Abstract
Aims of methods
Effects of intervention with Lactobacillus casei Shirota (LcS) on the incidence of antibiotic-associated diarrhoea (AAD), Clostridium difficile infection (CDI) and changes in faecal microbiota were analysed using C. difficile ELISA (678 patients), qPCR using 16S rRNA group-specific primers, C. difficile-toxin kit and polymerase chain reaction/denaturing gradient gel electrophoresis (56 patients).
Results
Results
As much as 18.5% of antibiotic treated group developed AAD, but only 5% of patients treated with antibiotics and LcS. Following antibiotic therapy, a decrease in the abundance of total Bacteria, Clostridium cluster IV and XI, Bifidobacterium spp. and butyryl-CoA CoA transferase genes was observed, whereas Enterobacteriaceae increased. LcS intervention reduced the antibiotic-associated decrease in the diversity of microbiota, increased the abundance of Lactobacillus spp. and reduced the antibiotic-induced decrease of Bifidobacterium spp.
Conclusions
Antibiotic treatment affects the diversity and the composition of the microbiota impairing butyrate production. Intervention with certain Lactobacillus strains may antagonise some of these changes, and more potent short-chain fatty acid-stimulating probiotics are desirable for intervention in AAD.
1. Introduction
The human gut contains approximately 10–100 trillion organisms (Zhu, Wang, & Li, Citation2010), including all three domains of life: Bacteria, Archaea and Eukarya (Backhed, Ley, Sonnenburg, Peterson, & Gordon, Citation2005); recently three functional subtypes (‘enterotypes’) have been described (Arumugam et al., Citation2011). The gastrointestinal microbiota is dominated by two divisions of Bacteria: Bacteroidetes and Firmicutes (Backhed et al., Citation2005; Wang, Heazlewood, Krause, & Florin, Citation2003). Ninety-five per cent of the Firmicutes belong to the Clostridium, while large variations can be observed among the Bacteroidetes phylotypes (Eckburg et al., Citation2005).
A high microbial diversity supports a stable gut ecosystem, providing the host with protection against pathogens (Backhed et al., Citation2005). This stability can be influenced by many factors including intestinal pH, microbial interactions, environmental temperature, physiologic factors, peristalsis, bile acids, host secretions, immune responses, drug therapy and bacterial mucosal receptors (Thompson-Chagoyan, Maldonado, & Gil, Citation2007). If this ecosystem is disturbed and there is a reduction in the abundance or group specific shifts, pathogens are more able to colonise and cause harm.
Antibiotic treatment is one factor that allows pathogens to proliferate, and antibiotics can result in both short- and long-term effects on the human gut microbiota. Bacterial diversity may decrease, the relative proportions of different species can change, new species can be introduced and/or existing species completely eradicated (Dethlefsen & Relman Citation2011; Sommer & Dantas, Citation2011). Proteobacteria, for example, which are normally low in abundance, are increased at the expense of the normally dominant Firmicutes and Bacteroidetes. There is also continuing concern about the increasing rise in antibiotic resistance (Sommer & Dantas, Citation2011), all of which makes it important that antibiotic-associated changes to the intestinal microbiota are characterised and to find ways to resist these changes and achieve a rapid return to the original composition (De La Cochetiere et al., Citation2005).
Antibiotic-associated diarrhoea (AAD) is a common complication of antibiotic use. Nearly all types of antibiotics are reported to induce AAD, but those with a spectrum of activity including anaerobic bacteria have been particularly associated with higher rates of AAD (Breves et al., Citation2000). Clinically mild AAD is mostly caused by the toxic effects of the antibiotics themselves on the intestinal mucosa, or their pharmacological effects on gut motility, functional disturbances of intestinal bile acid or carbohydrate metabolism (Hogenauer, Hammer, Krejs, & Reisinger, Citation1998), accompanied by a decrease in the microbial metabolism of complex carbohydrates to short-chain fatty acids (SCFAs). SCFAs are essential for sodium and water uptake (Breves et al., Citation2000), as well as having a role as important energy sources for intestinal epithelial cells (Hamer et al., Citation2008), and anti-inflammatory, epigenetic and genetic activities (Canani et al., Citation2011). More severe AAD-causing complications include electrolyte imbalances, dehydration, pseudomembranous colitis, toxic megacolon or death. These complications can be caused by infection with Clostridium difficile (a member of Clostridium cluster XI), or other infectious agents including C. perfringens, Staphylococcus aureus, Klebsiella oxytoca, Candida species and Salmonella species (Breves et al., Citation2000; Hogenauer et al., Citation1998).
Intestinal overgrowth with C. difficile is a particularly dangerous complication of antibiotic intake. The pathogen causes severe diarrhoea and pseudomembranous colitis, entities associated with outbreaks having a high rate of mortality for hospitalised patients (Thompson-Chagoyan et al., Citation2007). This anaerobic spore-forming bacterium is believed to be responsible for 5–20% of all cases of AAD (Hogenauer et al., Citation1998), for virtually all cases of pseudomembranous colitis (Bartlett, Citation1987), and a frequent cause of outbreaks (Arumugam et al., Citation2011; Hensgens et al., Citation2011; Mcfarland, Beneda, Clarridge, & Raugi, Citation2007). Clostridium difficile releases at least two potent toxins that cause mucosal damage and inflammation of the colon (Hogenauer et al., Citation1998).
The almost complete inhibition of butyrate fermentation may be an important pathophysiological factor for the development of AAD, because butyrate has an essential role in ensuring the morphological and functional integrity of colonocytes (Breves et al., Citation2000). The effect of decreasing SCFA production could be compensated by probiotic intervention. This has been shown with Saccharomyces boulardii although the yeast restored acetate and propionate fermentation but not butyrate (Breves et al., Citation2000).
Saccharomyces boulardii has the strongest evidence with regard to reducing incidence of AAD and/or C. difficile-associated diarrhoea (McFarland, Citation2010) but evidence is also available for probiotic bacteria including Lactobacillus rhamnosus GG (McFarland, Citation2006), L. acidophilus CL1285 and L. casei LBC80R (Gao, Mubasher, Fang, Reifer, & Miller, Citation2010), L. rhamnosus GG, L. acidophilus La-5 and Bifidobacterium Bb-12 (Wenus et al., Citation2008), L. casei DN-114 001 with the yogurt cultures L. delbrueckii subsp. bulgaricus and Streptococcus thermophilus (Hickson et al., Citation2007) and L. casei Shirota (Lewis, Thomas, & Weir, Citation2009; Martinez, Paz, & O'Donnell, Citation2003). The safety of Lactobacillus casei Shirtoa (LcS) has been confirmed in several studies (Snydman, Citation2008; Whelan & Myers, Citation2010).
The rationale for combining a probiotic with antibiotic therapy is that the antibiotic kills any vegetative C. difficile cells in the intestine and eliminates their toxigenic effect, the probiotic helps to re-establish the protective intestinal microbiota so that when any residual spores germinate, their outgrowth and colonisation is prevented by the newly restored microbiota barrier (McFarland, Citation2006).
In the present study, the effects of using a probiotic with antibiotic therapy on the incidence of AAD and C. difficile infection (CDI) were investigated in a group of 678 hospitalised patients receiving antibiotics. The probiotic was a fermented milk drink containing LcS administered during and for three days after a course of antibiotics. Changes in faecal microbiota were analysed in a subgroup of 56 subjects. The results suggest an important role of LcS in antagonising antibiotic-induced changes in the intestinal microbiota with regard to its abundance, diversity and SCFA-producing capacity.
2. Material and methods
2.1. Subjects
The study was performed at the department of Internal Medicine of the General Hospital Oberpullendorf (Austria). Ethical clearance was given by the local ethical committee (Ethikkommission Burgenland). For the clinical study, patients given antibiotics were divided into two cohorts. Group AP (antibiotic + probiotic) comprised 340 patients (182 females and 158 males with a mean age of 71 years) fulfilling the following criteria: (1) they received a daily probiotic drink containing the probiotic strain LcS (one 65 ml bottle containing a minimum of 6.5×109 live cells of LcS) whilst taking antibiotics and for 3 days after the antibiotic treatment ceased; (2) they were treated with appropriate antibiotics administered either by oral or parenteral route according to their clinical indication (penicillins, cephalosporins, quinolones, clindamycin and vancomycin); (3) they were on a ward where all patients and the staff received probiotic (regardless of antibiotic therapy). Evaluation of compliance showed that at least 80% of the patients and 95% of the staff ingested the probiotic drink on a daily basis. Group A (on antibiotics but not given probiotic) comprised 338 patients (172 females and 166 males with a mean age of 69 years). The following exclusion criteria were defined: (1) diarrhoea on admission or within the previous week of the admission; (2) intake of high-risk antibiotics within one month before admission to the hospital (cephalosporins, clindamycin, aminopenicillin and quinolones were predefined as high-risk antibiotics); (3) recurrent diarrhoea or chronic intestinal diseases associated with diarrhoea; (4) known re-current CDI. The patient groups did not differ significantly in terms of demographic characteristics or severity of disease. Of note, both groups received similar antibiotic regimens and there was no change in the infection prevention policy over the trial period. All subjects agreed to participate in the study and gave their informed consent.
Faecal samples were taken for further laboratory study from the sub-set of 56 subjects (aged 60±22 years), which were divided into four groups (). Patients of groups A and AP had been treated with antibiotics; patients of groups AP and P had received a daily fermented milk drink (Yakult®) containing the probiotic strain L. casei Shirota. The control group (C) consisted of healthy adults receiving neither antibiotics nor probiotics.
Table 1. Characterisation of participant groups.
2.2. Clinical endpoints
Primary endpoint: The occurrence of diarrhoea, defined as more than two abnormally loose bowel movements (i.e. unformed or liquid stools that were strictly defined as stool taking up the shape of the container and confirmed as such by a nurse) per day for the duration of three or more days.
Secondary endpoint: The incidence of CDI, defined as the onset of diarrhoea (as described above) plus detection of toxin A or toxin B in stool samples by immunoassay (TOX A/B QUICK CHEK®, Wampole®).
2.3. Sampling and DNA extraction from stool
Faecal samples were taken at three time points within the course of a week: before antibiotic treatment and/or intake of probiotic (day 0) and two times during antibiotic treatment and/or intake of probiotic (after 3 and 5 days). Samples of the control group were taken at similar time points. All samples were immediately stored at−70°C.
For DNA extraction, 200 mg of frozen stool sample was treated twice for 45 s in a bead-beater (Mini-Beadbeater-8) with one intervention minute on ice. The DNA was then extracted using QIAamp DNA Stool Mini Kit (QUIAGEN) following the manufacturers’ protocol and immediately stored at−20°C.
2.4. Quantification of total bacteria, bacterial subgroups and detection of butyryl-CoA CoA transferase genes by q-PCR
For TaqMan q-PCR of total bacteria, Clostridium cluster IV and XIVa, all Bacteroides and Bifidobacterium spp., a reaction mix with the total volume of 10 µL, 5 µL SensiMix™ Probe Kit (TaqMan®) (3 mM MgCl2), 1 µL of each primer, 1 µL of TaqMan®-probe () and 10 ng template were used. For SYBR Green Real Time PCR of Lactobacillus spp., Enterobacteriaceae (4 mM MgCl2) and Clostridium cluster XI, a reaction mix with the total volume of 10 µL, 5µL SensiMix™ SYBR No-ROX Kit (SYBR® Green) (3 mM MgCl2), 1 µL of each primer () and 10 ng template were used. Assays were carried out in a StepOnePlus™ Instrument (96 wells) using Real-Time PCR System Version 2.1 (Applied Biosystems).
Table 2. Primers and TaqMan®-probes targeting 16rRNA coding regions of bacteria.
Table 3. Primers targeting 16rRNA coding regions of bacteria (SYBR® Green) and butyryl-CoA CoA transferase genes.
For comparison of PCR reaction efficiencies among different experiments, one faecal sample and tenfold series of DNA dilutions of following type strains were used: Clostridium leptum DSM 753, Clostridium blautia, Bifidobacterium longum DSM 20211, L. casei, Escherichia coli 1029 and a bacterial mix containing C. leptum DSM 735, C. blautia, C. difficile 301968DNA, S. aureus, Enterococcus faecium DSM 20477, Listeria monocytogenes, L. casei, Bacteroides thetaiotaomicron DSM 2079, E. coli 1029, Citrobacter caseri, Enterobacter cloacae 1252, Salmonella typhimurium SL7207, B. longum subsp. suis DSM 20211.
Standard curves were created using serial dilutions of known concentrations of organisms containing the respective amplicons for each set of primers, and quantification was done. All templates were determined in duplicate, and the average used for calculation.
Euroclone®C. difficile A/B kit was used for specific C. difficile toxin determination, following the user manual.
Butyryl-CoA CoA transferase genes were detected as described previously (Hippe et al., Citation2011; Louis & Flint, Citation2007).
2.5. PCR/DGGE
Polymerase chain reaction (PCR) was used to amplify 16S rRNA gene sequences from total bacteria in faecal samples and type strains for further use in denaturing gradient gel electrophoresis (DGGE) analysis.
Assays were carried out in a 96-well Gradient Thermal Cycler (Labnet MultiGene™), using a ready-to-use GoTaq® Green Master Mix (Promega) with 1.5 mM MgCl2 and the specific primer sets 341f-GC 5′-CCT ACG GGA GGC AGC AG-3′ (Muyzer, de Waal, & Uitterlinden, Citation1993) and 518r 5′-ATT ACC GCG GCT GCT GG-3′ (Neefs, Van De Peer, De Rijk, Goris, & De Wachter, Citation1991). The DGGE gel was prepared as described previously (Muyzer & Smalla, Citation1998) with a linear gradient of 25–65%, using a gradient mixer (Hoefer SG 30) and a peristaltic pump. A reference marker was generated, containing fragments of 16S rRNA genes from cultured bacteria and clones generated from faecal material: Bac. thetaiotaomicron, Ent. faecium, C. leptum 16, E. coli, C. coccoides 43, L. reuteri and B. longum.
2.6. Statistical analysis
Fisher's exact test was performed to compare the rates of AAD and CDI. Faecal analysis data were statistically analysed using the program OriginPro 8 (OriginLab®).
To compare two unpaired groups of interval values the parametric two-sample t-test, and of interval values the non-parametric Mann–Whitney U-test was used. For three unpaired group comparison of interval values the parametric one-way ANOVA and of ordinal values the non-parametric Kruskal–Wallis ANOVA were used. P-values less than 0.05 were defined as statistically significant.
3. Results
3.1. AAD and C. difficile infection
In the control group, 63 of 338 patients developed AAD, equivalent to an incidence of 18.6%. Of the patients suffering from AAD, an immunoassay proved that 21 had CDI, demonstrating a CDI incidence of 6.2% in this control group. On the other hand, 17 of 340 patients in the intervention group developed AAD, equivalent to an AAD incidence of 5%. One of the patients suffering from AAD had a positive immunoassay, confirming CDI. As a result, the incidence of CDI in the intervention group was 0.3%. Compared to the control group, the probiotic intervention group demonstrated a relative risk reduction of 73.2% for development of AAD (p<0.001) and 95.3% for CDI (p<0.001) (based on the immunoassay).
Among the faecal samples for microbiota analysis, one patient in group A tested positive using the qPCR Euroclone®C. difficile A/B kit but no one in group AP (). This test detected all three C. difficile strains provided from the Division for Human Medicine of the Austrian Agency for Health and Food Safety (AGES). Two patients in group A and one patient in group AP developed AAD.
Table 4. Group distribution concerning antibiotic-associated diarrhoea (AAD) and Clostridium difficile infection (CDI).
3.2. Total bacteria
In the faecal analysis of the subset of subjects, both antibiotic-treated groups had a significant lower number of copies per gram than the control groups (p=8.4 * 10−4), particularly at time points two (AP: p=0.02; A: p=0.03) and three (AP: p=0.003; A: p=0.02) compared to group C. Within the antibiotic groups, a mean decrease of copies per gram was observed (A).
(A: antibiotic treatment; AP: antibiotic treatment and intake of a probiotic drink containing Lactobacillus casei Shirota; P: intake of a probiotic drink containing L. casei Shirota; C: control group; 1: day 0, 2: day 3, 3: day 5).
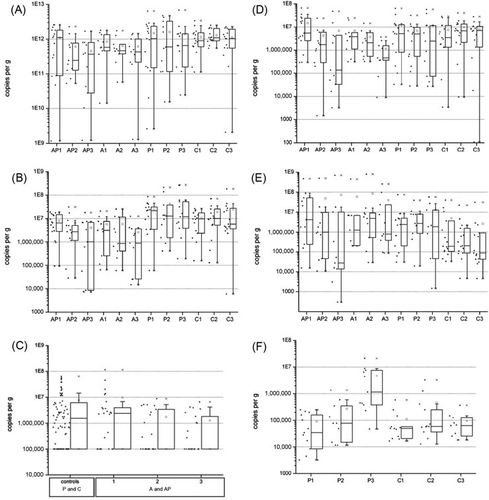
Denaturing gradient gel electrophoresis band pattern analysis () of 16S rRNA coding regions of total bacteria amplified with primer pair 341GC-518 revealed that on average two more bands could be detected in group AP, increasing from time point 1 to 3, in comparison to group A (A1:A3 10:8 bands, AP1:AP3 8:9 bands), indicating a higher diversity was maintained in the AP group.
(A: antibiotic treatment; AP: antibiotic treatment and intake of a probiotic drink containing Lactobacillus casei Shirota; P: intake of a probiotic drink containing L. casei Shirota; C: control group; 1: day 0, 3: day 5, SL: standard line).
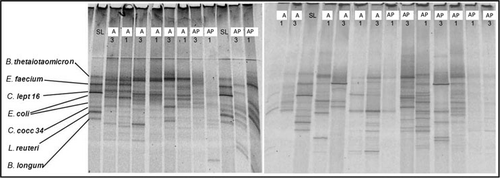
3.2.1. Relative amount of bacteria
Most striking is that in patients receiving antibiotics, the Clostridium Clusters IV and XIV are clearly reduced compared to the healthy controls and at the first time point the Enterobacteriaceae are clearly increased (). Furthermore, in patients under antibiotic treatment, there were more bacteria which cannot be identified by the primers used in this study ().
(A: antibiotic treatment; AP: antibiotic treatment and intake of a probiotic drink containing Lactobacillus casei Shirota; P: intake of a probiotic drink containing L. casei Shirota; C: control group; 1: day 0, 2: day 3, 3: day 5).
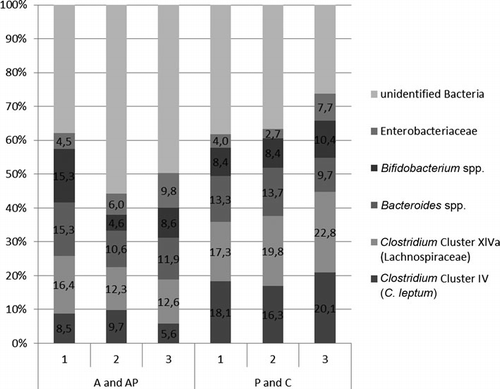
3.3. Bacterial subgroups
Clostridium cluster IV (C. leptum subgroup): The values of the groups receiving antibiotics were on average lower by a half-power than the two control groups not on antibiotics. This difference was statistically significant (p=6.31 * 10−7). Group AP and A and group P and C did not differ from each other (B).
Clostridium cluster XI: After commencement of the antibiotic treatment, the abundance of Clostridium cluster XI decreased, whereas in the control groups values remained constant (C).
All Bacteroides: Evaluation of the results revealed no remarkable differences.
Bifidobacterium spp.: The mean number of copies per gram of both antibiotic groups was lower than those of the control groups (p=0.051). The average numbers of copies fell in group AP and A, but in group AP this decrease stopped, after the LcS intake was begun and levels started to rise again slightly (D).
Enterobacteriaceae: In the antibiotic groups AP and A, the number of copies per gram was higher by a power than those of both groups without antibiotic treatment (P and C) (p=0.06). Furthermore, it was apparent that individual values were widely spread. There was a statistically significant difference between all four groups (p=6.9 * 10−5) but not within the three time points of each group (E).
Lactobacillus spp.: In group P, the values of each time point differed significantly (p=0.01) and the number of copies per gram increased significantly from time point 1 to 3 (p=0.02) as well as from time point 2 to 3 (p=0.045). At time point 3, the number of copies per gram was significantly higher in group P than in group C (p=0.04) (F).
3.4. Butyryl-CoA CoA transferase gene
In patients receiving antibiotics (group AP and A), the amount of butyryl-CoA CoA transferase genes was lower than that in the controls (p=1.08 * 10−5). The graph also showed a difference in values between the two groups on antibiotic treatment, group AP and A; however, this was not statistically significant ().
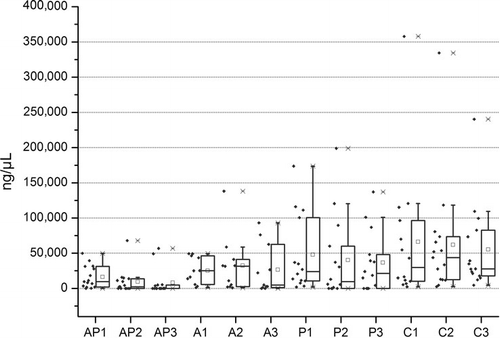
4. Discussion
Results of a clinical trial including 678 patients suggest that AAD is significantly decreased by giving the probiotic L. casei Shirota in combination with antibiotic therapy. We used 16S rRNA based qPCR and PCR/DGGE to analyse possible differences in faecal microbiota of subjects receiving antibiotics and/or a combined antibiotic/L. casei Shirota intervention.
Antibiotics clearly changed the composition of the gastrointestinal microbiota as indicated by a change in the abundance of subgroups and in their diversity. As described in recent studies (De La Cochetiere et al., Citation2005; Dethlefsen & Relman Citation2011; Jakobsson et al., Citation2010; Sommer & Dantas, Citation2011), our results showed that antibiotic intake caused group-specific shifts, and in general a reduction of bacterial abundance.
The quantitative analysis by qPCR revealed that the total amount of bacteria decreased as a result of antibiotic treatment, either with or without probiotic intake. However, further qualitative analysis using DGGE indicated that diversity remains higher when the probiotic was consumed during and after antibiotic therapy. This result certainly merits further investigation with a bigger sample size.
It was also observed that the abundance of the bacterial subgroups Bifidobacterium spp., Clostridium clusters IV and XI was reduced during antibiotics, whereas the abundance of Enterobacteriaceae increased. A dramatic decline in the Actinobacteria phylum, which includes Bifidobacteria, following antibiotic intake has been described previously (Jakobsson et al., Citation2010). Bifidobacteria are inulin and starch degraders; lactate is the primary fermentation product and is converted to butyrate by secondary fermenters (Dethlefsen, Eckburg, Bik, & Relman, Citation2006) thus a decline in Bifidobacteria represents a decrease in an important SCFA-producing group.
Short-chain fatty acids result from anaerobic microbial fermentation of indigestible carbohydrate or dietary fibre (Fredstrom, Lampe, Jung, & Slavin, Citation1994). The main SCFAs are acetate, propionate and butyrate, which stimulate the colonic blood flow and fluid and electrolyte uptake (Topping & Clifton, Citation2001). Furthermore, indirect actions of butyrate are believed to involve the hormone-neuro-immuno system (Guilloteau et al., Citation2010). As described previously, SCFAs are also essential for sodium and water uptake. Reduced production of SCFAs is linked to pathogenic mechanisms causing diarrhoea. Therefore, the observation that combined use of L. casei Shirota antagonises the drop of Bifidobacteria caused by antibiotics could be linked to the clinical results showing reduced AAD incidence with the probiotic. This is in line with the previous reports of increasing abundance of Bifidobacteria during LcS intake (Bian et al., Citation2011; Nagata et al., Citation2011).
The decline of Clostridium cluster IV would also have a strong impact on SCFA-production. This subgroup represents 22% of the total faecal bacteria, with Faecalibacterium prausnitzii strains being the most abundant (Lay et al., Citation2005). Together with Clostridium cluster XIVa, cluster IV contains the main butyrate-producing bacteria in human gut, and in particular strains related to F. prausnitzii (Pryde, Duncan, Hold, Stewart, & Flint, Citation2002). These results are in line with the observed lower values of butyryl-CoA CoA transferase gene in antibiotic-treated patients. Butyrate has also been implicated in the down-regulation of bacterial virulence (Guilloteau et al., Citation2010); a decrease in butyrate allows pathogen growth in the intestinal ecosystem. In the present study, the butyryl-CoA CoA transferase gene was used as a marker for butyrate production because direct analysis of SCFAs in faeces is hampered by the rapid binding and degradation of SCFAs in the gut. Clearly, results from the analysis of the butyryl-CoA CoA transferase gene focus on the prediction of butyrate production and not on the actual production which is difficult to assess.
The abundance of Clostridium cluster XI was decreased in patients who were prescribed antibiotics and declined during their treatment. Clostridium cluster XI is a heterogeneous phylogenetic cluster including opportunistic pathogens, such as C. difficile (Nadal et al., Citation2009). Clostridium difficile is a Gram-positive, spore-forming, anaerobe that can be carried asymptomatically in the human intestinal tract. Antibiotics suppressing the intestinal microbiota can allow C. difficile to proliferate and subsequently cause intestinal damage, inflammation and clinical disease (Lawley et al., Citation2009). Results of the present clinical study suggest that consumption of L. casei Shirota efficiently prevents the development of CDI and reduces development of AAD. In this study, CDI was evaluated by TOX A/B QUICK CHEK®, Wampole® immune assay for all the patients on antibiotics in the study. For the molecular analysis of the subset of 56 samples, Euroclone®C. difficile A/B kit was used. Problems and different outcomes in the detection of C. difficile using different test systems have been widely discussed (Alcala et al., Citation2008; Eastwood, Else, Charlett, & Wilcox, Citation2009; Luna et al., Citation2011).
Administration of probiotics upon administration of antibiotics has been discussed for developing antibiotic resistance and the use of probiotics is critically discussed in critically ill patients (Singhi & Baranwal, Citation2008). Although L. casei is vancomycin resistant, L. casei Shirota does not have vanA or vanB genes which confer transferable vancomycin resistance (Kiwaki and Kushiro, Citation2010)
5. Conclusions
This study also confirmed that antibiotic treatment, as well as the underlying disease requiring this therapy, was mainly associated with changes in the diversity and composition of the gastrointestinal microbiota, accompanied by a lower occurrence of butyryl-CoA CoA transferase genes. Pathogens are more able to colonise a disturbed intestinal ecosystem; reduction in both bacterial diversity and production of SCFAs might significantly contribute to problems following antibiotic treatment. It is apparent that intervention with L. casei Shirota antagonises some of these changes. Successful probiotic intervention in AAD might therefore be directed at the problem of reduced production of SCFAs that occurs after antibiotic treatment.
Acknowledgements
We thank the staff of the General Hospital Oberpullendorf. The project was funded by the Austrian Science Fund FWF.
References
- Alcala, L., Sanchez-Cambronero, L., Catalan, M.P., Sanchez-Somolinos, M., Peláez, M.T., Marín, M., et al. (2008). Comparison of three commercial methods for rapid detection of Clostridium difficile toxins A and B from fecal specimens. Journal of Clinical Microbiology, 46(11), 3833–3835.
- Arumugam, M., Raes, J., Pelletier, E., Le Paslier, D., Yamada, T., Mende, D.R., et al. (2011). Enterotypes of the human gut microbiome. Nature, 473(7346), 174–180.
- Backhed, F., Ley, R.E., Sonnenburg, J.L., Peterson, D.A., & Gordon, J.I. (2005). Host-bacterial mutualism in the human intestine. Science, 307(5717), 1915–1920.
- Bartlett, C.L. (1987). Efficacy of different surveillance systems in detecting hospital-acquired infections. Chemioterapia, 6(3), 152–155.
- Bian, L., Nagata, S., Asahara, T., Rahman, M.S., Ohta, T., Yuki, N., et al. (2011). Effects of the continuous intake of Lactobacillus casei strain Shirota-fermented milk on risk management of long-term inpatients at health service facilities for the elderly. International Journal of Probiotics and Prebiotics, 6(2), 123–132.
- Breves, G., Faul, K., Schroder, B., Holst, H., Caspary, W.F., & Stein, J. (2000). Application of the colon-simulation technique for studying the effects of Saccharomyces boulardii on basic parameters of porcine cecal microbial metabolism disturbed by clindamycin. Digestion, 61(3), 193–200.
- Canani, R.B., Costanzo, M.D., Leone, L., Pedata, M., Meli, R., & Calignano, A. (2011). Potential beneficial effects of butyrate in intestinal and extraintestinal diseases. World Journal of Gastroenterology, 17(12), 1519–1528.
- De La Cochetiere, M.F., Durand, T., Lepage, P., Bourreille, A., Galmiche, J.P., & Dore, J. (2005). Resilience of the dominant human fecal microbiota upon short-course antibiotic challenge. Journal of Clinical Microbiology, 43(11), 5588–5592.
- Dethlefsen, L., Eckburg, P.B., Bik, E.M., & Relman, D.A. (2006). Assembly of the human intestinal microbiota. Trends in Ecology and Evolution, 21(9), 517–523.
- Dethlefsen, L., & Relman, D.A. (2011). Incomplete recovery and individualized responses of the human distal gut microbiota to repeated antibiotic perturbation. Proceedings of the National Academy of Sciences of the United States of America, 108(Suppl. 1), 4554–4561.
- Eastwood, K., Else, P., Charlett, A., & Wilcox, M. (2009). Comparison of nine commercially available Clostridium difficile toxin detection assays, a real-time PCR assay for C. difficile tcdB, and a glutamate dehydrogenase detection assay to cytotoxin testing and cytotoxigenic culture methods. Journal of Clinical Microbiology, 47(10), 3211–3217.
- Eckburg, P.B., Bik, E.M., Bernstein, C.N., Purdom, E., Dethlefsen, L., Sargent, M., et al. (2005). Diversity of the human intestinal microbial flora. Science, 308(5728), 1635–1638.
- Fredstrom, S.B., Lampe, J.W., Jung, H.J., & Slavin, J.L. (1994). Apparent fiber digestibility and fecal short-chain fatty acid concentrations with ingestion of two types of dietary fiber. JPEN Journal of Parenteral and Enteral Nutrition, 18(1), 14–19.
- Gao, X.W., Mubasher, M., Fang, C.Y., Reifer, C., & Miller, L.E. (2010). Dose-response efficacy of a proprietary probiotic formula of Lactobacillus acidophilus CL1285 and Lactobacillus casei LBC80R for antibiotic-associated diarrhea and Clostridium difficile-associated diarrhea prophylaxis in adult patients. American Journal of Gastroenterology, 105(7), 1636–1641.
- Guilloteau, P., Martin, L., Eeckhaut, V., Ducatelle, R., Zabielski, R., & Van Immerseel, F. (2010). From the gut to the peripheral tissues: The multiple effects of butyrate. Nutrition Research Reviews, 23(2), 366–384.
- Hamer, H.M., Jonkers, D., Venema, K., Vanhoutvin, S., Troost, F.J., & Brummer, R.J. (2008). Review article: the role of butyrate on colonic function. Alimentary Pharmacology and Therapeutics, 27(2), 104–119.
- Hensgens, M.P., Goorhuis, A., Van Kinschot, C.M., Crobach, M.J., Harmanus, C., & Kuijper, E.J. (2011). Clostridium difficile infection in an endemic setting in the Netherlands. European Journal of Clinical Microbiology Infectious Diseases, 30(4), 587–593.
- Hickson, M., D'souza, A.L., Muthu, N., Rogers, T.R., Want, S., Rajkumar, C., et al. (2007). Use of probiotic Lactobacillus preparation to prevent diarrhoea associated with antibiotics: randomised double blind placebo controlled trial. BMJ, 335(7610), 80.
- Hippe, B., Zwielehner, J., Liszt, K., Lassl, C., Unger, F., & Haslberger, A.G. (2011). Quantification of butyryl CoA:acetate CoA-transferase genes reveals different butyrate production capacity in individuals according to diet and age. FEMS Microbiology Letters, 316(2), 130–135.
- Hogenauer, C., Hammer, H.F., Krejs, G.J., & Reisinger, E.C. (1998). Mechanisms and management of antibiotic-associated diarrhea. Clinical Infectious Diseases, 27(4), 702–710.
- Jakobsson, H.E., Jernberg, C., Andersson, A.F., Sjolund-Karlsson, M., Jansson, J.K., & Engstrand, L. (2010). Short-term antibiotic treatment has differing long-term impacts on the human throat and gut microbiome. PLoS One, 5(3), e9836.
- Kiwaki, M., & Kushiro, A. (2010). Antibiotic susceptibility testing of Lactobacillus casei by the broth microdilution method and a survey of vancomycin-resistance genes in the probiotic L. casei strain Shirota. Annual Report of Yakult Central Institute for Microbiological Research, 29, 27–36.
- Lawley, T.D., Clare, S., Walker, A.W., Goulding, D., Stabler, R.A., Croucher, N., et al. (2009). Antibiotic treatment of Clostridium difficile carrier mice triggers a supershedder state, spore-mediated transmission, and severe disease in immunocompromised hosts. Infection and Immunity, 77(9), 3661–3669.
- Lay, C., Sutren, M., Rochet, V., Saunier, K., Dore, J., & Rigottier-Gois, L. (2005). Design and validation of 16S rRNA probes to enumerate members of the Clostridium leptum subgroup in human faecal microbiota. Environmental Microbiology, 7(7), 933–946.
- Layton, A., Mckay, L., Williams, D., Garrett, V., Gentry, R., & Sayler, G. (2006). Development of Bacteroides 16S rRNA gene TaqMan-based real-time PCR assays for estimation of total, human, and bovine fecal pollution in water. Applied and Environmental Microbiology, 72(6), 4214–4224.
- Lewis, J.D.N., Thomas, L.V., & Weir, W. (2009). The potential of probiotic fermented milk products in reducing risk of antibiotic-associated diarrhoea and Clostridium difficile disease. International Journal of Dairy Technology, 62(4), 461–471.
- Louis, P., & Flint, H.J. (2007). Development of a semiquantitative degenerate real-time PCR-based assay for estimation of numbers of butyryl-coenzyme A (CoA) CoA transferase genes in complex bacterial samples. Applied and Environmental Microbiology, 73(6), 2009–2012.
- Luna, R.A., Boyanton, B.L., Jr., Mehta, S., Courtney, E.M., Webb, C.R., Revell, P.A., et al. (2011). Rapid stool-based diagnosis of Clostridium difficile infection by real-time PCR in a children's hospital. Journal of Clinical Microbiology, 49(3), 851–857.
- Martinez, C.O., Paz, V.S., & O'Donnell, A. (2003). Lactobacillus casei Shirota prevention approach for healthy children with mild acute infection treated with antibiotics. Pediatric Research, 4(Suppl. 2), 174A.
- Matsuki, T., Watanabe, K., Fujimoto, J., Takada, T., & Tanaka, R. (2004). Use of 16S rRNA gene-targeted group-specific primers for real-time PCR analysis of predominant bacteria in human feces. Applied and Environmental Microbiology, 70(12), 7220–7228.
- McFarland, L.V. (2006). Meta-analysis of probiotics for the prevention of antibiotic associated diarrhea and the treatment of Clostridium difficile disease. American Journal of Gastroenterology, 101(4), 812–822.
- McFarland, L.V. (2010). Systematic review and meta-analysis of Saccharomyces boulardii in adult patients. World Journal of Gastroenterology, 16(18), 2202–2222.
- Mcfarland, L.V., Beneda, H.W., Clarridge, J.E., & Raugi, G.J. (2007). Implications of the changing face of Clostridium difficile disease for health care practitioners. American Journal of Infection Control, 35(4), 237–253.
- Meier, H., Amann, R., Ludwig, W., & Schleifer, K.H. (1999). Specific oligonucleotide probes for in situ detection of a major group of gram-positive bacteria with low DNA G + C content. Systematic and Applied Microbiology, 22(2), 186–196.
- Muyzer, G., de Waal, E.C., & Uitterlinden, A.G. (1993). Profiling of complex microbial populations by denaturing gradient gel electrophoresis analysis of polymerase chain reaction-amplified genes coding for 16S rRNA. Applied and Environmental Microbiology, 59(3), 695–700.
- Muyzer, G., & Smalla, K. (1998). Application of denaturing gradient gel electrophoresis (DGGE) and temperature gradient gel electrophoresis (TGGE) in microbial ecology. Antonie Van Leeuwenhoek, 73(1), 127–141.
- Nadal, I., Santacruz, A., Marcos, A., Warnberg, J., Garagorri, M., Moreno, L.A., et al. (2009). Shifts in clostridia, bacteroides and immunoglobulin-coating fecal bacteria associated with weight loss in obese adolescents. International Journal of Obesity (London), 33(7), 758–767.
- Nagata, S., Asahara, T., Ohta, T., Yamada, T., Kondo, S., Bian, L., et al. (2011). Effect of the continuous intake of probiotic-fermented milk containing Lactobacillus casei strain Shirota on fever in a mass outbreak of norovirus gastroenteritis and the faecal microflora in a health service facility for the aged. British Journal of Nutrition, 106(4), 549–556.
- Neefs, J.M., Van De Peer, Y., De Rijk, P., Goris, A., & De Wachter, R. (1991). Compilation of small ribosomal subunit RNA sequences. Nucleic Acids Research, 19(Suppl), 1987–2015.
- Otsubo, M., Shimizu, T., Tanaka, R., Sawabe, T., Tajima, K., Yoshimizu, M., et al. (2002). Oligonucleotide probe for detecting Enterobacteriaceae by in situ hybridization. Journal of Applied Microbiology, 93(1), 60–68.
- Penders, J., Vink, C., Driessen, C., London, N., Thijs, C., & Stobberingh, E.E. (2005). Quantification of Bifidobacterium spp., Escherichia coli and Clostridium difficile in faecal samples of breast-fed and formula-fed infants by real-time PCR. FEMS Microbiology Letters, 243(1), 141–147.
- Pryde, S.E., Duncan, S.H., Hold, G.L., Stewart, C.S., & Flint, H.J. (2002). The microbiology of butyrate formation in the human colon. FEMS Microbiology Letters, 217(2), 133–139.
- Singhi, S.C., & Baranwal, A. (2008). Probiotic use in the critically ill. Indian Journal of Pediatrics, 75(6), 621–627.
- Snydman, D.R. (2008). The safety of probiotics. Clinical Infectious Diseases, 46(Suppl. 2), S104–S111; discussion S144–S151.
- Sommer, M.O., & Dantas, G. (2011). Antibiotics and the resistant microbiome. Current Opinion in Microbiology, 14, 556563.
- Song, Y., Liu, C., & Finegold, S.M. (2004). Real-time PCR quantitation of clostridia in feces of autistic children. Applied and Environmental Microbiology, 70(11), 6459–6465.
- Thompson-Chagoyan, O.C., Maldonado, J., & Gil, A. (2007). Colonization and impact of disease and other factors on intestinal microbiota. Digestive Diseases and Sciences, 52(9), 2069–2077.
- Topping, D.L., & Clifton, P.M. (2001). Short-chain fatty acids and human colonic function: Roles of resistant starch and nonstarch polysaccharides. Physiological Reviews, 81(3), 1031–1064.
- Walter, J., Hertel, C., Tannock, G.W., Lis, C.M., Munro, K., & Hammes, W.P. (2001). Detection of Lactobacillus, Pediococcus, Leuconostoc, and Weissella species in human feces by using group-specific PCR primers and denaturing gradient gel electrophoresis. Applied and Environmental Microbiology, 67(6), 2578–2585.
- Wang, X., Heazlewood, S.P., Krause, D.O., & Florin, T.H. (2003). Molecular characterization of the microbial species that colonize human ileal and colonic mucosa by using 16S rDNA sequence analysis. Journal of Applied Microbiology, 95(3), 508–520.
- Wenus, C., Goll, R., Loken, E.B., Biong, A.S., Halvorsen, D.S., & Florholmen, J. (2008). Prevention of antibiotic-associated diarrhoea by a fermented probiotic milk drink. European Journal of Clinical Nutrition, 62(2), 299–301.
- Whelan, K., & Myers, C.E. (2010). Safety of probiotics in patients receiving nutritional support: A systematic review of case reports, randomized controlled trials, and nonrandomized trials. American Journal of Clinical Nutrition, 91(3), 687–703.
- Woo, P.C., Leung, P.K., Leung, K.W., & Yuen, K.Y. (2000). Identification by 16S ribosomal RNA gene sequencing of an Enterobacteriaceae species from a bone marrow transplant recipient. Molecular Pathology, 53(4), 211–215.
- Yu, Y., Lee, C., Kim, J., & Hwang, S. (2005). Group-specific primer and probe sets to detect methanogenic communities using quantitative real-time polymerase chain reaction. Biotechnology and Bioengineering, 89(6), 670–679.
- Zhu, B., Wang, X., & Li, L. (2010). Human gut microbiome: the second genome of human body. Protein Cell, 1(8), 718–725.
- Zwielehner, J., Liszt, K., Handschur, M., Lassl, C., Lapin, A., & Haslberger, A.G. (2009). Combined PCR-DGGE fingerprinting and quantitative-PCR indicates shifts in fecal population sizes and diversity of Bacteroides, bifidobacteria and Clostridium cluster IV in institutionalized elderly. Experimental Gerontology, 44(6–7), 440–446.