Abstract
Bovine β-lactoglobulin (βLG) is a major allergen in cow's milk. It is crucial to develop new methods that would reduce the allergenicity and not destroy the functional properties of βLG. To reduce allergenicity of βLG, tea catechins were bound to βLG to form βLG–catechin complex. The structure of βLG–catechin complex was analysed by circular dichroism (CD) spectra and fluorescence spectra assays. The results suggested that βLG was bound by catechin without apparent disruption of native conformation. Enzyme-linked immunosorbent assay (ELISA) indicated that the IgE-binding and IgG-binding activities of βLG were decreased upon catechin binding. Our study may be helpful for development of a new method to prepare milk proteins with reduced allergenicity.
Introduction
Bovine β-lactoglobulin (βLG) is a major whey protein of MW 18,400 with two disulfide bridges and one free cysteine residue. This protein is one of the best characterised lipid-binding proteins and as such it is capable of binding a wide range of molecules including retinol, β-carotene and fatty acid (Flower, Citation1996). βLG is ubiquitously used as a food additive for its multiple functions in food processing (Foegeding, Kuhn, & Hardin, Citation1992; Shimizu, Saito, & Yamauchi, Citation1985). However, βLG is a major allergen in cow's milk, and about 82% of milk allergy patients are sensitive to this protein (Spies, Citation1973). Many attempts to reduce the allergenicity of proteins have been made by enzymatic digestion and denaturation (del Val et al., Citation1999; Ishizaka, Okudaira, & King, Citation1975; Kurisaki, Nakamura, Kaminogawa, & Yamauchi, Citation1982; Watanabe, Suzuki, Ikezawa, & Arai, Citation1994). However, these methods might destroy useful functions of the proteins and have adverse effects of their taste (Monaci, Tregodt, Hengel, & Anklam, Citation2006). Therefore, it is crucial to develop new methods that would reduce the allergenicity and not destroy the functional properties of βLG.
Polyphenols of green tea are widely investigated for their multifarious properties and potential health benefits attributed to their strong antioxidative activity (Jaziri, Slama, Mhadhbi, Urdaci, & Hamdi, Citation2009). A considerable amount of research has reported that phenolic compounds have an ability to interact with milk proteins (Kanakis et al., Citation2011; Fox, Citation2001; Svetli, Guy, & Heidar-Ali, Citation2010; Zerrin, Elif, & Yasar, Citation2010). Tea polyphenols contribute to the functionality of the milk products (Batra & Saxena, Citation2005; O'Connell & Fox, Citation1999). Binding interactions of green tea polyphenols with milk proteins can be used for manufacturing of novel milk products with desired textural properties as well as antioxidant capacity (Zerrin et al., Citation2010). It is thought that the interacting ability of green tea polyphenols with milk protein was vital in modification of textural properties of milk products. Therefore, we expect that through binding interaction, tea polyphenols not only alter functions of βLG such as emulsifying, foaming and gelling properties but also can change its immunoreactivity.
Herein we prepared a complex of βLG by binding βLG with green tea catechins. After exploring the structures of this complex, we analysed IgE-binding and IgG-binding capacity of βLG before and after treatment of catechin by enzyme-linked immunosorbent assay (ELISA).
Materials and methods
Preparation of βLG–catechin complex
β-lactoglobulin was from Sigma Chemical Co., (St. Louis, MO,USA). Green tea catechins were purchased from Chengdu Biopurify Photochemicals Ltd. (Chengdu, China). The βLG–catechin complex was prepared according to the method of Prigent et al. (Citation2003). In brief, 0.1 g of βLG was dissolved in 100 mL of 0.1 M phosphate-buffered saline (PBS; pH 7.0). Fifty mole catechin per mole protein was added to the βLG solution and incubated for 1 h at room temperature with stirring. Blanks without catechin were also incubated at the same time. After incubation, all samples were dialysed overnight in Slide-A-Lyzer minidialysis units (Pierce Chemical Co., Rockford, IL, USA.) with a molecular weight cut-off of 10 kDa against distilled water at 4°C to remove the free catechin.
Production of anti-βLG polyclonal antibodies
Anti-βLG polyclonal antibodies were prepared in mice by using native βLG as a soluble antigen. The antigen protein in Freund's complete adjuvant was intraperitoneally injected into BALB/c mice. Booster injections of the same amount of the antigen protein in Freund's incomplete adjuvant were administered twice at two-week intervals. The antiserum was prepared after centrifugation to remove blood clots and analysed by western blotting to test its IgG binding ability toward βLG. The antiserum was stored at −20°C until use.
Human sera
Pooled human sera were collected from 15 children. Individual serum samples from children with proven allergy to milk proteins were collected the Shenzhen Children's Hospital. All patients showed specific IgE antibodies towards milk proteins, which were determined by the Uni-CAP System (Pharmacia Diagnostics, Uppsala, Sweden). All sera were analysed by western blotting and demonstrated IgE-binding ability toward βLG. Pooled normal sera from patients without a cow's milk hypersensitivity reaction were used as negative controls. All serum samples were given informed consent and authenticated by the ethical board of Shenzhen University and stored at −20°C until use.
Conformational analysis of the βLG–catechin complex by spectroscopic methods
The circular dichroism (CD) spectra for native βLG and βLG–catechin complex were measured. The samples were recorded at 25°C with a Jasco-810 spectrophotometer (JASCO Co., Tokyo, Japan) in cells of 1.0 mm path length. Each sample was dissolved in PBS at a protein concentration of 1.5×10−6 M. The spectra were recorded in the range of 190–250 nm with a scan rate of 30 nm/min and a response time of 1 s. Three scans were accumulated for each spectrum.
The intrinsic fluorescence of native βLG and βLG–catechin complex dissolved in PBS at a 1.0×10−6 M (as the protein concentration) were recorded on a Hitachi-850 spectrofluorometer (Hitachi Co., Tokyo, Japan) in a 1-cm quartz cell using an excitation wavelength of 280 nm. The excitation and emission bandwidths were 5 nm. The emission spectra were recorded from 310 to 450 nm.
The retinol-binding activity of βLG and βLG–catechin complex was investigated by fluorescence titration with the Hitachi-850 spectrofluorometer (Hitachi Co., Tokyo, Japan) according to Hattori, Ogino, Nakai, and Takahashi (Citation1997).
ELISA experiments
The IgG binding of native βLG and βLG–catechin complex was evaluated by competitive ELISA. Aliquots of βLG solution (100µL; 0.001% βLG in PBS) were added to the wells of a polystyrene microtitration plate and plates were incubated at 4°C overnight. The βLG solution was then removed. The wells were washed with PBST (0.05% Tween 20 in PBS). This washing system was used after each incubation step. And 3.0% BSA in PBST (0.02% Tween 20 in PBS) for 2 h at 37°C was added. The plate was again incubated at 37°C for 2 h and washed. βLG or βLG-catechin at various concentrations (50 µL) was added to the wells, then anti-βLG polyclonal antibodies (50 µL) were immediately added, and the plate was further incubated at 37°C for 1 h. After removing the solution and washing the wells, plates were then incubated with 100 µL per well of horseradish peroxidase (HRP) labelled Goat Anti-Mouse IgG (Kirkegaard & Perry Laboratories, Inc., Gaithersburg, MD, USA) diluted 1:10,000 in PBS solution. The plate was incubated for 45 min at 37°C. Fifty µL of substrate containing 0.4 mg/mL o-phenylenediamine and five µL H2O2 were added for colour development. Fifty µL of 2 M H2SO4 was added to stop the enzyme reaction. Optical densities (OD) were read at 450 nm on an automated ELISA plate reader (Multiskan MK3, Thermo Labsystems,Vantaa, Finland).
The IgE-binding assay was performed similarly to that of IgG but with slight modifications. Briefly, wells were coated with 100 µL of antigen as described earlier. After the blocking step, βLG or βLG-catechin at various concentrations (50 µL) was added to the wells, plates were then incubated with 50 µL per well of human sera (serum at 1:50 diluted in PBST solution) for 1 h at 37°C. 100 µL per well of HRP-conjugated antihuman IgE (Kirkegaard & Perry Laboratories, Inc., Gaithersburg, MD, USA) were added at a 1:1000 dilution in PBST solution. Finally, colour development was performed as described earlier.
The ELISA determinations were carried out in triplicate and measurements averaged.
Results and discussion
Structural features of the βLG–catechin complex
The fluorescence emission spectra of the βLG–catechin complex are shown in A. The fluorescence emission maximum wavelength was 337 nm for native βLG and 336 nm for the complex. It has been previously shown that the fluorescence intensity decreases with the blue-shift of the wavelength for maximum emission as the conformation of βLG changes. As only a slight blue-shift was observed in the complex, the conformation around the tryptophan (Trp) residues of the βLG–catechin complex was considered to be maintained in the native form. The fluorescence intensity of βLG–catechin complex was lower than that of native βLG, which suggested that the catechin shielded the area around the Trp residues (Hattori, Nagasawa, Ametani, Kaminogawa, & Takahashi, Citation1994; Hattori et al., Citation1997, 2000a; Lakowicz & Weber, Citation1973).
The CD spectra of the βLG–catechin complex are shown in B. The CD spectra of samples exhibited two negative bands in the ultraviolet region at 208 nm and 218 nm, this is characteristic of α-helical structures of a protein (Venyaminov & Vesilenko, Citation1994). The spectrum for the complex was similar to the spectrum for native protein. The secondary structure was determined using CONTIN method in DICHROWEB (http://dichroweb.cryst.bbk.ac.uk/html/home.shtml). By this method, it was found that the α-helical content of the protein changed from 21% to 24% upon binding with catechin. This suggested that the binging interaction of catechin with βLG only caused slight conformation change of protein.
β-lactoglobulin belongs to the lipocalin superfamily and can bind two molecules of retinol (Fugate & Song, Citation1980; Pèrez & Calvo, Citation1995). Change in the retinol-binding activity of βLG after binding by catechin was investigated by fluorescence titration (). The retinol-binding activity of βLG was reduced a little by conjugation with catechin. The structure involved in retinol-binding in the βLG–catechin complex was considered to have been maintained in the native form.
Therefore, βLG binding by catechin maintained the native-like protein structure, although slight changes in the structure were observed.
ELISA experiments
The IgE-binding and IgG-binding capacities of βLG and βLG-catechin were measured by ELISA. shows that the reactivity of native βLG with IgG antibodies and IgE antibodies was apparently decreased after binding by catechin.
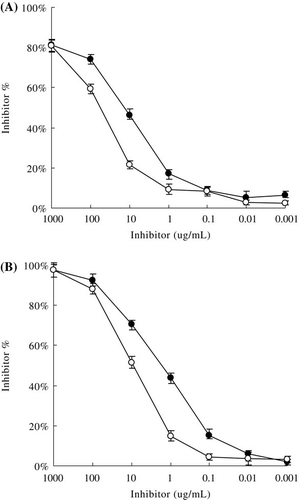
The molecular basis of changes in allergenic activity is the inactivation or destruction of epitope structures or increased inaccessibility to epitopes (Besler, Steinhart, & Paschke, Citation2001). According to structural analyses, binding catechin did not disrupt the native conformation of βLG greatly. βLG almost maintained the native-like protein structure. Therefore, the reduced allergenicity of βLG may not cause by significant structures changes. The reduction of the binding affinity of βLG–catechin complex may due to the shielding action of the βLG epitopes induced by the attachment of catechin to βLG.
As for the IgE response to βLG, 41Val-60Lys, 102Tyr-124Val, 149Arg-162Ile are reported to be major epitopes (Selo et al., 1999). And 51Glu-64Asp, 67Arg-88Asn, 129Asp-144Pro, 139Arg-156Thr are major epitopes for the IgG (Jarvinen et al., 2010). All these epitopes are on the protein surface. The results of structural analyses according to the spectroscopic experiment s indicated that the surface of βLG was covered by catechin. Catechin in βLG–catechin complex may be attached directly to the epitope(s) or region(s) in the proximity, which thereby prevent anti-βLG antibodies from accessing the antigens.
Kanakis et al. (Citation2011) observed that tea polyphenols could bind to βLG in solution. The catechins in green tea with antioxidant activity include epicatechin, epigallocatechin, epigallocatechingallate and epicatechingallate. Both hydrophobic and hydrophilic interactions were observed in the polyphenol–βLG complexation (Kanakis et al., Citation2011).
It is essential to develop a new method that can reduce the allergenicity and maintain the functional properties of βLG. Proteins can combine with non-allergenic materials, simultaneously decreasing the allergenicity and improving the function of the proteins (Hattori et al., 2000b). Although a little conformational change in βLG was observed after binding by catechin, the complex maintained the retinol-binding activity of protein. Our work is the first study to find that the catechin effectively shielded epitopes of βLG, which reduced IgE-binding and IgG-binding capacity of βLG in vitro. However, the mechanism behind the reduced immunogenicity of βLG through binding by catechin needs more study. We hope that this method will contribute to preparation of novel proteins with desired functional properties, but reduced allergenicity.
Acknowledgements
This study was supported in part by research funding from the High-tech Industrialization Funding of Guangdong Province (No.2009B011300010), and the National Natural Science Foundation of PRC (No.31101280).
References
- Batra, A., & Saxena, R.K. (2005). Potential tannase producers from the genera Aspergillus and Penicillium. Process Biochemistry, 40, 1553–1557.
- Besler, M., Steinhart, H., & Paschke, A. (2001). Stability of food allergens and allergenicity of processed foods. Journal of Allergy and Clinical Immunology B, 756, 228–238.
- del Val, G., Yee, B.C., Lozano, R.M., Buchanan, B.B., Ermel, R.W., Lee, Y.M., et al. (1999). Thioredoxin treatment increases digestibility and lowers allergenicity of milk. Journal of Allergy and Clinical Immunology, 103, 690–669.
- Flower, D.R. (1996). The lipocalin protein family: Structure and function. Biochemical Journal, 318, 1–14.
- Foegeding, E.A., Kuhn, P.R., & Hardin, C.C. (1992). Specific divalent cation-induced changes during gelation of β-lactoglobulin. Journal of Agriculture and Food Chemistry, 40, 2092–2097.
- Fox, P.F. (2001). Milk proteins as food ingredients. International Journal of Dairy Technology, 54, 41–55.
- Fugate, R., & Song, P. (1980). Spectroscopic characterization of β-lactoglobulin retinol complex. Biochimica and Biophysica Acta, 625, 28–42.
- Hattori, M., Ogino, A., Nakai, H., & Takahashi, K. (1997). Functional improvement of β-lactoglobulin by conjugating with alginate lyase-lysate. Journal of Agriculture and Food Chemistry, 45, 703–708.
- Hattori, M., Nagasawa, K., Ametani, A., Kaminogawa, S., & Takahashi, K. (1994). Functional changes in β-lactoglobulin by conjugation with carboxymethyl dextran. Journal of Agriculture and Food Chemistry, 42, 2120–2125.
- Hattori, M., Nagasawa, K., Ohgata, K., Sone, N., Fukuda, A., Matsuda, H., et al. (2000a). Reduced immunogenicity of β-lactoglobulin by conjugation with carboxymethyl dextran. Bioconjugate Chemisty, 11, 84–93.
- Hattori, M., Numamoto, K., Kobayashi, K., & Takahashi, K. (2000b). Functional changes in β-lactoglobulin by conjugation with cationic saccharides. Journal of Agriculture and Food Chemistry, 48, 2050–2056.
- Ishizaka, K., Okudaira, H., & King, T. (1975). Immunogenic properties of modified antigen E. II. Ability of urea-denatured antigen and a polypeptide chain to prime T cells specific for antigen. Journal Immunology, 114, 110–115.
- Jarvinen, K.M., Chatchatee, P., Bardina, L., Beyer, K., & Sampson, H.A. (2010). IgE and IgG binding epitopes on alpha-lactalbumin and beta-lactoglobulin in cow's milk allergy. International Archives of Allergy and Immunology, 126, 111–118.
- Jaziri, I., Slama, M.B., Mhadhbi, H., Urdaci, M.C., & Hamdi, M. (2009). Effect of green and black teas (Camellia sinensis L.) on the characteristic microflora of yogurt during fermentation and refrigerated storage. Food Chemistry, 112, 614–620.
- Kanakis, C.D., Hasni, I., Bourassa, P., Tarantilis, P.A., Polissiou, M.G., & Tajmir-Riahi, H.A. (2011). Milkβ-lactoglobulin complexes with tea polyphenols. Food Chemistry, 127, 1046–1055.
- Kurisaki, J., Nakamura, S., Kaminogawa, S., & Yamauchi, K. (1982). The antigenic properties of β-lactoglobulin examined with mouse IgE antibody. Agricultural Biology and Chemistry, 46, 2069–2075.
- Lakowicz, J.R., & Weber, G. (1973). Quenching of protein fluorescence by oxygen. detection of structural fluctuations in proteins on the nanosecond time scale. Biochemistry, 12, 4171–4179.
- Monaci, L., Tregodt, V., Hengel, A.J.V., & Anklam, E. (2006). Milk allergens, their characteristics and their detection in food: A review. European Food Research and Technology, 223, 149–179.
- O'Connell, J.E., & Fox, P.F. (1999). Effect of extracts of oak (Quercus petraea) bark, oak leaves, aloe vera (Curacao aloe), coconut shell and wine on the colloidal stability of milk and concentrated milk. Food Chemistry, 66, 93–96.
- Pèrez, M.D., & Calvo, M. (1995). Interaction of â-lactoglobulin with retinol and fatty acids and its role as a possible biological function for this protein. Journal of Dairy Science, 78, 978–988.
- Prigent, S.V.E., Gruppen, H., Visser, A.J.W.G., Van Koningsveld, G.A., De Jong, G.A.H., & Voragen, A.G.J. (2003). Effect of non-covalent interactions with 5-ocoffeoylquinic acid (chlorogenic acid) on the heat denaturation and solubility of globular proteins. Journal of Agriculture and Food Chemistry, 51, 5088–5095.
- Selo, I., Clement, G., Bernard, H., Chatel, J.M., Creminon, C., Peltre, G., & Wal, J.M. (1999). Allergy to bovine beta-lactoglobulin: specificity of Human IgE to tryptic peptides. Clinical and Experimental Allergy, 78, 1055–1063.
- Shimizu, M., Saito, M., & Yamauchi, K. (1985). Emulsifying and structural properties of β-lactoglobulin at different pHs. Agricultural Biology and Chemistry, 49, 189–194.
- Spies, J. (1973). Milk allergy. Milk Food Technology, 36, 225–231.
- Svetli, D., Guy, S., & Heidar-Ali, T. (2010). Dual effect of milk on the antioxidant capacity of green, Darjeeling, and English breakfast teas. Food Chemistry, 122, 539–545.
- Venyaminov, S.Y., & Vesilenko, K.S. (1994). Determination of protein tertiary structure class from circular dichroism spectra. Analytical Biochemistry, 222, 176–184.
- Watanabe, M., Suzuki, T., Ikezawa, Z., & Arai, S. (1994). Controlled enzymatic treatment of wheat proteins for production of hypoallergenic flour. Bioscience Biotechnology & Biochemistry, 58, 388–390.
- Zerrin, Y., Elif, A., & Yasar, K.E. (2010). Characterization of binding interactions between green tea flavanoids and milk proteins. Food Chemistry, 121, 450–456.