 detergent (BBST) buffer as extractant. Lab 3 performed Agdia ELISA with a modified sample preparation protocol. Lab 4 applied the Bt-Cry1Ab/Ac ELISA kit by Abraxis using linear and sigmoid curve fitting for calibration. The Cry1Ab analytical standard was applied in serial dilution series at 16 concentrations between 0 and 50 ng/ml. Samples were analysed in triplicates at least twice on different microtiter plates on different days, with separate calibrator sets involved.
Statistical analysis
Analytical data obtained in each laboratory were evaluated following the ISO 5725-2 guidelines for collaborative studies (International Organization for Standardization, 1994). Statistical analyses on Cry1Ab toxin concentrations were carried out in parallel for samples A, D, F and L. Type 1 outliers (extreme values within a laboratory) were identified based on reported raw data in ELISA using Grubb's test. Data were tested with a 95% confidence interval. An additional Grubb's test was applied to identify Type 2 outliers (extreme values in the mean of all laboratories for individual samples). After elimination of Type 2 outliers, means of data for all laboratories were calculated for each sample. The normality of data distribution was evaluated by the Shapiro–Wilk test. If experimental data sets showed normal distribution, one way analysis of variance (ANOVA) and Tukey test were carried out on the data sets at 0.01 significance level. In case of data sets of non-normal distribution, Kruskal–Wallis ANOVA by ranks was performed and data were compared to each other by multiple comparison for mean rank of all groups at 0.01 significance level. Statistical analyses were performed using software Statistica v. 6.1 (StatSoft, Inc., Tulsa, OK, USA). Sample standard deviation (SD), standard error (SE), within-laboratory standard deviations (WLSD), within-laboratory standard errors (WLSE), as well as inter-laboratory standard deviations (ILSD) and inter-laboratory standard errors (ILSE) were calculated for both raw data and after elimination of outliers. The bias refers to deviations from the grand averages established by this test after elimination of outliers. For the evaluation of laboratory performance, bias and WLSD were calculated as compared to grand averages. The bias is a measure for the overall trueness of the measurements (systematic errors) and the corresponding SD is used as a measure for the measurement precision. Data obtained by the standardised JP and in-house (own) protocol, where applied, were compared to each other by paired t-test at 0.01 significance level on the combined data set in the laboratory of the given sample.
Results and discussion
Performance of the ELISA methods on maize samples
Cry1Ab toxin concentration was determined in the leaf samples by a standardised analytical JP and by in-house (own) protocols. Leaf samples were chosen as reference samples because Cry1Ab toxin content is reportedly the highest in this plant tissue in MON 810 event Bt maize (Monsanto, Citation2002; Nguyen & Jehle, Citation2007; Székács et al., Citation2010a). The Cry1Ab-1Ac ELISA kit from Agdia is distributed as a qualitative test, allowing quantitative determination using user-supplied analytical standards. As activated (trypsin-cleaved) Cry1Ab protein was used as analytical standard, detected Cry1Ab concentrations referred to those of the activated toxin. In contrast, results of the quantitative Abraxis Cry1Ab ELISA kit, providing manufacturer-supplied Cry1Ab protoxin protein standards and Cry1Ab protoxin-specific antibodies were expressed as protoxin equivalent concentrations.
Having measured Cry1Ab concentrations in the distributed maize samples by different methods in the laboratories, comparison of the determined values was carried out in three steps: (1) evaluation of the analytical standard curves obtained in each laboratory with Cry1Ab standards in assay buffer and blank leaf extract, (2) comparison among Cry1Ab concentrations determined in different laboratories by the JP and (3) comparison between Cry1Ab concentrations determined in a given laboratory by the JP and their own protocol.
To carry out statistical analyses, the normality of the distribution of Cry1Ab concentrations detected in each the maize sample in each participating laboratory by each measurement protocol applied had to be determined. For this purpose, the Shapiro–Wilk test (Shapiro & Wilk, Citation1965) is widely advised as the most reliable test to prove non-normality in small to medium sized samples. Results of the Shapiro–Wilk test carried out in each data set (by each protocol, each laboratory and the inter-laboratory dataset using the standardised JP) after elimination of outliers are summarised in . The value of the Shapiro–Wilk test statistics (SW-W) and corresponding probability values (p) indicated normal distribution in the majority of the separate data sets (by laboratory, sample and protocol). In contrast, combined data sets (by sample in all laboratories by the JP) did not follow normal distribution.
Table 3. Analysis of normality of data distribution by the Shapiro–Wilk test by sample, laboratory and protocol.
Cry1Ab concentrations determined by the standardised joint protocol
Analytical standard curves were established using activated Cry1Ab standards at 15 concentrations between of 0.01 and 50 ng/ml with a blank control. Standard curves were measured in assay buffer and isogenic leaf extracts. The standard curves showed an upward sigmoid course typical for sandwich ELISA systems (). To compare standard curves obtained in several laboratories on different assay runs (microplates), sigmoid standard curves were plotted as optical densities (ODs) normalised between maximal (ODmax) and minimal (ODmin) values obtained on the same microplate as a function of the Cry1Ab toxin concentration applied. Thus, incidental differences in the actual peak and background ODs among different immunoassay runs were compensated and allowed direct comparison of assay performance. Because occasional decline was observed on the sigmoid standard curves at 50 ng/ml Cry1Ab toxin concentration, curves were normalised for the higher plateau OD values (ODmax) detected at 20 ng/ml analyte concentration. The sigmoid curves obtained in the different laboratories showed very close course to each other. Their differences were tested by one-way analysis of variance at three concentration levels at approximately 10, 50 and 80% (at 0.56, 4.4 and 10 ng/ml concentrations, respectively), and were found statistically not different from each other at each concentration level, indicating good reproducibility among the laboratories (). The C50 values calculated from the sigmoid curves in assay buffer were 3.19±0.23, 4.92±0.12, 4.01±0.1 and 3.98±0.17 ng/ml in Lab 1, Lab 2, Lab 3 and Lab 4, respectively. No matrix effect occurred when the standard curve was obtained in maize leaf extract diluted at least 1:10 ().
Figure 1. Analytical standard curves obtained in Lab 1 (▪, solid line), Lab 2 (•, dashed line), Lab 3 (
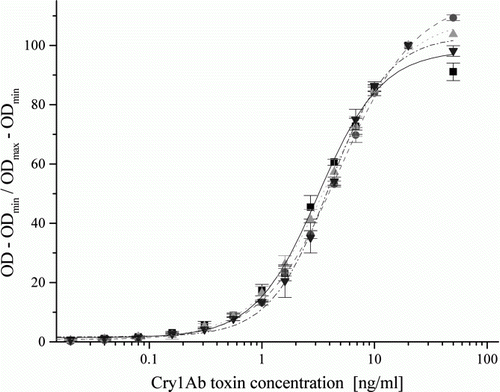
Figure 2. Analytical standard curves obtained in Lab 4 in buffer (▪, solid line) and in isogenic leaf extract (•, dashed line) in the standardised JP. Spiked concentrations of the Cry1Ab toxin standard were 0.01, 0.02, 0.04, 0.08, 0.16, 0.31, 0.56, 1, 1.6, 2.7, 4.4, 6.8, 10, 20 and 50 ng/ml.
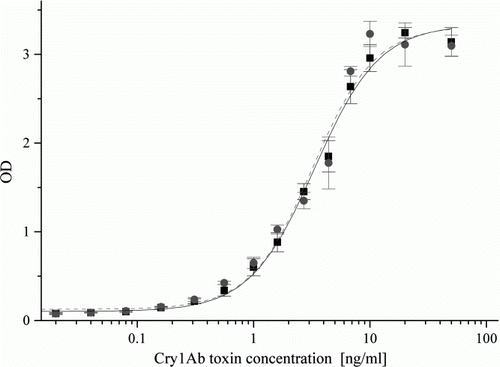
Cry1Ab toxin content determined in the maize samples in the participating laboratories by the JP, for example, the means of the detected Cry1Ab concentrations, their SDs and the deviation from the inter-laboratory average (IA) are summarised for all laboratories in . Deviations of detected Cry1Ab concentrations from the corresponding IA ranged from –33.5% (Lab 3) to +60.1% (Lab 4). In other words, extreme values of the detected concentrations ranged between two-thirds and over one and half times the average. This is also reflected by the statistical clustering of the concentration values: Cry1Ab concentrations detected for the same sample in different laboratories were often statistically different from each other, nonetheless, grouping of the data was not uniform. For sample A, Lab 1 and Lab 2 detected statistically similar concentrations, while Lab 3 and Lab 4 measured statistically significantly below and above, respectively. For sample D, all laboratories determined statistically different values. For sample F Lab 3 and Lab 4 detected statistically similar concentrations, while Lab 1 measured statistically significantly below. For sample L Lab 1 and Lab 3 detected statistically similar concentrations, while Lab 4 measured statistically significantly above ().
Table 4. Cry1Ab toxin concentrations in GM maize samples determined in different laboratories using the standardised JP.
lists sample standard deviations and errors (SD, SE, WLSD, WLSE, ILSD and ILSE) as calculated from individual determinations. The data illustrate different sources for the variation of the results. The influence of pipetting is indicated in the separate triplicate analyses, which are accurate in each laboratory, however, occasionally reaching as high as 10%. The SD explained for separate triplicate analyses range 0.4–7.0, 0–4.7, 0.3–10.5 and 0.1–7.7% for Labs 1 to 4, respectively. (The corresponding%SE values were 0.3–4.1, 0–2.7, 0.2–6.1 and 0.1–5.5%, respectively.) Plate-to-plate variability reflecting the performance of the entire protocol among different runs of the ELISA test (separate plates were performed separately), being always in the range of the corresponding triplicate variability, indicated good assay reproducibility in each laboratory, except for Lab 3 in the case of sample F. Within laboratory%SDs showed substantially higher variation ranging 0.6–14.5%, 6.1–6.4%, 9.9–16.8% and 3.6–8.5% for Labs 1 to 4, respectively (the corresponding percentage SE values ranged between 0.2 and 4.0%). This is certainly above the ±5% SD required for analytical measurements, but is within the range commonly seen for ELISA determinations. Especially Lab 3 ranged relatively high within laboratory variability. Because the corresponding variability of triplicates is low in Lab 3, the high within laboratory%SD originated from variation between different plates. Comparison of statistically evaluated data among laboratories provided inter-laboratory%SD values ranging between 15.5 and 31.6% (the corresponding%SE values ranged between 2.4 and 3.5%). The distribution of the SD and SE values indicated random error (as opposed of systematic error) as a source of inter-laboratory deviation.
Table 5. Relative SD and SE in Cry1Ab toxin determination in GM maize samples determined in different laboratories using a standardised JP.
Cry1Ab concentrations determined by in-house (own) protocols
In addition to the JP, samples were analysed in Lab 1, Lab 3 and Lab 4 by their in-house methods (own protocols) that may have been very similar or quite diverse from the JP in given laboratories. For example, the only difference in the own method of Lab 1 from the JP was the use of BBST instead of PBST. In contrast, Lab 4 used an ELISA from a different manufacturer (Abraxis) with Cry1Ab protoxin standards in its own in-house method.
Cry1Ab toxin content determined in the maize samples in the laboratories by their own protocols with their SDs and deviations from the corresponding values determined in the same laboratory by the JP are listed in with corresponding statistical parameters shown on . As seen from the data, Lab 1 determined statistically similar Cry1Ab concentrations for samples A and D, indicating that the change of the assay buffer from PBST to BBST does not affect assay performance. In contrast, Lab 3 obtained significantly higher (one-third to two-fold higher) concentration values with its own method than with the JP. Moreover, the statistical parameters (e.g.%SD) of the results obtained with their own method are mostly higher than those by the JP, indicating that results by the JP appear to be more reliable than those by their own protocol. This is explained by the fact that the JP has been formulated as an optimised version of their in-house method. Lab 4 obtained substantially lower Cry1Ab concentration values by their own protocol than by the JP. This may be explained by two main factors: differences in the extraction procedure and the ELISA kit applied. Lab 4 applied traditional mortar and pestle method for homogenisation during sample extraction, and lower efficacy of this method may account for a part of the difference in the performance of the two assay protocols. Nonetheless, experimental data (data not shown) indicated that this factor cannot explain the 60.8% difference in the detected concentration values. An additional and probably more important factor is the difference of the ELISA methods applied in the two protocols. The Bt-Cry1Ab-1Ac ELISA kit by Agdia, based on Cry1Ab protoxin-specific antibodies, was used with activated Cry1Ab protoxin as analytical standard. In contrast, Bt-Cry1Ab/Ac ELISA kit by Abraxis, also based on Cry1Ab protoxin-specific antibodies, contains manufacturer-supplied Cry1Ab protoxin standards, and uses linear calibration in the concentration range of 0.25–4 ng/ml (with blank control). Therefore, detected concentration values refer to protoxin equivalents, and are subject to correction by Cry1Ab protoxin/toxin cross-reactivity. Moreover, due to detection in a lower concentration range, the Abraxis ELISA protocol required higher sample dilution rates, and results were based on the less accurate calibration on the lower (linear) plateau of the sigmoid standard curve than near the inflexion point at the C50 level.
Table 6. Relative D and SE in Cry1Ab toxin determination in GM maize samples determined in different laboratories using a standardised JP.
Conclusion
Our data emphasize both the importance and the feasibility of a standardised protocol among laboratories for comparable Cry1Ab toxin quantification. However, even when using a standardised protocol, significant differences still occur among toxin concentrations detected in different laboratories although with a substantially smaller range of variation. Although a significant (−30 to 60%) SD still occurred among Cry1Ab toxin values detected in the corresponding laboratories by the JP, this deviation remains far below corresponding variability seen in other systematic studies (Nguyen et al., Citation2008) or observed plant-to-plant variation in Cry1Ab toxin levels (Then & Lorch, Citation2008). This improvement is certainly due to the use of well-controlled reference materials (standards, ELISA kits and protocol). However, in future efforts this can likely be further reduced when standardising the remaining differences in protocols between the laboratories. We have therefore demonstrated that it is feasible to standardise ELISA detection methods for quantification of Bt toxins with due diligence and carefully detailed protocols. However, room for improvement remains as we detail below.
Table 7. Relative SD and SE in Cry1Ab toxin determination in GM maize samples determined in different laboratories using their modified (own) protocols.
Results of our inter-laboratory study demonstrated that no matrix effects occurred between toxin concentrations detected in assay buffer or blank leaf extract calibrators. Within laboratory variability may be due to human factors (practice, pipetting, dilution errors) or impreciseness of the methods and instruments used (differences in sample preparation/extraction). It is obvious that using automated extraction and pipetting lowers the within laboratory variability of the data compared to manual techniques. However, such expensive equipment may not be readily available in all laboratories, for example, in developing countries or countries in transition struggling for proper funding or research and equipment.
The significant differences in results obtained by different ELISA methods on the same samples calls the attention to accurate comparisons of commercially available ELISA methods and proper interpretation of the results they provide (e.g. activated toxin/protoxin cross-reactivity). The remaining variability of the analytical results can be reduced by further standardisation of toxin determination including sample handling and preparation, laboratory instruments and equipment, process automation, water quality and so on. Using certified reference samples with known Bt concentrations also would contribute to the evaluation of the analytical reliability of given concentration values determined. Larger round robin analyses are the next logic step towards developing a certified standardised protocol.
Finally, our results provide compelling evidence that, currently, reproducibility of reported Cry toxin measurements is low. Hence, single results of Cry1Ab concentrations cannot be taken as a definitive or absolute concentrations without further validation. An outstanding example is the reported concentrations of Cry toxins in pollen of MON 810 maize stemming from one study only (Hellmich et al., Citation2001) and widely cited as the ‘gold standard’ until determined in other studies later (Nguyen & Jehle, Citation2007; Székács et al., Citation2010a). Further, any mathematical calculations of decline or increases in Cry toxin concentrations through food chains or soil compartments or over time (Romeis, Meissle, Raybould, & Hellmich, Citation2009) are associated with such huge uncertainties that they are at this point of time of little (to no) relevance for ERA and IRM strategies until further confirmation through repeated and rigorous validation using standardised ELISA protocols.
Acknowledgements
This work was supported by a consortium of different foundations Stiftung Gekko, Zukunftsstiftung Landwirtschaft, Testbiotech e.V. and Gesellschaft für ökologische Forschung e.V. This work was carried out as a joint project of members of the European Network of Scientists for Social and Environmental Responsibility (ENSSER).
References
- Adamczyk , J.J. Jr , Adam , L.C. and Hardee , D.D. 2001 . Field efficacy and seasonal expression profiles for terminal leaves of single and double Bacillus thuringiensis toxin cotton genotypes . Journal of Economic Entomology , 94 : 1589 – 1593 .
- Andrews , R.E. , Bibilos , M.M. and Bulla , L.A. 1985 . Protease activation of the entomocidal protoxin of Bacillus thuringiensis subsp kurstaki . Applied and Environmental Microbioliology , 50 : 737 – 742 .
- Barton , K.A. , & Miller , M.J. 1998 . Expression of genes in plants . U.S. Patent 2001/0003849, 17 April 1998 .
- Baumgarte , S. and Tebbe , C.C. 2005 . Field studies on the environmental fate of the Cry1Ab Bt-toxin produced by transgenic maize (MON810) and its effect on bacterial communities in the maize rhizosphere . Molecular Ecology , 14 : 2539 – 2551 .
- Bietlot , H. , Carey , P.R. , Choma , C. , Kaplan , H. , Lessard , T. and Pozsgay , M. 1989 . Facile preparation and characterization of the toxin from Bacillus thuringiensis var. kurstaki . Biochemical Jornal , 260 : 87 – 91 .
- Bravo , A. , Gill , S.S. and Soberón , M. 2007 . Mode of action of Bacillus thuringiensis Cry and Cyt toxins and their potential for insect control . Toxicon , 49 : 423 – 435 .
- Bravo , A. , Sanchez , J. , Kouskoura , T. and Crickmore , N. 2002 . N-terminal activation is an essential early step in the mechanism of action of the Bacillus thuringiensis Cry1Ac insecticidal toxin . Journal of Biological Chemistry , 277 : 23985 – 23987 .
- Bruns , H.A. and Abel , C.A. 2003 . Nitrogen fertility effects on Bt delta-endotoxin and nitrogen concentrations of maize during-early growth . Agronomy Journal , 95 : 207 – 211 .
- Chen , M. , Ye , G. , Liu , Z. , Fang , Q. , Hu , C. Peng , Y. 2009 . Analysis of Cry1Ab toxin bioaccumulation in a food chain of Bt rice, an herbivore and a predator . Ecotoxicology , 18 : 230 – 238 .
- Chilcutt , C.F. and Tabashnik , B.E. 2004 . Contamination of refuges by Bacillus thuringiensis toxin genes from transgenic maize . Proceedings of the National Academy of Sciences of the United States of America , 101 : 7526 – 7529 .
- Choma , C.J. and Kaplan , H. 1990 . Folding and unfolding of protoxin from Bacillus thuringiensis: Evidence that the toxic moiety is present in an active confirmation . Biochemistry , 29 : 10971 – 10977 .
- Choma , C.J. and Kaplan , H. 1991 . The toxic moiety of Bacillus thuringiensis protein undergoes a conformational change upon activation . Biochemical and Biophysical Research Communications , 179 : 1934 – 1938 .
- Crespo , A.L.B. , Spencer , T.A. , Nekl , E. , Pusztai-Carey , M. , Moar , W.J. and Siegfried , B.D. 2008 . Comparison and validation of methods to quantify Cry1Ab toxin from Bacillus thuringiensis for standardization of insect bioassays . Applied and Environmental Microbioliology , 74 : 130 – 135 .
- De Maagd , R.A. , Bosch , D. and Stiekema , W. 1999 . Bacillus thuringiensis toxin mediated insect resistance in plants . Trends in Plant Science , 4 : 9 – 13 .
- Dhillon , M.K. and Sharma , H.C. 2009 . Effects of Bacillus thuringiensis delta-endotoxins Cry1Ab and Cry1Ac on the coccinellid beetle, Cheilomenes sexmaculatus (Coleoptera, Coccinellidae) under direct and indirect exposure conditions . Biocontrol Science and Technology , 19 : 407 – 420 .
- Díaz-Mendoza , M. , Farinós , G.P. , Castañera , P. , Hernández-Crespoand , P. and Ortego , F. 2007 . Proteolytic processing of native Cry1Ab toxin by midgut extracts and purified trypsins from the Mediterranean corn borer Sesamia nonagrioides . Journal of Insect Physiology , 53 : 428 – 435 .
- Dively , G.P. , Rose , R. , Sears , M.K. , Hellmich , R.L. , Stanley-Horn , D.E. Calvin , D.D. 2004 . Effects on Monarch butterfly larvae (Lepidoptera: Danaidae) after continuous exposure to Cry1Ab-expressing corn during anthesis . Environmental Entomology , 33 : 1116 – 1125 .
- Douville , M. , Gagne , F. , Masson , L. , McKay , J. and Blaise , C. 2005 . Tracking the source of Bacillus thuringiensis Cry1Ab endotoxin in the environment . Biochemical Systematics and Ecology , 33 : 219 – 232 .
- Ermolli , M. , Fantozzi , A. , Marini , M. , Scotti , D. , Balla , B. Hoffmann , S. 2006a . Food safety: screening tests used to detect and quantify GMO proteins . Accreditation and Quality Assurance , 11 : 55 – 57 .
- Ermolli , M. , Prospero , A. , Balla , B. , Querci , M. , Mazzeo , A. and Van Den Eede , G. 2006b . Development of an innovative immunoassay for CP4EPSPS and Cry1AB genetically modified protein detection and quantification . Food Additives and Contaminants , 23 : 876 – 882 .
- Ezequiel , M. , Reggiardo , M.I. , Vallejos , R.H. and Permingeat , H.R. 2006 . Detection of BT transgenic maize in foodstuffs . Food Research International , 39 : 250 – 255 .
- Fantozzi , A. , Ermolli , M. , Marini , M. , Scotti , D. , Balla , B. Querci , M. 2007 . First application of a microsphere-based immunoassay to the detection of genetically modified organisms (GMOs): Quantification of Cry1Ab protein in genetically modified maize . Journal of Agricultural and Food Chemistry , 55 : 1071 – 1076 .
- Gao , Y. , Fancil , K.J. , Xu , X. , Schwedler , D.A. , Gilbert , J.R. and Herman , R.A. 2006 . Purification and characterization of a chimeric Cry1F delta-endotoxin expressed in transgenic cotton plants . Journal of Agricultural and Food Chemistry , 54 : 829 – 835 .
- Gao , Y. , Schafer , B.W. , Collins , R.A. , Herman , R.A. , Xu , X. Gilbert , J.R. 2004 . Characterization of Cry 34Ab1 and Cry35Ab1 insectcidal crystal proteins expressed in transgenic corn plants and Pseudomonas fluorescens . Journal of Agricultural and Food Chemistry , 52 : 8057 – 8065 .
- Gill , S.S. , Cowles , E.A. and Pietrantonio , P.V. 1992 . The mode of action of Bacillus thuringiensis endotoxins . Annual Review of Entomology , 37 : 615 – 636 .
- Gould , F. 1998 . Sustainability of transgenic insecticidal cultivars: Integrating pest genetics and ecology . Annual Review of Entomology , 43 : 701 – 726 .
- Gould , F. 2000 . Testing Bt refuge strategies in the field . Nature Biotechnology , 18 : 266 – 267 .
- Grothaus , G.D. , Bandla , M. , Currier , T. , Giroux , R. , Jenkins , G.R. Lipp , M. 2006 . Immunoassay as an analytical tool in agricultural biotechnology . Journal of AOAC International , 89 : 913 – 928 .
- Harwood , J.D. , Wallin , W.G. and Obrycki , J.J. 2005 . Uptake of Bt endotoxins by nontarget herbivores and higher order arthropod predators: molecular evidence from a transgenic corn agroecosystem . Molecular Ecology , 14 ( 9 ) : 2815 – 2823 .
- Hellmich , R.L. , Siegfried , B.D. , Sears , M.K. , Stanley-Horn , D.E. , Mattila , H.R. Spencer , T. 2001 . Monarch larvae sensitivity to Bacillus thuringiensis purified proteins and pollen . Proceedings of the National Academy of Sciences of the United States of America , 98 : 11925 – 11930 .
- Hilbeck , A. , Baumgartner , M. , Fried , P.M. and Bigler , F. 1998a . Effects of transgenic Bt corn-fed prey on immature development of Chrysoperla carnea (Neuroptera: Chrysopidae) . Environmental Entomology , 27 : 480 – 487 .
- Hilbeck , A. , Moar , W. , Pusztai-Carey , M. , Filipini , A. and Bigler , F. 1998b . Toxicity of Bacillus thuringiensis Cry1Ab toxin to the predator Chrysoperla carnea (Neuroptera: Chrysopidae) . Environmental Entomology , 27 : 1255 – 1263 .
- Hilbeck , A. , Moar , W. , Pusztai-Carey , M. , Filipini , A. and Bigler , F. 1999 . Prey-mediated effects of Cry1Ab toxin and protoxin and Cry2A protoxin on the predator Chrysoperla carnea (Neuroptera: Chrysopidae) . Entomologia Experimentalis et Applicata , 91 : 305 – 316 .
- Hilbeck , A. and Schmidt , J.E.U. 2006 . Another view on Bt proteins – How specific are they and what else might they do? . Biopesticides International , 2 : 1 – 50 .
- Höfte , H. and Whiteley , H. 1989 . Insecticidal crystal proteins of Bacillus thuringiensis . Microbiological Reviews , 53 : 242 – 255 .
- Huber-Lukac , M. , Luthy , P. and Braun , D.G. 1983 . Specificities of monoclonal antibodies against the activated delta-endotoxin of Bacillus thuringiensis var. thuringiensis . Infection and Immunity , 40 : 608 – 612 .
- Icoz , I. , Andow , D. , Zwahlen , C. and Stotzky , G. 2009 . Is the Cry1Ab protein from Bacillus thuringiensis (Bt) taken up by plants from soils previously planted with Bt corn and by carrot from hydroponic culture? . Bulletin of Environmental Contamination and Toxicology , 83 : 48 – 58 .
- International Organization for Standardization . 1994 . ISO 5725-4:1994 Accuracy (trueness and precision) of measurement methods and results . Part 4: Basic methods for the determination of the trueness of a standard measurement method (http://www.iso.org/iso/home.htm) .
- Kruger , M. , van Rensburg , J.B.J. and van den Berg , J. 2009 . Perspective on the development of stem borer resistance to Bt maize and refuge compliance at the Vaalharts irrigation scheme in South Africa . Crop Protection , 28 : 684 – 689 .
- Lambert , B. , Buysse , L. , Decock , C. , Jansens , S. , Piens , C. Saey , B. 1996 . A Bacillus thuringiensis insecticidal crystal protein with a high activity against members of the family Noctuidae . Applied and Environmental Microbioliology , 62 : 80 – 86 .
- Lang , A. , Brunzel , S. , Dolek , M. , Otto , M. and Theißen , B. 2011 . Modelling in the light of uncertainty of key parameters: a call to exercise caution in field predictions of Bt-maize effects . Proceedings of the Royal Society B , 278 : 980 – 981 .
- Lipp , M. , Anklam , E. and Stave , J.W. 2000 . Validation of an immunoassay for detection and quantitation of genetically modified soybean in food and food fractions using reference materials: Interlaboratory study . Journal of AOAC International , 83 : 919 – 927 .
- Liu , C.-M. and Tzeng , Y.M. 2001 . Quantitative analysis of parasporal crystal protein from Bacillus thuringiensis by capillary electrophoresis . Journal of Food and Drug Analysis , 9 : 79 – 83 .
- Lung , J.H.T. , Male , K.B. , Mazza , A. , Masson , L. and Broussau , R. 2004 . Analysis of insecticidal proteins from Bacillus thuringiensis and recombinant Escherichia coli by capillary electrokinetic chromatography . Electrophoresis , 25 : 3292 – 3299 .
- Margarit , E. , Reggiardo , M.I. , Vallejos , R.H. and Permingeat , H.R. 2006 . Detection of BT transgenic maize in foodstuffs . Food Research International , 39 : 250 – 255 .
- Masson , L. , Erlandson , M. , Pusztai-Carey , M. , Brousseau , R. , Juarez-Perez , V. and Frutos , R. 1998 . A holistic approach for determining the entomopathogenic potential of Bacillus thuringiensis strains . Applied and Environmental Microbioliology , 64 : 4782 – 4788 .
- McGaughey , W.H. , Gould , F. and Gelernter , W. 1998 . Bt resistance management . Nature Biotechnology , 16 : 144 – 146 .
- Mendelsohn , M. , Kough , J. , Zigfridais , V. and Matthewsm , K. 2003 . Are Bt-crops safe? . Nature Biotechnology , 21 : 1003 – 1009 .
- Milne , R. and Kaplan , H. 1993 . Purification and characterization of a trypsin-like digestive enzyme from spruce budworm (Choristoneura fumiferana) responsible for the activation of Δ-endotoxin from Bacillus thuringiensis . Insect Biochemistry and Molecular Biology , 23 : 663 – 673 .
- Miranda , R. , Zamudio , F.Z. and Bravo , A. 2001 . Processing of Cry1Ab delta endotoxin from Bacillus thuringiensis by Manduca sexta and Spodoptera frugiperda midgut proteases: Role in protoxin activation and toxin inactivation . Insect Biochemistry and Molecular Biology , 31 : 1155 – 1163 .
- Mohan , M. and Gujar , G.T. 2003 . Characterization and comparison of midgut proteases of Bacillus thuringiensis susceptible and resistant diamondback moth (Plutellidae: Lepidoptera) . Journal of Invertebrate Pathology , 82 : 1 – 11 .
- Monsanto . 2002 . Safety assessment of YieldGard insect-protected event MON810 . Published by agbios.com as Product Safety Description. Retrieved March 17, 2009, from http://agbios.com/docroot/decdocs/02-269-010.pdf
- Nguyen , H.T. , Hunfeld , H. , Meissle , M. , Miethling-Graff , R. , Pagel-Wieder , S. Rauschen , S. 2008 . Round robin quantitation of Cry3Bb1 using the qualitative PathoScreen ELISA . IOBC/WPRS Bulletin , 33 : 59 – 66 .
- Nguyen , T.H. and Jehle , J.A. 2007 . Quantitative analysis of the seasonal and tissue-specific expression of Cry1Ab in transgenic maize MON 810 . Journal of Plant Diseases and Protection , 114 : 82 – 87 .
- Nguyen , J. and Russel , S.C. 2010 . Targeted proteomics approach to species-level identification of Bacillus thuringiensis spores by AP-MALDI-MS . Journal of the American Society for Mass Spectrometry , 21 : 993 – 1001 .
- Oppert , B. 1999 . Protease interactions with Bacillus thuringiensis insecticidal toxins . Archives of Insect Biochemistry and Physiology , 42 : 1 – 12 .
- Park , H.W. , Bideshi , D.K. and Federici , B.A. 2000 . Molecular genetic manipulation of truncated Cry1C protein synthesis in Bacillus thuringiensis to improve stability and yield . Applied and Environmental Microbioliology , 66 : 4449 – 4455 .
- Perry , J.N. , Devos , Y. , Arpaia , S. , Bartsch , D. , Gathmann , A. Hails , R.S. 2010 . A mathematical model of exposure of nontarget Lepidoptera to Bt-maize pollen expressing Cry1Ab within Europe . Proceedings of the Royal Society B , 277 : 1417 – 1425 .
- Pigott , C.R. and Ellar , D.J. 2007 . Role of receptors in Bacillus thuringiensis crystal toxin activity . Microbiology and Molecular Biology Reviews , 71 : 255 – 281 .
- Ranasinghe , C. and Akhurst , J.R. 2002 . Matrix assisted laser desorption ionisation time of flight mass spectrometry (MALDI-TOF MS) for detecting novel Bt toxins . Journal of Invertebrate Pathology , 79 : 51 – 58 .
- Romeis , J. , Meissle , M. , Raybould , A. and Hellmich , R.L. 2009 . “ Impact of insect-resistant genetically modified crops on non-target arthropods ” . In Environmental impact of genetically modified crops , Edited by: Ferry , N. and Gatehouse , A.M.R. 165 – 198 . Wallingford : CABI .
- Schmidt , J.E.U. , Braun , C.U. , Whitehouse , L.P. and Hilbeck , A. 2008 . Effects of activated Bt transgene products (Cry1Ab, Cry3Bb) on immature stages of the ladybird Adalia bipunctata in laboratory ecotoxicity testing . Archives of Environmental Contamination and Toxicology , 56 : 221 – 228 .
- Schnepf , E. , Crickmore , N. , Van Rie , J. , Lereclus , D. , Baum , J. Feitelson , J. 1998 . Bacillus thuringiensis and its pesticidal crystal proteins . Microbiology and Molecular Biology Reviews , 62 : 775 – 806 .
- Shao , Z. , Cui , Y. , Liu , X. , Yi , H. , Ji , J. and Yu , Z. 1998 . Processing of delta-endotoxin of Bacillus thuringiensis subsp. kurstaki HD-1 in Heliothis armigera midgut juice and the effects of protease inhibitors . Journal of Invertebrate Pathology , 72 : 73 – 81 .
- Shapiro , S.S. and Wilk , M.B. 1965 . An analysis of variance test for normality . Biometrika , 52 : 591 – 599 .
- Sims , S.R. and Berberich , S.A. 1996 . Bacillus thuringiensis CryIA protein levels in raw and processed seed of transgenic cotton: Determination using insect bioassay and ELISA . Journal of Economic Entomology , 89 : 247 – 251 .
- Soberón , A. , Gill , S.S. and Bravo , A. 2009 . Signaling versus punching hole: How do Bacillus thuringiensis toxins kill insect midgut cells? . Cellular and Molecular Life Sciences , 66 : 1337 – 1349 .
- Stave , J.W. 1999 . Detection of new or modified proteins in novel foods derived from GMO-future needs . Food Control , 10 : 367 – 374 .
- Stave , J.W. 2002 . Protein immunoassay methods or detection of biotech crops: Applications, limitations, and practical considerations . Journal of AOAC International , 85 : 780 – 786 .
- Székács , A. , Lauber , É. , Juracsek , J. and Darvas , B. 2010a . Cry1Ab toxin production of MON 810 transgenic maize . Environmental Toxicology and Chemistry , 29 : 182 – 190 .
- Székács , A. , Lauber , É. , Takács , E. and Darvas , B. 2010b . Detection of Cry1Ab toxin in the leaves of MON 810 transgenic maize . Analytical and Bioanalytical Chemistry , 396 : 2203 – 2211 .
- Takahashi , Y. , Hori , H. , Furuno , H. , Kawano , T. , Takahashi , M. and Wada , Y. 1998 . Enzyme-linked immunosorbent assays for rapid and quantitative detection of insecticidal crystal proteins of Bt pesticides . Journal of Pesticide Science , 23 : 386 – 391 .
- Tapp , G. and Stotzky , G. 1995 . Dot blot enzyme-linked immunosorbent assay for monitoring the fate of insecticidal toxins from Bacillus thuringiensis in soil . Applied and Environmental Microbioliology , 61 : 602 – 609 .
- Then , C. 2010 . Risk assessment of toxins derived from Bacillus thuringiensis-synergism, efficacy, and selectivity . Environmental Science and Pollution Research , 17 : 791 – 797 .
- Then , C. and Lorch , A. 2008 . “ A simple question in a complex environment: How much Bt toxin do genetically engineered MON810 maize plants actually produce? ” . In Implications of GM-crop cultivation at large spatial scales. Theorie in der Ökologie: Vol. 14 , Edited by: Breckling , B. , Reuter , H. and Verhoeven , R. 17 – 21 . Frankfurt : Peter Lang .
- Volpe , G. , Ammid , N.H. , Moscone , D. , Occhigrossi , L. and Palleschi , G. 2006 . Development of an immunomagnetic electrochemical sensor for detection of BT-CRY1AB/CRY1AC proteins in genetically modified corn samples . Analytical Letters , 39 : 1599 – 1609 .
- Walschus , U. , Witt , S. and Wittmann , C. 2002 . Development of monoclonal antibodies against Cry1Ab protein from Bacillus thuringiensis and their application in an ELISA for detection of transgenic Bt-maize . Food and Agricultural Immunology , 14 : 231 – 240 .
- Wang , S. , Guo , A.Y. , Zheng , W.J. , Zhang , Y. , Qiao , H. and Kennedy , I.R. 2007 . Development of ELISA for the determination of transgenic Bt-cottons using antibodies against Cry1Ac protein from Bacillus thuringiensis HD-73 . Engineering in Life Sciences , 7 : 149 – 154 .
- Wu , Z.P. , Xu , B.J. and Chen , Z.Y. 2001 . Separation and purification of Bacillus thuringiensis (Bt) toxic protein Cry1Ab from transgenic rice by ion-exchange chromatography . Chromatogarphia , 53 : 571 – 573 .
- Xie , X. and Shu , Q. 2001 . Studies on rapid quantitative analysis of Bt toxin by using Envirologix kits in transgenic rice . Scientia Agricultura Sinica , 34 : 465 – 468 .
- Yamamoto , T. 1983 . Identification of entomocidal toxins of Bacillus thuringiensis by high performance liquid chromatography . Journal of General Microbiology , 129 : 2595 – 2603 .
- Zwahlen , C. , Hilbeck , A. , Gugerli , P. and Nentwig , W. 2003 . Degradation of the Cry1Ab protein within transgenic Bacillus thuringiensis corn tissue in the fields . Molecular Ecology , 12 : 765 – 775 .