Abstract
Bovine β-lactoglobulin (βLG) was conjugated with fructooligosaccharides (FOS), galactooligosaccharides (GOS) and isomaltooligosacharides (IMO) by Maillard reaction in an effort to reduce the allergenicity of βLG. Fourier transform infrared spectra indicated that βLG was bound to FOS, GOS and IMO covalently. Structural analyses by dichroism spectra and fluorescence studies suggested that the surface of βLG in each conjugate was covered with oligosaccharides without apparent disruption of native conformation. The βLG-oligosaccharides conjugates almost maintained the retinol-binding activity of βLG. Enzyme-linked immunosorbent assay indicated that, conjugation of βLG with these functional oligosaccharides was effective in reducing the allergenicity of βLG.
1. Introduction
Allergy to cow's milk proteins is a common food allergy with an incidence of 2–6% in young infants (Hosking, Heine, & Hill, Citation2000; Mills et al., Citation2004). β-lactoglobulin (βLG) is the most important allergen in cow's milk, and 82% of milk allergy patients are sensitive to it (Spies, Citation1973). βLG accounts for 50% of total protein in the whey fraction (Sawyer, Citation2003). βLG is categorised as a member of the lipocalin superfamily (Flower, Citation1996). Although the physiological function of βLG still remains unclear, βLG can bind and transport small hydrophobic ligands such as retinol and fatty acids. βLG is used as a food additive for its multiple functions in food processing such as emulsifying, foaming and gelling properties (Foegeding, Kuhn, & Hardin, Citation1992; Kinsella, Citation1988; Shimizu, Saito, & Yamauchi, Citation1985). The monomer of βLG is an 18-kDa globular protein of 162 amino acids with four disulphide bonds and one free cysteine group. βLG is stable against hydrolysis and heat due to its intramolecular bonds maintain its structural integrity (Hambling, McAlpine, & Sawyer, Citation1992). Reduction of the allergenicity of βLG has been attempted by enzymatic digestion and denaturation (del Val et al., Citation1999; Ishizaka, Okudaira, & King, Citation1975; Kurisaki, Nakamura, Kaminogawa, & Yamauchi, Citation1982; Watanabe, Suzuki, Ikezawa, & Arai, Citation1994). However, these methods might be detrimental to its useful functions relevant to food processing. Therefore, it is essential to develop new methods that would reduce the allergenicity without destroying the functional properties of βLG.
Carbohydrates react with proteins may reduce the antigenicity of the protein (Rickard, David, Anne, & Mendel, Citation1990). Studies to reduce the antigenicity and the immunogenicity of βLG by conjugation to carbohydrates have been reported (Hattori, Citation2002; Hattori et al., Citation2000b). These conjugates can achieve improved functions (thermal stability, solubility, emulsifying ability, and so on) as well as reduced allergenicity of the proteins. Conjugation of a protein with polysaccharides would be preferable than other low molecular weight molecules, because a polysaccharide could cover the epitopes of the allergens more effectively than a low molecular weight molecule (Sehon, Citation1982). As the area of protein epitopes are constrained to six to eight amino acids (Amit, Mariuzza, Phillips, & Poljak, Citation1986), effective shielding can be expected with covalent binding of oligosaccharides (Hattori et al., Citation2004).
Functional oligosaccharides have been used extensively both as pharmacological supplements and food ingredients in processed food (Xu, Chao, & Wan, Citation2009). Among the carbohydrates that are qualified as prebiotics, fructooligosaccharides (FOS), galactooligosaccharides (GOS) and isomaltooligosacharides (IMO) are of interest. Some infant milk products contain these functional oligosaccharides to improve their nutrimental values. We postulate that conjugation with functional oligosaccharides could additionally reduce the allergenicity of a protein.
The main objective of this study was to determine the effects of FOS, GOS and IMO conjugation on the immunoreactivity of βLG. Herein, we conjugated βLG with FOS, GOS and IMO, respectively. After exploring the structures of these conjugates, we analysed IgE-binding and IgG-binding capacity of βLG before and after treatment of functional oligosaccharides by Enzyme-linked immunosorbent assay (ELISA).
2. Materials and methods
2.1. Materials
βLG, FOS, GOS and IMO were from Sigma Chemical Co., USA. All other reagents and solvents were of analytical reagent grade.
2.2. Production of anti-βLG polyclonal antibodies
Native βLG was used as the soluble antigen to prepare anti-βLG polyclonal antibodies. Briefly, the antigen protein was dissolved in Freund's complete adjuvant and intraperitoneally injected into BALB/c mice. The immunisation was boosted by administration of the same amount of the antigen protein in Freund's incomplete adjuvant, twice at two-week intervals. The resulting serum was collected and its IgG-binding ability toward βLG was assayed by western blotting. The antiserum was stored at −20°C until use.
2.3. Human sera
Individual serum samples from 15 children with milk allergies were collected from Shenzhen Children's Hospital. All patients demonstrated specific IgE antibodies toward milk proteins by Uni-CAP System (Pharmacia Diagnostics, Sweden). Western blotting verified that all sera demonstrated IgE-binding ability toward βLG. Pooled normal sera from patients were used as negative controls. All the patients gave their informed consent, as authenticated by the ethical board of Shenzhen University and stored at −20°C until use.
2.4. Preparation of βLG-oligosaccharides conjugates
The βLG-oligosaccharides conjugates were prepared by Maillard reaction according to the method of Hattori et al. (Hattori et al., Citation2004). In brief, 300 mg βLG and 300 mg oligosaccharide (FOS, GOS or IMO individually) were dissolved in 300 mL distilled water and lyophilised. The mixture was incubated at 50°C in a relative humidity of 79% for 48 h. After dialysis against distilled water and lyophilising, a crude βLG-oligosaccharides conjugate was obtained. Free oligosaccharides were removed by salting. Each crude sample was dissolved in distilled water, and ammonium sulphate was added to 100% saturation. The precipitate was recovered by centrifuging (20,000 rpm for 30 min) at 20°C. The purified conjugate was obtained after dialysis against distilled water and lyophilising.
Sugar concentration of sample solution was determined using HPLC. After filtration, 20 µL sample was fractionated using a NH2-derivatised column (4 mm ID×250 mm, 5 m particle size; Lichrosorb NH2, Merck, Germany) using acetonitrile/water (80/20) as mobile phase eluting at a rate of 1.0 mL/min. Sugar peaks were detected by a RI detector (2414 RI-monitor detector, Waters, USA) and calculated using a software (Empower 2, Waters, USA). Standard curves against the three oligosaccharides (FOS, GOS and IMO) were profiled by calculation of peak areas of standard oligosaccharides at concentrations of 15 mg/mL, 10 mg/mL, 7.5 mg/mL, 5 mg/mL and 2.5 mg/mL, respectively. The concentrations of the samples were calculated according to the plotted standard curves.
2.5. Fourier transform infrared (FTIR) spectroscopy
FTIR measurements were carried out on a FTIR-8300PCS FTIR spectrometer (Shimadzu Co., Japan) at 25°C. The spectrometer is fitted with a KBr beam splitter. All spectra were taken via the attenuated total reflection method with a resolution of 5 cm−1 and using 32 scans. The spectra-processing procedure involved collecting spectra of the KBr under the same conditions. The absorbency of the KBr was subtracted from the spectra of the samples to obtain the FTIR spectra. The spectral data from the scans of samples in the FTIR spectra were baseline corrected and normalised according to the protein peak from 1600 cm−1 to 1700 cm−1.
2.6. Circular dichroism (CD) measurements
The samples were recorded with a Jasco-810 spectrophotometer (Jasco Co., Japan) in cells of 1.0 mm path length at 25°C. Each sample was dissolved in phosphate-buffered saline (PBS) at a protein concentration of 0.015 mg/mL. The spectra were recorded in the range of 190–250 nm (the scan rate was 30 nm/min and the response time was 1 s). Three scans were accumulated for each spectrum.
2.7. Fluorescence spectroscopy
The sample dissolved in PBS at a 1.0×10−6 M (as the protein concentration) were recorded by Hitachi-850 spectrofluorometer (Hitachi Co., Japan) in a 1-cm quartz cell using an excitation wavelength of 280 nm. The excitation and emission bandwidths were 5 nm.
2.8. Measurement of the retinol-binding activity of the βLG-oligosaccharides conjugates
The retinol-binding activity of βLG and βLG-oligosaccharides conjugates was investigated by fluorescence titration with the Hitachi-850 spectrofluorometer (Hitachi Co., Japan) according to Hattori et al. (Hattori, Ogino, Nakai, & Takahashi, 1997).
2.9. Indirect ELISA experiments
The IgG-binding of native βLG and βLG-oligosaccharides conjugates was evaluated by competitive ELISA. Aliquots of βLG solution (100µL; 0.001% βLG in PBS) were added to a polystyrene microtitration plate and were incubated at 4°C overnight. The βLG solution was then removed. The wells were washed with phosphate-buffered saline tween-20 (PBST), 3.0% BSA in PBST was used as the blocking buffer. The blocking buffer was added to the plate and incubated at 37°C for 2 h. βLG or the prepared βLG-oligosaccharides conjugates at various concentrations (50 µL) were added to the wells. Polyclonal antibodies against βLG (50 µL) were immediately added, and the plate was further incubated at 37°C for 1 h. After washing with PBST, plates were then incubated with 100 µL per well of horseradish peroxidase (HRP) labelled goat anti-mouse IgG (Kirkegaard & Perry Laboratories, Inc., USA) diluted 1:10,000 in PBS solution. The plate was incubated for 45 min at 37°C. Optical densities (OD) were read at 450 nm on an automated ELISA plate reader (Multiskan MK3, Thermo, Finland).
The IgE-binding assay was conducted similarly to that of IgG with slight modifications. Briefly, wells were coated with 100 µL of antigen as described earlier. After the blocking step, βLG or βLG-oligosaccharides at various concentrations (50 µL) were added to the wells. The plate was then incubated with 50 µL per well of human sera (serum at 1:50 diluted in PBST solution) for 1 h at 37°C. About 100 µL per well of HRP-conjugated antihuman IgE (Kirkegaard & Perry Laboratories, Inc., USA) were added at a 1:1000 dilution in PBST solution. Finally, colour development was performed as described earlier.
The ELISA determinations were carried out in triplicate and measurements averaged.
3. Results and discussion
3.1. Structural features of the βLG-oligosaccharides conjugates
The composition of the conjugate determination (data not shown) indicated that βLG–FOS conjugate, βLG–GOS conjugate and βLG–IMO conjugate were composed of 1 molecule of βLG versus 9 molecules of FOS, 1 molecule of βLG versus 7 molecules of GOS and 1 molecule of βLG versus 7 molecules of IMO, respectively.
When a protein covalently binds to a saccharide molecule, a typical event is that the hydrogen bonds increase in the protein molecule. It is reflected as a wide peak in the range of 3700–3200 cm−1 in FRIT spectrum. The FRIT spectra of the βLG–oligosaccharides conjugates are shown in . Every βLG–oligosaccharides conjugate demonstrated stronger absorbency in the range of 3700–3200 cm−1. It indicated that βLG was bound to FOS, GOS and IMO covalently.
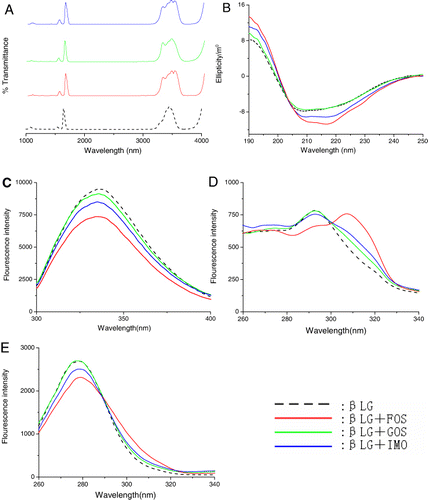
The CD spectra of the βLG–oligosaccharides conjugates are shown in . Two typical negative bands appeared in the ultraviolet region at 208 nm and 220 nm, which is characteristic of α-helical structures of proteins (Venyaminov & Vesilenko, Citation1994). The spectrum of the βLG–IMO conjugate was similar to the spectrum of the native protein. However, the βLG–GOS conjugate and βLG–FOS conjugate showed greater absolute θ values than those of native βLG from 208 nm to 218 nm in CD spectrum, suggesting the α-helical structures in the native βLG was increased to some extent after treatment. The secondary structure was determined using CONTIN method in DICHROWEB (Whitmore & Wallace, Citation2004). By this method, it was found that the α-helical content of the protein changed from 19% to 20%, 23% and 26% upon binding with GOS, IMO and FOS, respectively. This suggested that βLG experienced a slight conformational change upon conjugation with functional oligosaccharides.
The fluorescence spectra of the βLG–oligosaccharides conjugates are shown in . The fluorescence emission maximum wavelength was 335.6 nm for native βLG. Only a slight blue shift (<1 nm) was observed in all conjugates, implying that the conformation around the fluorophore residues of the βLG–oligosaccharides conjugates were maintained in the native form. The fluorescence intensity of βLG–oligosaccharides conjugates was lower than that of native βLG. FOS had the lowest intensity, followed by IMO and GOS. The results suggested that the oligosaccharides shielded the area around the fluorophore residues (Hattori et al., Citation1997, Citation2000a; Hattori, Nagasawa, Ametani, Kaminogawa, & Takahashi, Citation1994; Lakowicz & Weber, Citation1973).
To further investigate the binding of FOS, GOS and IMO to the βLG, synchronous fluorescence was utilised. The synchronous fluorescence spectra of the βLG-oligosaccharides systems are presented in the and . As shown in and , the fluorescence intensity of βLG was similar to βLG–GOS conjugate, whereas decreased a little along with the addition of βLG–FOS conjugate and βLG–IMO conjugate. As shown in , the fluorescence emission maximum wavelength of native βLG was 292 nm, the βLG–GOS conjugate and βLG–IMO conjugate exhibited a slight red shift (<1 nm) of the λmax, βLG–FOS conjugate had an apparent red shift (292–307 nm) of the λmax. The result implied that the functional oligosaccharides bound to βLG and located in close proximity to the Tyr residues (20Tyr, 42Tyr, 99Tyr and 102Tyr) and had an effect on the microenvironment around Tyr residues (Lakowicz, Citation1999). As shown in , βLG–GOS conjugate and βLG–IMO conjugate had a slight (<1 nm) red shift of the λmax, whereas βLG-FOS had a slight (<1 nm) blue shift of the λmax, indicating that functional oligosaccharides were close to the Trp residues (19Trp and 61Trp) and had an effect on the microenvironment around Trp residues.
According to multi-spectroscopic analysis, the results indicated that FOS, GOS and IMO bound to the surface of βLG via covalent bonds. The conformations are considered to have slight changes as a result of conjugation with functional oligosaccharides. FOS had the greatest influence on the structure of βLG, followed by IMO and GOS.
β-lactoglobulin can bind two molecules of retinol (Pèrez & Calvo, Citation1995). Changes in the retinol-binding activity of βLG after conjugation with FOS, GOS and IMO were investigated by fluorescence titration (). The retinol-binding activity of βLG was reduced a little by conjugation with all oligosaccharides. Of the three oligosaccharides, FOS reduced the retinol-binding activity the most, followed by IMO and GOS. This was in line with observations in spectroscopic experiments. Conjugation of βLG with oligosaccharides caused conformational changes that affect retinol-binding activity. Whereas the retinol-binding activity of βLG was reduced to some extent upon conjugation, the conjugates kept most of their retinol-binding activity.
3.2. ELISA experiments
The IgE-binding and IgG-binding capacities of βLG and βLG–oligosaccharides conjugates were measured by ELISA. As show in , the reactivity of native βLG with IgG antibodies and IgE antibodies was decreased after conjugation with all three oligosaccharides. ELISA results showed that the βLG–GOS conjugate had the lowest reactivity, while the βLG–FOS conjugate had the highest of the three.
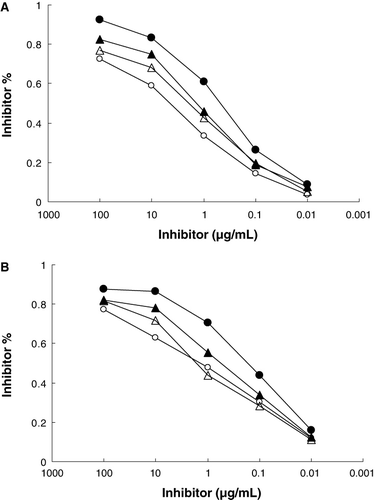
The molecular basis of changes in allergenic activity is the inactivation or destruction of epitope structures or increased inaccessibility to epitopes (Besler, Steinhart, & Paschke, Citation2001). According to structural analyses of the βLG–oligosaccharides conjugates, the conjugates did not significantly disrupt the native conformation of βLG. However, the changes in the conformation of the conjugates were not consistent with those of IgE-binding and IgG-binding reactivities of the conjugates. Therefore, the reduction of the binding affinity of βLG–oligosaccharides conjugates may be due mainly to shielding of the βLG epitopes by the attachment of oligosaccharides.
As for the IgG response to βLG, 51Glu-64Asp, 67Arg-88Asn, 129Asp-144Pro, 139Arg-156Thr are reported to be major epitopes (Jarvinen et al., Citation2010). And 41Val-60Lys, 102Tyr-124Val, 149Arg-162Ile are major epitopes for the IgE (Selo et al., Citation1999). All these epitopes are on the protein surface. The results of structural analyses according to the spectroscopic experiments indicated that the surface of βLG was covered by oligosaccharides. FOS, GOS and IMO in βLG–oligosaccharides conjugates may be attached directly to the epitope(s) or region(s) in the proximity, which thereby prevent anti-βLG antibodies from accessing the antigens.
It is essential to develop a new method that can reduce the allergenicity and maintain the functional properties of βLG. Although a little conformational change in βLG was observed after conjugation with oligosaccharides, the conjugates maintained the retinol-binding activity of protein. Our work found that the functional oligosaccharides effectively shielded epitopes of βLG, which reduced IgE-binding and IgG-binding capacity of βLG in vitro. However, the mechanism behind the reduced immunogenicity of βLG through conjugation with oligosaccharides needs more studies. We hope that this method will contribute to prepare novel proteins with reduced allergenicity and maintain functional properties.
Acknowledgements
This study was supported in part by research funding from the High-tech Industrialization Funding of Guangdong Province (No.2009B011300010), and the National Natural Science Foundation of PRC (No.31101280).
References
- Amit, A.G., Mariuzza, R.A., Phillips, S.E., & Poljak, R.J. (1986). Three-dimensional structure of an antigen antibody complex at 2.8 Å resolution. Science, 233, 747–753.
- Besler, M., Steinhart, H., & Paschke, A. (2001). Stability of food allergens and allergenicity of processed foods. Journal of Allergy and Clinical Immunology B, 756, 228–238.
- del Val, G., Yee, B.C., Lozano, R.M., Buchanan, B.B., Ermel, R.W., Lee, Y.M., et al. (1999). Thioredoxin treatment increases digestibility and lowers allergenicity of milk. Journal of Agricultural and Food Chemistry, 103, 690–669.
- Flower, D.R. (1996). The lipocalin protein family: Structure and function. Biochemical Journal, 318, 1–14.
- Foegeding, E.A., Kuhn, P.R., & Hardin, C.C. (1992). Specific divalent cation-induced changes during gelation of β-lactoglobulin. Journal of Agricultural and Food Chemistry, 40, 2092–2097.
- Hambling, S.G., McAlpine, A.S., & Sawyer, L. (1992). β-Lactoglobulin. In P.F. Fox (Ed.), Advanced dairy chemistry (pp. 141–190). London: Elsevier Applied Science.
- Hattori, M. (2002). Functional improvements in food proteins in multiple aspects by conjugation with saccharides: Case studies of â-lactoglobulin-acidic polysaccharides conjugates. Food Science and Technology Research, 8, 291–299.
- Hattori, M., Miyakawa, S., Ohama, Y., Kawamura, H., Takashi, Y., Kenji, T., et al. (2004). Reduced Immunogenicity of β-Lactoglobulin by Conjugation with Acidic Oligosaccharides. Journal of Agricultural and Food Chemistry, 52, 4546–4553.
- Hattori, M., Nagasawa, K., Ametani, A., Kaminogawa, S., & Takahashi, K. (1994). Functional changes in β-Lactoglobulin by conjugation with carboxymethyl dextran. Journal of Agricultural and Food Chemistry, 42, 2120–2125.
- Hattori, M., Nagasawa, K., Ohgata, K., Sone, N., Fukuda, A., Matsuda, H., et al. (2000a). Reduced immunogenicity of β-Lactoglobulin by conjugation with carboxymethyl dextran. Bioconjugate Chemistry, 11, 84–93.
- Hattori, M., Numamoto, K., Kobayashi, K., & Takahashi, K. (2000b). Functional changes in β-Lactoglobulin by conjugation with cationic saccharides. Journal of Agricultural and Food Chemistry, 48, 2050–2056.
- Hattori, M., Ogino, A., Nakai, H., & Takahashi, K. (1997). Functional improvement of β-Lactoglobulin by conjugating with alginate lyase-lysate. Journal of Agricultural and Food Chemistry, 45, 703–708.
- Hosking, C.S., Heine, R.G., & Hill, D.J. (2000). The Melbourne milk allergy study-two decades of clinical research. Allergy and Clinical Immunology International, 12, 198–205.
- Ishizaka, K., Okudaira, H., & King, T. (1975). Immunogenic properties of modified antigen E. II. Ability of urea-denatured antigen and a polypeptide chain to prime T cells specific for antigen. Journal of Immunology, 114, 110–115.
- Jarvinen, K.M., Chatchatee, P., Bardina, L., Beyer, K., & Sampson, H.A. (2010). IgE and IgG binding epitopes on alpha-lactalbumin and beta-lactoglobulin in cow's milk allergy. International Archives of Allergy and Immunology, 126, 111–118.
- Kinsella, J.E. (1988). Foaming and emulsifying properties of glycosylated â-lactoglobulin. Food Hydrocolloids, 2, 439–449.
- Kurisaki, J., Nakamura, S., Kaminogawa, S., & Yamauchi, K. (1982). The antigenic properties of β-lactoglobulin examined with mouse IgE antibody. Agricultural and biological chemistry, 46, 2069–2075.
- Lakowicz, J.R. (1999). Principles of fluorescence spectroscopy (2nd ed). New York: Kluwer Academic/Plenum.
- Lakowicz, J.R., & Weber, G. (1973). Quenching of protein fluorescence by oxygen. Detection of structural fluctuations in proteins on the nanosecond time scale. Biochemistry, 12, 4171–4179.
- Mills, E.N.C., Valovirta, E., Madsen, C., Taylor, S.L., Vieths, S., Anklam, E., et al. (2004). Information provision for allergy consumers-where are we going with food allergen labeling. Allergy, 59, 1262–1268.
- Pèrez, M.D., & Calvo, M. (1995). Interaction of β-lactoglobulin with retinol and fatty acids and its role as a possible biological function for this protein. Journal of Dairy Science, 78, 978–988.
- Rickard, E.O., David, L.B., Anne, H.B., & Mendel, F. (1990). Effect of Maillard browning reactions of the Kunitz soybean trypsin inhibitor on its interaction with monoclonal antibodies. Journal of Agriculture and Food Chemistry, 38, 258–261.
- Sawyer, L. (2003). β-lactoglobulin. In P. Fox & P. McSweeney (Eds.), Advanced dairy chemistry (pp. 319–386). New York: Kluwer Academic/Plenum.
- Sehon, A.H. (1982). Suppression of IgE antibody responses with tolerogenic conjugates of allergens and haptens. Progress in Allergy, 32, 161–202.
- Selo, I., Clement, G., Bernard, H., Chatel, J., Creminon, C., Peltre, G., et al. (1999). Allergy to bovine beta-lactoglobulin: Specificity of Human IgE to tryptic peptides. Clinical and Experimental Allergy, 29, 1055–1063.
- Shimizu, M., Saito, M., & Yamauchi, K. (1985). Emulsifying and structural properties of βlactoglobulin at different pHs. Agricultural and biological chemistry, 49, 189–194.
- Spies, J. (1973). Milk allergy. Milk Food Technology, 36, 225–231.
- Venyaminov, S.Y., & Vesilenko, K.S. (1994). Determination of protein tertiary structure class from circular dichroism spectra. Analytical Biochemistry, 222, 176–184.
- Watanabe, M., Suzuki, T., Ikezawa, Z., & Arai, S. (1994). Controlled enzymatic treatment of wheat proteins for production of hypoallergenic flour. Bioscience Biotechnology and Biochemistry, 58, 388–390.
- Whitmore, L., & Wallace, B.A. (2004). DICHROWEB: An online server for protein secondary structure analyses from circular dichroism spectroscopic data. Nucleic Acids Research, 32, 668–673.
- Xu, Q., Chao, Y.L., & Wan, Q.B. (2009). Health benefit application of functional oligosaccharides. Carbohydrate Polymers, 77, 435–441.
Supplementary Information
Methods
Sugar concentration determination of βLG–oligosaccharides conjugates
Sugar concentration of sample solution was determined using HPLC. After filtration, 20 µl sample was fractionated in a NH2 column (4 mm ID×250 mm, 5 m particle size; Lichrosorb NH2, Merck, Germany) using acetonitrile/water (80/20) as mobile phase eluting at a rate of 1.0 ml/min. Sugar peaks were detected by a RI detector (2414 RI-monitor detector, Waters, USA) and calculated using a software (Empower 2, Waters, USA). Standard curves against the three oligosaccharides (FOS, GOS and IMO) were profiled by calculation of peak areas of standard oligosaccharides at concentrations of 15 mg/mL, 10 mg/mL, 7.5 mg/mL, 5 mg/mL and 2.5 mg/mL, respectively. The concentrations of the samples were calculated according to the plotted standard curves.
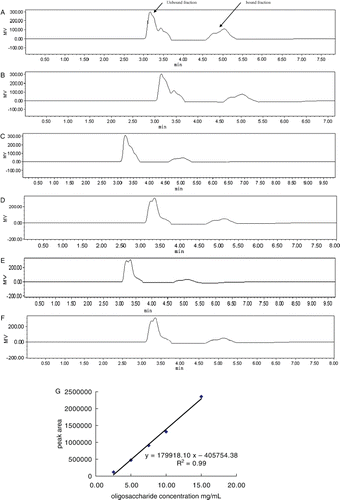